1 Introduction
Alzheimer's disease (AD) is the most common neurodegenerative disease among senile dementia. AD brains are characterized by β-amyloid peptide (βA) accumulation. This is a consequence of the cleavage of amyloid precursor protein (APP) by α- and β-secretases. Moreover, the loss of the cholinergic system efficiency plays an important role in AD. Postmortem studies of AD patients consistently have demonstrated the loss of cortical cholinergic neurons and the decrease of the enzymatic activity of choline acetyltransferase (ChAT), the enzyme responsible for acetylcholine (ACh) synthesis [1]. This decrease of cholinergic function contributes to the severity of the cognitive and behavioral deficits, especially in the brain areas of memory and attention. In addition to this feature, numerous factors are involved in the development of AD, such as cholesterol metabolism and, in particular, the apolipoprotein E (ApoE) that plays a role in the formation of the βA burden [2,3].
A credible homologous model of AD should ideally display both the behavioural characteristics of the disease and its pathology. The Tg2576 mouse was developed by Hsiao et al. as an animal model of AD [4]. It bears the “Swedish” familial AD mutation, consisting of a double amino acid substitution in the amyloid precursor protein (APP), resulting in overproduction of βA. Studies demonstrated that these transgenic mice showed behavioural impairments, as well as pathophysiological characteristics of AD, including βA deposits, amyloid plaques, neuritic dystrophy and abnormal phosphorylation of protein tau (Tubular A Unit).
Some studies suggested that chronic contamination by metals could be responsible for the development of neurodegenerative disorders. For example, increased levels of mercury have been found in patients suffering from AD [5]. Furthermore, other studies suggested that the risk of developing AD was higher in areas where drinking water contains a high concentration of aluminium (between 0.1 and 0.2 mg/L) [6,7]. Uranium is another heavy metal, and because of its high density, it is widely used in military as well as in civilian applications and its utilization has spread almost worldwide. Uranium has a double toxicity: a radiological toxicity and a chemical toxicity [8]. In order to avoid radiological toxicity, depleted uranium (DU), with very weak radioactive activity, has been chosen in this study. Little information is available concerning the potential health hazard of chronic ingestion of low amounts of DU. Ingestion of DU may result in the manifestation of toxicity in many parts of the body [9]. The most serious concern is DU toxicity in the kidneys [10], but more recently the central nervous system has also appeared as a sensitive target organ to DU exposure [11].
Previous studies have demonstrated that DU chronically ingested modifies the cholinergic system [12–14] and the brain cholesterol metabolism [15] in normal rats, but without neurological disorders. However, the question to know if DU could worsen the development of the pathology in organisms that will develop the Alzheimer disease is still open. The effects of a chronic ingestion of DU through drinking water (20 mg/L) over an 8-month period were analyzed on ACh and cholesterol metabolisms at gene expression level in the cerebral cortex of APP transgenic mice (Tg2576). In order to characterize the transgenic model, a comparison between wild-type and Tg2576 mice has been also made on cholinergic and cholesterol systems.
2 Materials and methods
2.1 Animals and contamination procedure
The transgenic APP mice used in this study contain the APP695 human gene, which was inserted into a hamster prion protein cosmid vector [4]. This gene with the Swedish mutation is known to be responsible for a familial form of AD [16]. The mice were supplied by Taconic US (Germantown, NY, USA).
Tg2576 (n = 16) male mice, aged 12–13 weeks, were housed 4 per cage for a period of 8 months. The animals were kept on a 12 h/12 h light/darkness cycle at 21 ± 2 °C. After a one-week acclimatization period, animals were distributed in two groups of 8 mice. One group of Tg2576 mice was exposed to depleted uranyl nitrate hexahydrate (98.74% 238U, 0.26% 235U, 0.001% 234U, specific activity 1.4 × 104 Bq/g, AREVA-NC, France) at a dose of 20 mg/L (280 Bq/L) in their drinking water for 8 months. The second (“control”) group of Tg2576 mice received mineral water. A third group, the wild-type mice (n = 8), has been constituted and drank mineral water for 8 months.
The water DU content was close to maximum uranium levels found naturally in some areas, such as southern Finland [17]. At the end of exposure, mice were 10.5 months old, corresponding to the time when behavioral impairments are just beginning to set in [18]. All groups were fed a regular rodent chow, with water and food ad libitum.
Throughout the experiment, body weight gain and food and water intake were measured weekly. After the contamination, the animals were anaesthetized and decapitated. The brain was removed and frontal cortex was dissected on ice. Samples were immediately deep-frozen in liquid nitrogen and stored at −80 °C until analysis.
All experiments were approved by the IRSN Animal Care Committee and complied with French legislation concerning animal experimentation (Ministry of Agriculture Act No. 87-848, October 19, 1987, amended May 29, 2001).
