1 Introduction
Alpha-glucosidase (EC 3.2.1.20) is an enzyme that catalyzes the hydrolysis of 1, 4-alpha-glucosidic linkages, releasing alpha-glucose. This enzyme strongly hydrolyzes sucrose, maltose, maltodextrin and pNP-α-D-glucopyranoside. It can be found in the alimentary canal, salivary secretions of insects and hypopharyngeal glands of some insects, such as Apis melifera L. [1,2]. Glucosidase are enzymes widely spread in living organisms. In insects, digestive β-glucosidases are important for the hydrolysis of di- and oligo-β-saccharides derived from hemicelluloses and cellulose and are involved in insect–plant interactions [3]. Although hydrolyzing similar substances, insect digestive β-glucosidases have different specificities, preferring β-glucosides or β-galactosides as substrates with hydrophobic or hydrophilic moieties in the aglycone part of the substrate [1].
Glucosidases have been found in several lepidopteran insects, such as Chilo suppressalis Walker (Lep.: Pyralidae) [4], Thaumetopoea pityocampa Schiffermuller (Lep.: Notodontidae) [5] and Parnassius apollo L. (Lep.: Papilionidae) [6]. Thus, more data on other lepidopteran species are essential to further our understanding on the properties of these enzymes.
The Lesser Mulberry Pyralid, Glyphodes pyloalis Walker (Lep.: Pyralidae), has been found in Guilan province, Iran, since 2002 and it was abundant in recent years. This pest is a specialist insect on mulberry (Morus spp.), and is widely distributed throughout Asia and the Northern provinces of Iran, where the species causes serious damage to sericulture not only by its larval grazing on leaves, but also by its possible transmission of some viral diseases infectious to the silkworm [7]. Since insecticide application should be at minimum in sericulture, it is worth developing alternative and bio-rational methods to control the species by a better understanding of insect digestive enzymes for developing transgenic plants to control phytophagous insects.
Digestion is a process of splitting unabsorbable macromolecules of food by digestive enzymes into utilizable and absorbable molecules by the addition of water. Thus the study of the digestive enzymes forms an important base for the study of digestive physiology. There are good reasons to investigate α- and β-glucosidases activity in this pest because these enzymes play an important role in insect digestion, as L-glycans is major dietary components of many insect species [1]. These enzymes are active upon a large range of substrates and in some cases they are implicated in plant resistance to insect predation, because of the occurrence in many plants of β-glucosides that release cyanide (or other toxic compounds) upon hydrolysis [1].
At present, there is no information available on the α- and β-glucosidases of G. pyloalis and a detailed knowledge of G. pyloalis digestive physiology will provide new opportunities for a sustainable pest management to control this insect pest. The goal of the present study is to characterize the glucosidases in the midgut and salivary extract of G. pyloalis. The present note reports some properties α- and β-glucosidases of salivary glands and midgut of G. pyloalis.
2 Materials and methods
2.1 Insect
The insect was collected from mulberry (Morus alba L.) leaves in Guilan provinces of Iran, Rasht, and was reared on the M. alba leaves. The same-aged larvae (24 h after molt for each instar) were randomly selected for measuring of enzyme activity.
2.2 Sample preparation and enzyme assays
Larvae were immobilized on ice and dissected under a stereo microscope in ice-cold saline buffer. The gut and salivary glands were removed and midgut content was eliminated (midgut without food). The samples were transferred to the freezer (−80 °C) and stored for 2 weeks and then homogenized in cold double-distilled water using a hand-held glass homogenizer and centrifuged at 13,000 × g (27,000 rpm) for 15 min at 4 °C. The supernatants were collected and stored at −20 °C for subsequent analyses. Concerning the whole body of the larvae, we used the same-aged larvae (24 h after molt for each instar) for analysis. At first, the samples was transferred to freezer (−80 °C) and stored for about 3 weeks and then homogenized in 40 mM citrate-phosphate-acetate sodium buffer (pH 7.5 for α-glucosidase activity and 5.5 for β-glucosidase activity).
2.3 Determination of α- and β-glucosidase activity and protein concentration
Total and specific activities of midgut digestive enzymes and salivary glands were determined in the homogenates. All assays were performed at 35 °C in 40 mM citrate-phosphate-acetate sodium buffer in five replicates. Activities of α- and β-glucosidases were measured with p-nitrophenyl-α-D-glucopyranoside (pNαG), p-nitrophenyl-β-D-glucopyranoside (pNβG) as substrates, respectively. Homogenate were incubated for 30 min at 35 °C with 45 μL of pNαG (25 mM) or pNβG (25 mM) and 115 μL of 40 mM citrate-phosphate-acetate sodium buffer. The reaction was stopped by addition of 600 μL of NaOH (0.25 M). Optical density was measured at 405 nm using microplate reader (Stat Fax 3200, Awareness Technology, USA) after 10 min. Controls without enzyme or without substrate were included. A standard curve of absorbance against amount of p-nitrophenol released was constructed to enable calculation of the amount of p-nitrophenol released during the α- and β-glucosidase assays. The protein concentration was determined by the Bradford method [8] using bovine serum albumin (Bio-Rad, München, Germany) as standard.
2.4 Determination of pH optimum and effect of temperature on enzyme activity
The activity of α- and β-glucosidases was determined at several pH values using 40 mM citrate-phosphate-acetate sodium buffer (pH 4.0 to 11.5). The effect of temperature on α- and β-glucosidase activities were measured using the homogenate of midgut of larvae by incubating the reaction mixture at 15, 22, 35, 45, 55 and 65 °C for 35 min, followed by measurement of activity.
2.5 Effect of activators and inhibitors on enzyme activity
To test the effect of different ions on the enzyme activities, midguts were dissected in distilled water. Enzyme assays were performed in the presence of different concentrations of chloride salts of Na+, Mg2+, Ca2+ and K+ (5, 10, 20 and 40 mmol L−1), and ethylenediaminetetraacetic acid (EDTA; 0.5, 1, 2 and 4 mmol L−1), sodium dodecylsulfate (SDS; 1, 2, 4 and 8 mmol L−1) and urea (0.5, 1, 2 and 4 mol L−1). These compounds were added to the assay mixture, and activity was measured after 35 min incubation.
2.6 Kinetic parameters of β-glucosidase in midgut
The Michaelis–Menten constant (Km) and the maximal reaction velocities (Vmax) of β-glucosidase were determined by Lineweaver–Burk plots. The homogenate was incubated in an appropriate buffer (pH 7.5) at 35 °C with pNβG in concentrations ranging from 5 to 160 mM (final concentration ranging from 0.25–6 mM). The experiments were performed in triplicate. The Michaelis–Menten constant (Km) and maximum velocity (Vmax) were evaluated by non-linear regression analysis using the software package Prism (GraphPad Software).
2.7 Statistical analysis
Data were compared by one-way analysis of variance (ANOVA) followed by Tukey's test when significant differences were found at p = 0.05 using SAS program [9].
3 Results
3.1 α- and β-glucosidase activity
Studies showed that α- and β-glucosidase activity is present in the midgut and salivary glands of larvae G. pyloalis and in the whole body of the larvae (Table 1 and Fig. 1). The activity of the β-glucosidase was always more in the midgut than salivary glands; the specific activity of midgut enzyme in 5th instar larvae was 1.07 ± 0.003 μmol−1 min−1 mg protein−1 and the specific activity of this enzyme in the salivary glands was 0.072 ± 0.002 μmol−1 min−1 mg protein−1 (Table 1). There was a significant difference in α- and β-glucosidase activity of whole body homogenate between 2nd, 3rd, 4th and 5th instars (df = 3, F = 347.3, p < 0.0001). The amounts of β-glucosidase activity did not change significantly in the whole body homogenate of 3rd and 4th larval stages (Fig. 1). Enzyme activities in these stages were 0.25 and 0.24 μmol−1 min−1 mg protein−1, respectively. When activity of α- and β-glucosidase in whole body homogenate of larval stage was determined, data showed that the highest activity of α- and β-glucosidase was observed in the 5th larval stage as 0.168 and 0.645 μmol−1 min−1 mg protein−1, respectively, and the lowest activity in the 2nd larval stage, 0.042 and 0.164 μmol−1 min−1 mg protein−1, respectively (Fig. 1).
Comparison of the activity of α- and β-glucosidase in different tissue of G. pyloalis.
Specific activity (μmol/min/mg protein); (mean ± SE) | Unit activity (nmol/min/tissue); (mean ± SE) | |||
Tissue | Alpha | Beta | Alpha | Beta |
Salivary glands of 3rd instar lavae | 0.0289 ± 0.001d | 0.068 ± 0.005d | 0.062 ± 0.001e | 0.24 ± .017e |
Midgut of 3rd instar lavae | 0.0937 ± 0.008c | 0.42 ± 0.01c | 3.14 ± 0.36c | 29.84 ± 0.77c |
Midgut of 5th instar larvae + food | 0.195 ± 0.004a | 1.07 ± 0.003a | 8.77 ± 0.19a | 104.68 ± 5a |
Midgut of 5th instar larvae without food | 0.13 ± .008b | 0.998 ± 0.01b | 5.87 ± 0.34b | 99.9 ± 2.5b |
Salivary glands of 5th instar lavae | 0.194 ± 0.003a | 0.072 ± 0.002d | 1.6 ± 0.067d | 4.35 ± .23d |

Activity level of α- and β-glucosidase in whole body of 2nd–5th instar larvae of the lesser mulberry pyralid. Different letters indicate that the activity of enzymes in different instar are significantly different from each other by Tukey's test (p < 0.05).