2.2 Real-time PCR
Total RNA was extracted with the RNeasy Lipid Tissue Mini-Kit (Qiagen, Courtaboeuf, France). The cDNA was produced from 1 μg of total RNA by reverse transcription with BD Sprint PowerScript PrePrimed 96 plate (BD Biosciences Clontech, Erembodegem, Belgium). Real-time PCR was performed on an AbiPrism 7900 Sequence Detection System (Applied Biosystems, Courtaboeuf, France) using SYBR Green technology, with 16 ng of template cDNA for each reaction. Thermoprofile conditions were: 50 °C for 2 min, 95 °C for 10 min and 40 cycles at 95 °C for 15 s and 60 °C for 1 min. PCR assays were done in duplicate. The comparative ΔΔCT-method was used for relative mRNA quantification [19]. Samples were normalized to hypoxanthine-guanine phosphoribosyltransferase (HPRT). Primer sequence of HPRT was found in the study of Ropenga et al., 2004 [20] and the other sequences were designed with PrimerExpress software and fold-inductions calculated relative to the Tg2576 control group. Sequences for the primers are indicated in Table 1.
Oligonucleotide sequences of primers used for real-time qPCR.
Gene | Foward | Reverse | Length (bp) | Gene accession # |
HPRT | AAACTTTGCTTTCCCTGGTTA | AGGCTTTGTATTTGGCTTTTC | 225 | NM_013556.2 |
HMGR | TCGCTGGATAGCTGATCCTTCT | TTCGTCCAGACCCAAGGAAAC | 73 | NM_008255 |
HMGS | TACCTGCGGGCCTTGGAT | GAGGGTGAAAGGCTGGTTGTT | 93 | NM_008256 |
CYP46A1 | CTGCATCGGCCAACAGTTT | CAATGGCTTGAGCGTAGCC | 130 | NM_010010 |
α-secretase | CGCCACAGCCCATTCAG | TCGCATGTGTCCCATTTGAT | 68 | NM_007399 |
β-secretase | CATTGCTGCCATCACTCAAT | CAGTGCCTCAGTCTGGTTGA | 208 | NM_011792 |
LXRβ | GATCGGATCCATGTCTTCTTCCCCCACAAGTTC | GATCCTCGAGTAAGATGACCACGATGTAGG | 159 | NM_009473 |
RXRα | CGCAAAGACCTGACCTACACC | TCCTCCTGCACAGCTTCCC | 134 | NM_011305 |
PPARγ | TCATGACCAGGGAGTTCCTCA | TCATCTAATTCCAGTGCATTGAACTT | 103 | NM_011146 |
SREBP 1c | CCAGAGGGTGAGCCTGACAA | CACTAAGGTGCCTACAGAGCAAGAG | 116 | NM_011480 |
ApoE | TGGCTACCAACCCCATCATC | TTGCAGGACAGGAGAAGGATACT | 69 | NM_009696 |
ABC A1 | ATCTCATAGTATGGAAGAATGTGAAGCT | CGTACAACTATTGTATAACCATCTCCAAA | 132 | NM_013454 |
ABC G4 | GGCCACCACTCCCAATCC | TCTATCCAGACCGAAGGCTTAGG | 79 | NM_138955 |
SR-B1 | GTTGGTCACCATGGGCCA | CGTAGCCCCACAGGATCTCA | 61 | NM_016741 |
LDLr | TCTGCCCCAGGTTCTGTTTTAT | CAAGGAAATGAAGATGGGAGAAA | 118 | NM_010700 |
ChAT | GAGCGAATCGTTGGTATGACAA | AGGACGATGCCATCAAAAGG | 72 | NM_009891 |
VAChT | GGGTCGGCTCGGTCAATC | CAAATAGCACGCCTATCTTCACAT | 146 | NM_021712 |
nAChR α5 | CAACATCCACCACCGCTCTT | TTTGGGAAGCTTGTGGAGAAAT | 81 | NM_176844 |
nAChR β2 | TCGTCGCAAACCGCTCTT | GATGGCCAGCGAGGTGAT | 69 | NM_009602 |
m1AChR | CCCCTGGGTCACCTTCCT | TTCCCCGGGTTTCACTCTCT | 100 | NM_001112697 |
AChE | CCTGGATCCCTCGCTGAA | CCTGTGCGGGCAAAATTG | 65 | NM_009599 |
BuChE | GTTTTCTGCAGTGAGTGACAGGTATT | CAGAATCTGGATGCTGAAGTAGATAA | 89 | NM_009738 |
2.3 Statistical analysis
Results are reported as means ± SEM. Statistical analyses were performed with Student's t-test. Differences were considered significant when p < 0.05, p < 0.01 or p < 0.001.
3 Results and discussion
The cholinergic system is among the classical neuron-modulation system of the brain. In AD, an early and progressive loss of cholinergic neurons has been described [21]. This loss has been suggested to cause the cognitive impairments observed in this neurodegenerative disease. In control Tg2576 mice, the mRNA levels of choline acetyl transferase (ChAT), the biosynthesis enzyme of ACh, and the vesicular ACh transporter (VAChT) are lowered (decrease of respectively 89 and 86%, p < 0.01) in comparison with the wild-type mice (Fig. 1A). Concerning ChAT, a decrease of the enzymatic activity has been previously described in AD [22]. Previous studies showed no significant difference between transgenic and wild-type mice for VAChT, but these results are not at gene expression level [23,24]. All others genes of the cholinergic system were not significantly modified in control Tg2576 mice in comparison to wild-type mice (Fig. 1A). These results suggest that ChAT and VAChT seem to be specific target genes in AD, at least in this AD model.