Result also showed that α- and β-glucosidase activity in salivary glands and midgut of 5th instar was higher than 3rd instar (Table 1).
3.2 Effect of pH and temperature on the α- and β-glucosidase activity
The effect of pH and temperature on the hydrolytic activity toward pNαG and pNβG were tested using 40 mM citrate-phosphate-acetate sodium buffer (pH 3–11.5). Maximum activity in midgut was observed at pH 7.5 and 5.5 for α- and β-glucosidase, respectively but the enzyme activity was not much affected in the range of pH 5.5–6.5 for β-glucosidase (Figs. 2 and 3); at pH 6.5, the reaction velocity was 96% of the maximum value achieved at pH 7.5. Most of α-glucosidase, purified from several sources, exhibits pH optima ranging from 4.5 to 7.0 [10]. As shown in Fig. 4, the optimum pH of the α- and β-glucosidase from salivary glands was around 8-9. The activity of the enzyme decreased rapidly below pH 8 according to the relative activity of 100% for the β-glucosidase at pH 8. The G. pyloalis α- and β -glucosidase has an optimum temperature activity at 45 °C (Fig. 5).
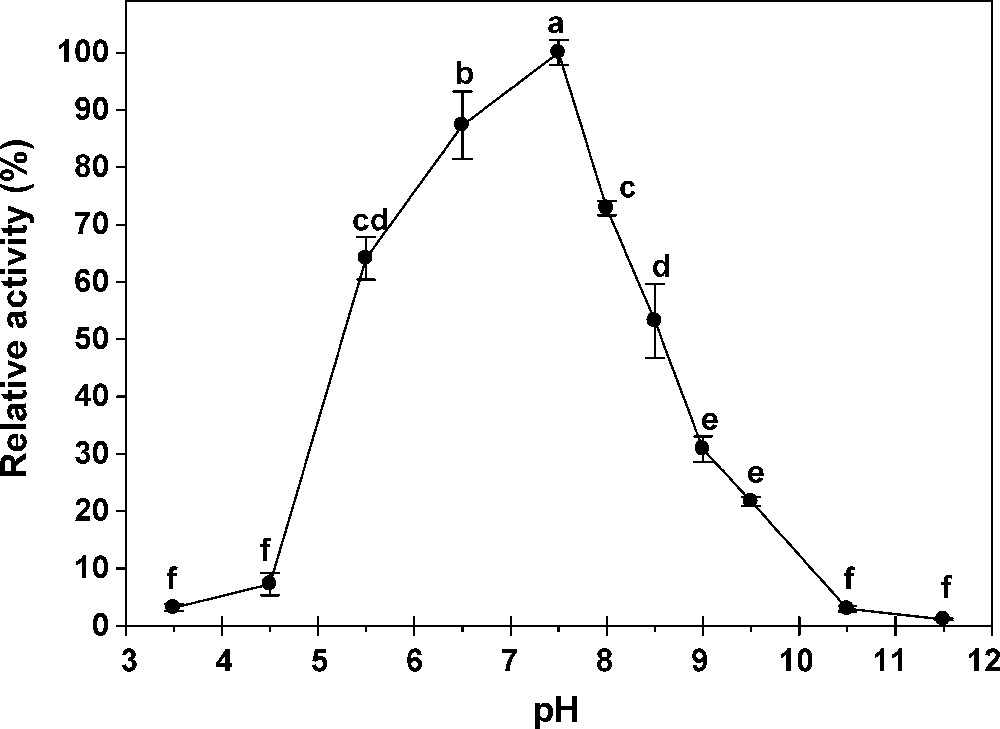
Effect of pH on activity of α-glucosidases extracted from midgut of G. pyloalis. Different letters indicate that the activity of enzymes in different pH are significantly different from each other by Tukey's test (p < 0.05).
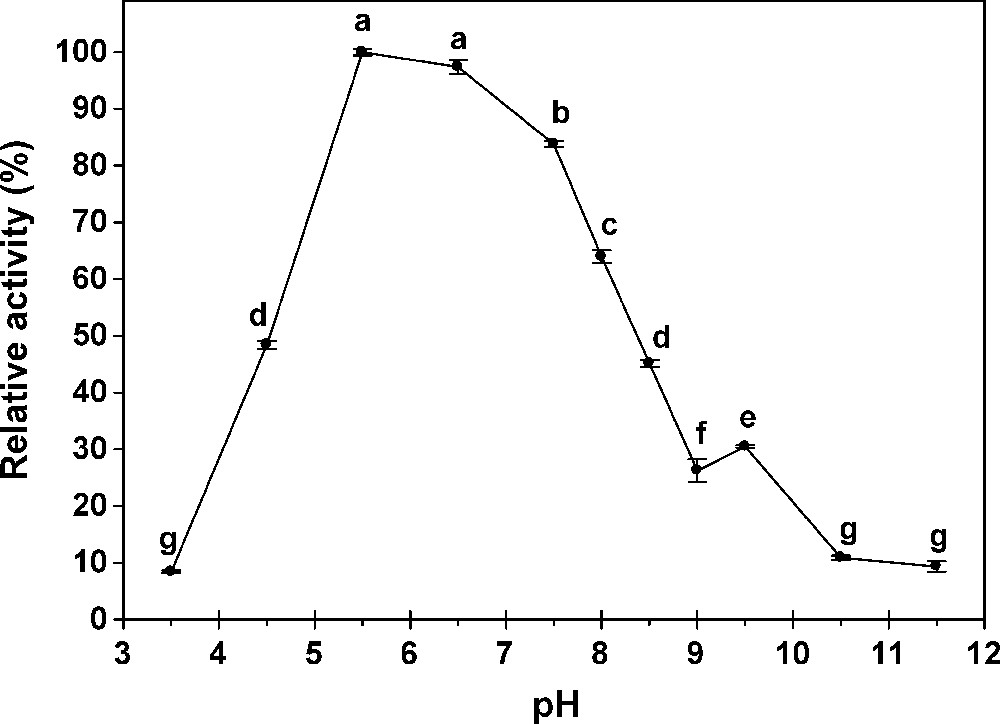
Effect of pH on activity of ß-glucosidases extracted from midgut of G. pyloalis. Different letters indicate that the activity of enzymes in different pH are significantly different from each other by Tukey's test (p < 0.05).
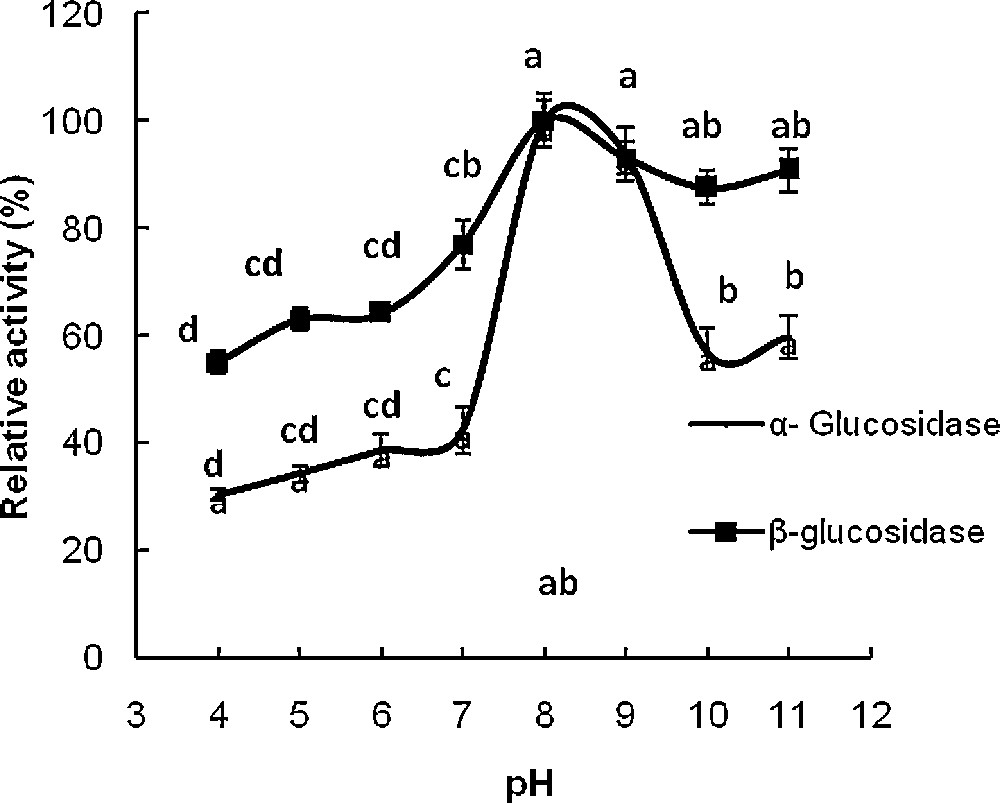
Effect of pH on activity of α- and β-glucosidases extracted from salivary glands G. pyloalis. Different letters indicate that the activity of enzymes in different pH are significantly different from each other by Tukey's test (p < 0.05).

Effect of temperature on activity of α- and β-glucosidases extracted from midgut of G. pyloalis. Different letters indicate that the activity of enzymes in different temperature are significantly different from each other by Tukey's test (p < 0.05).
3.3 Effects of metal ions and other reagents on the α- and β-glucosidase activity
A wide variety of metal ions and other chemical reagents were examined for their effects on the α and β-glucosidase activity. The CaCl2 (40 mM) decreased β-glucosidase activity and increased α-glucosidase activity. The α-glucosidase activity was enhanced with increasing in concentration of EDTA, whereas β-glucosidase activity was decreased with increasing in the concentration of the reagent. The β-glucosidase activity decreased due to the use of different concentrations of KCl (5, 10, 20 and 40), but these concentrations did not have any effect on α-glucosidase activity. Also, the α-glucosidase activity was not affected by using of MgCl2, but 10 mM of this ion increased significantly the β-glucosidase activity. Urea (4 mM) and SDS (8 mM) decreased significantly the β-glucosidase activity (Figs. 6–12).

Relative activity of midgut G. pyoalis α- and β- glucosidase toward different concentration of CaCl2. Different letters indicate that the activity of enzymes in various concentration are significantly different from each other by Tukey's test (p < 0.05).

Relative activity of midgut G. pyoalis α- and β- glucosidase toward different concentration of EDTA. Different letters indicate that the activity of enzymes in various concentration are significantly different from each other by Tukey's test (p < 0.05).

Relative activity of midgut G. pyoalis α- and β-glucosidase toward different concentration of KCl. Different letters indicate that the activity of enzymes in various concentration are significantly different from each other by Tukey's test (p < 0.05).

Relative activity of midgut G. pyoalis α- and β-glucosidase toward different concentration of MgCl2. Different letters indicate that the activity of enzymes in various concentration are significantly different from each other by Tukey's test (p < 0.05).

Relative activity of midgut G. pyoalis α- and β-glucosidase toward different concentration of NaCl. Different letters indicate that the activity of enzymes in various concentration are significantly different from each other by Tukey's test (p < 0.05).
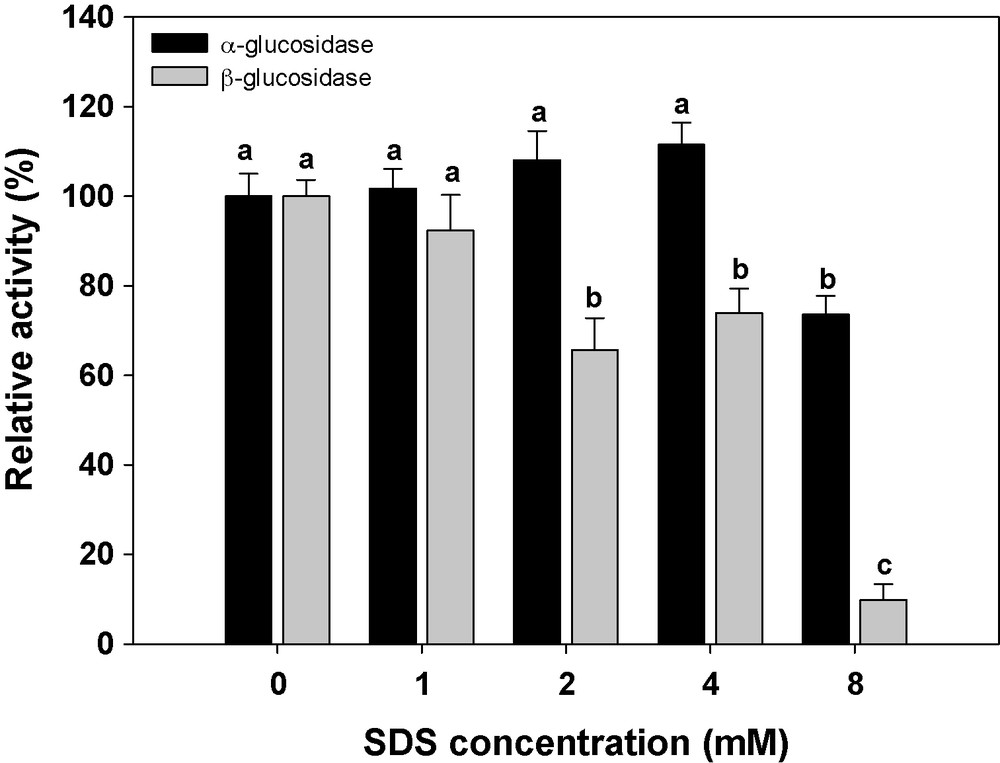
Relative activity of midgut G. pyoalis α- and β-glucosidase toward different concentration of SDS. Different letters indicate that the activity of enzymes in various concentration are significantly different from each other by Tukey's test (p < 0.05).

Relative activity of midgut G. pyoalis α- and β-glucosidase toward different concentration of urea. Different letters indicate that the activity of enzymes in various concentration are significantly different from each other by Tukey's test (p < 0.05).
3.4 Kinetic parameters of β-glucosidase in the midgut
Kinetic parameters of the midgut β -glucosidase against pNβG were estimated at pH 7.5 and 37 °C. The Km and Vmax values of the enzyme were estimated as 0.99 mM and 0.30 μmol−1 min−1 mg protein−1, respectively (Fig. 13).

Lineweaver–Burk plots of β-glucosidase in midgut of G. pyloalis larvae.
4 Discussion
Alpha- and β-glucosidase activity was detected in midgut (0.195 ± 0.004 and 1.07 ± 0.003 μmol−1 min−1 mg protein−1, respectively) and salivary glands (0.194 ± 0.003 and 0.072 ± 0.002 μmol−1 min−1 mg protein−1, respectively) of 5th larval instar using pNαG and pNβG as substrate (Table 1). The presence of α- and β-glucosidase activity in the salivary glands of other phytophagous lepidopterans has been reported [4,11–14]. Beta-glucosidases are important enzymes for insect metabolism and for insect–plant interactions. Besides hydrolyzing several carbohydrates, thus liberating monosaccharides that can be absorbed, they can cleave plant toxic glycosides. These, after being hydrolyzed by a β-glucosidase, can form cyanide or other toxic compounds such as inhibitors of glucose 6-phosphate dehydrogenase [15] or trehalase [16]. Some insects avoid intoxication by these substances lowering the β-glucosidase activity or by losing the relevant enzymes activity. This reason perhaps explains the low activity of these enzymes in midgut and salivary glands of G. pyloalis. Also, the activities of α- and β-glucosidase in the midgut and salivary glands of C. suppressalis were 0.009, 0.0063, 0.005 and 0.003 μmol−1 min−1mg protein−1, respectively [4]. Azevedo et al. [17] showed that the specific β-glucosidases activity in crude midgut homogenate of the sugar cane borer, Diatraea saccharalis Fabricius (Lepidoptera: Pyralidae) was 0.021 μmol−1 min−1 mg protein−1 using NPβGlu (p-nitrophenyl β-glucoside) as substrate. It seems the activity of these enzymes are low in lepidopterian insect such as G. Pyloalis, Spodoptera frugiperda Smith (Lepidoptera: Noctuidae), Erinnyis ello Linnaeus (Lepidoptera: Sphingidae), D. saccharalis Fabricius (Lepidoptera: Pyralidae) and C. suppressalis [4,18]. In contrast, Nakonieczny et al. [6] concluded that very high β-glucosidase activity (in comparison to other Lepidoptera) found in Apollo larvae suggests that its feeding strategy allows utilization of at least some compounds containing β-glycosidic bonds. High activities of detoxifying enzymes in P. apollo midgut tissues support this suggestion. Also, β-glucosidase activity in midgut and salivary glands of Neotermes koshunensis (Isoptera: Kalotermitidae) was 1.67 and 0.02 mmol−1 min−1 mg protein−1, respectively [19]. Therefore, the activity of this enzyme in midgut and salivary glands of N. koshunensis and P. apollo ssp. Frankenbergeri was much higher than that of G. pyloalis. Also, our results showed that the α- and β-glucosidase activity in midgut and salivary glands of G. pyloalis was much higher than C. suppressalis.
The 2nd larval stage of the lesser mulberry pyralid feeds little, which may be one reason why larvae of this stage show very low α- and β-glucosidase activity (0.042 and 0.164 μmol−1 min−1 mg protein−1) (Fig. 1). Feeding is usually intensified at the 5th instar and α- and β-glucosidase activity was highest in this stage.
Ferreira et al. [18] reported that high β-glucosidase activity is found in the foliage feeder, Abracris flavolineata De Geer (Orthoptera: Acrididae), in the stored plant product-feeder Tenebrio molitor Linnaeus (Coleoptera: Tenebrionidae), and in the pollen-feeder Scaptotrigona bipunctata Lepeletier (Hymenoptera: Apidae). In contrast, low β-glucosidase activity is found among predaceous insects exemplified by Pheropsophus aequinoctialis Linnaeus (Coleoptera: Carabidae) and Pyrearinus termitilluminans Costa (Coleoptera: Elateridae). Their results showed that high β-glucosidase activity could be associated with feeding on plant or plant products, other data disagree with this suggestion. Beta-glucosidase activity is low in the decaying plant-feeder Rhynchosciara americana Wiedemann (Diptera: Sciaridae) and in feeders of plant aerial parts, exemplified by S. frugiperda, E. ello and D. saccharalis [18]. In the literature review, β-galactosidase activity is relatively low in insect midguts. Comparing activities against p-nitrophenyl glycosides of glucose and galactose, ratios of β-glucosidase/β-galactosidase is 88.5 in R. americana [20], 105 in Stomoxys calcitrans [21], 58 in midgut tissue of Rhodnius prolixus [22], and 2.5 in Callosobruchus maculates [23]. In Dysdercus peruvianus, the β-glucosidase/β-galactosidase ratio in midgut tissue is 28.7, but in the whole midgut (epithelium plus luminal contents) the ratio is 3.0, suggesting a major contribution of β-galactosidase activity by the seed meal present in gut lumen. The ratio found in D. peruvianus is of the same order as that found in the seed-eating beetle C. maculates [24]. Our results showed that there is no significant difference in activity of α- and β–glucosidase at midgut of 5th instar containing food and without food (Table 1). The results showed that activity of the β-glucosidase in the midgut of G. pyloalis was almost more than in the salivary glands, which is consistent with α- and β-glucosidase activity in Naranga aenescens L. (Lep.: Noctuidae) and Hyphantria cunea (Drury) (Lep.: Pyralidae) (unpublished data). In contrast, in N. koshunensis (Isoptera: Kalotermitidae), the highest activity was detected in the salivary glands, while lowest activity was found in the gut. In this insect, the specific activity was also highest in the salivary glands [18]. Also, Tokuda et al. [25] detected ß-glucosidase activity in the salivary glands of N. takasagoensis, more than 66% of total activity in the digestive system.
Alpha- and β-glucosidase in midgut of G. pyloalis are generally most active in neutral to slightly acidic pH conditions, but these enzymes in salivary glands are most active in slightly alkaline pH conditions. Alkaline pH optima for the assayed enzymes in the salivary glands of G. pyloalis larvae were similar to the ones stated for the majority of the examined lepidopterans [4,5]. Zibaee et al. [4], working on a C. supressalis Walker, detected α- and β-glucosidase activity between pH 3–8 and the suitable pH was observed at 8 in both midgut and salivary glands. Optimal pH for disaccharidases and specific glucosidases ranges between 5.3 for trehalase in wax moth Galleria mellonella L. (Lep.: Pyralidae) [26] and 8.0 for α-glucosidase in 3rd instar larvae of Earias vitella (Lepidoptera: Noctuidae) [27]. Ni et al. [28] showed that the optimum pH and thermostability for N. koshunensis expressed β-glucosidase were 5.0 and 45 °C, respectively. Also, in this insect, optimal pH of salivary β-glucosidase was 5.6 and the activity was stable from 20 up to 45 °C [18]. Maximal β-glucosidase activity of the palm weevil Rhynchophorus palmarum L. (Coleoptera: Curculionidae) was at 55 °C and pH 5.0 [29]. Nakonieczny et al. [6] showed that optimal pH for α-glucosidase in the larvae of Apollo butterfly, P. apollo ssp. Frankenbergeri was 4.9–5.6. These differences may reflect the phylogenetic relationship or response to different food sources [4]. Also, there may be a difference attributable to the origin of the enzyme, i.e. gut or salivary glands. Optimum pH generally corresponds to the pH prevailing in the midguts from which the α- and β-glucosidase are isolated. It has been suggested that high gut pH is a type of adaptation for insects to feed on plant materials which enrich with tannins [30]. Tannins bind to proteins at lower pH values then reducing the efficiency of digestion [31]. The G. pyloalis α- and β -glucosidase has an optimum temperature activity at 45 °C, which is consistent with other reports. Zibaee et al. [4] showed that α- and β-glucosidases of C. supresalis have an optimal activity in 45 °C for both midgut and salivary glands, respectively and activity increased steadily from 20–45 °C.
At temperatures ranging from 15 to 45 °C, the enzyme activity increased steadily, achieving the maximum at 45 °C (Fig. 5). At 55 °C, the α- and β-glucosidase activity dropped sharply to about 10 and 20% of the maximal value, respectively. Most insect α- and β-glucosidase exhibit temperature optima ranging from 20 to 50 °C [32,33]. Franzl et al. [14] reported a broad optimum between pH 3.5 and 6.5 for p-nitrophenyl-ß-D-glucoside in Zygaena trifolii Esper (Lep.: Zygaenidae) and the temperature optimum for ß-glucosidase was 40 °C.
Results showed that The CaCl2 increased significantly α-glucosidase activity. Zeng and Cohen [34] showed that Cu2+ had the most effect (118%) and Mg2+ the least effect (96%) on α- and β-glucosidase of Lygus Hesperus Fabricius (Hemiptera: Miridae). However, it has been reported that glucosidases are metalloproteins that require calcium for maximum activity. The α-glucosidase activity was enhanced with increasing in concentration of EDTA, whereas β-glucosidase activity decreased with increasing in concentration. Also, Urea (4 mM) and SDS (8 mM) decreased significantly the β-glucosidase activity. Yapi et al. [29] showed that CuCl2, ZnCl2, FeCl3 had an inhibitory effect on β-glucosidase activity in digestive fluid of larvae of the palm weevil, R. palmarum, whereas BaCl2, MgCl2, MnCl2, SrCl2 and CaCl2 had no effect on enzyme activity. Alpha- and β-glucosidase activity in both midgut and salivary glands of C. suppressalis increased with the addition of NaCl, MgCl2 and CaCl2 and decreased due to the use of different concentrations of KCl, EDTA, SDS and Urea [4].
Our results showed that the Km and Vmax values of the enzyme were 0.99 mM and 0.30 μmol−1 min−1mg protein−1, respectively (Fig. 13). Most insect α- and β-glucosidase exhibit Km ranging from 0.24–3 mM [4,35,36].
Glucosidases play an important role in insect digestion, as β-glycans are major dietary components of many insect species. The β-glucosidase acting on cyanogenic glycosides and glucosinolates all possess acidic pH optima (pH 4–6.2). Most of them are glycoproteins, and may occur in multiple forms. The degree of substrate specificity varies [37]. Also, β-glucosidases are the enzymes capable of hydrolyzing plant glycosides [11,15,38]. Proper quality and quantity of necessary nutritional compounds are the factors that ensure the developmental success of specialized herbivores; thus, their digestive functions change in parallel with their specific food source. This can be manifested in a synthesis of a limited set of digestive enzymes, the relative activities of which reflect food preferences of an insect [38–40]. Insect-resistant crops have been one of the major successes of applying plant genetic engineering technology to agriculture. Plants secondary metabolites can act as protective agents in plant against insects either by repellence or by direct toxicity. Much different types of secondary metabolites including alkaloids, terpenes, steroids, iridoid glycosides, aliphatic molecules, phenolics [41], and others, have been demonstrated to confer resistance to different plant species against insects. Among them, glycosides seem to play an important role in host plant resistance to insects. For example, Klun et al. [42] observed that when the DIMBOA (a glycoside) was isolated from the corn seedlings and bioassayed using an artificial diet for European corn borer, larval development was inhibited and 25% mortality resulted. The correlation between concentration of DIMBOA in plant tissues and level of resistance to the leaf feeding by the first brood of European corn borer was highly significant for inbreeds and single crosses [43]. The relation of DIMBOA and resistance to the corn leaf aphid was studied by Long et al. [44,45]. The DIMBOA showed a highly significant correlation with corn leaf aphid infestation. Also, high concentration of cyanogenetic glycosides phaseolunation and lotaustrin found in the tissues of Phaseolus inhibited feeding of Mexican bean beetle, Epilachna varivestris, and were toxic; suggesting that bean varieties containing high levels of glycosides might be resistant to the bean beetle [46].
Plants have developed many secondary metabolites involved in plant defense, which are collectively known as antiherbivory compounds and the study of carbohydrases in herbivorous insects is very important, not only for understanding of digestion biochemistry, but also for developing insect pest management strategies.
Acknowledgements
The authors express their gratitude to the research council of the University of Guilan and Ministry of Science, Researches, and Technology for financial support during the course of this project.