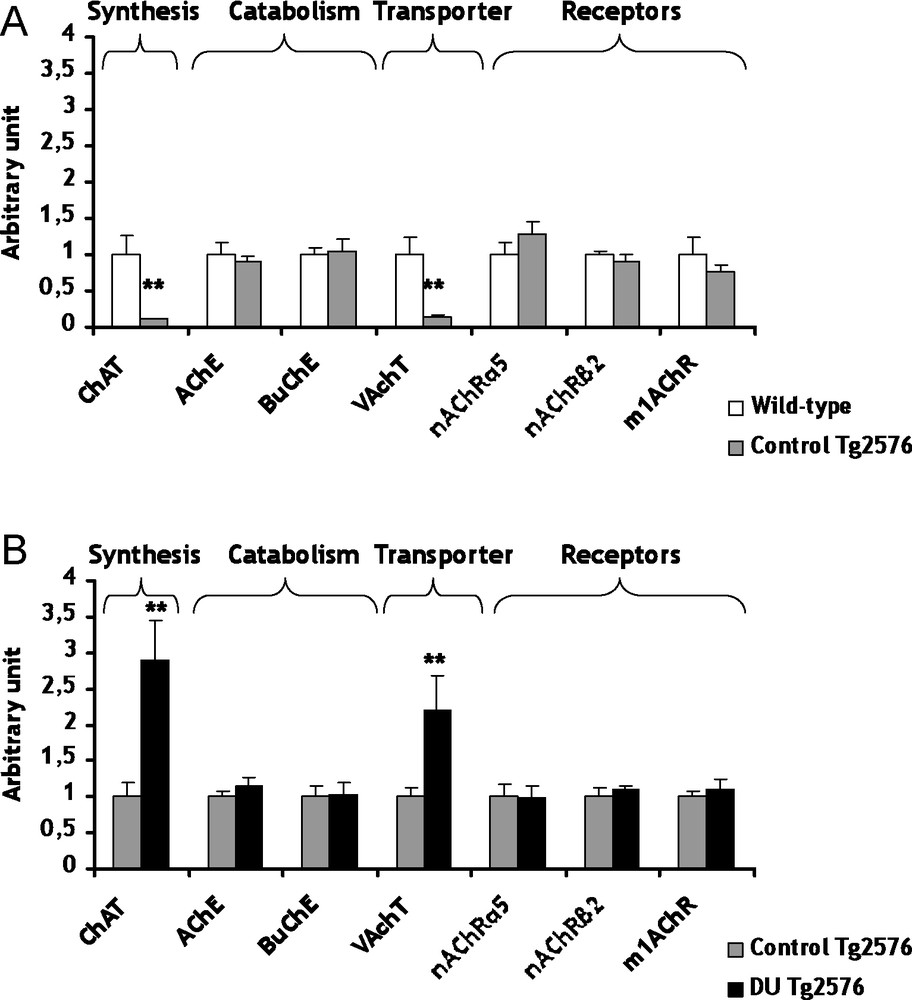
Gene expression of biosynthesis and catabolism enzymes, transporters and receptors involved in cholinergic system within cerebral cortex of control Tg2576 mice (A) and DU Tg2576 mice (B). Results are expressed as a ratio to HPRT mRNA level. A. The levels of wild-type mice were arbitrarily set at 1. B. The levels of control Tg2576 mice were arbitrarily set at 1. Data are expressed as means ± SEM (n = 8 for each group), with **p < 0.01, significantly different from wild-type mice or control Tg2576 mice; DU: depleted uranium.
Conversely to the cholinergic system which appears as a consequence of the disease, cholesterol metabolism seems more and more evidently involved in the genesis of AD [25]. The cerebral cholesterol is independent from the body pool since cholesterol cannot efficiently cross the blood-brain barrier. Therefore, the brain must produce its own cholesterol endogenously and maintain its homeostasis. However, there is an intracerebral transport of cholesterol between glial and neuronal cells. Among these transporters, only the gene expression of ATP-Binding Cassette A1 (ABC A1) decreased significantly (−44%, p < 0.001) in Tg2576 mice in comparison to wild-type mice (Fig. 2A). The gene expression modulation of ABC A1 relates to the work of Wahrle et al. showing that an over-expression of ABC A1 protein decreases the precipitation of ßA peptides [26]. These results demonstrated that the decrease of ABC A1 is in agreement with the development of the AD. All others genes of the cholesterol metabolism were not significantly modified in control Tg2576 mice in comparison to wild-type mice (Fig. 2A).
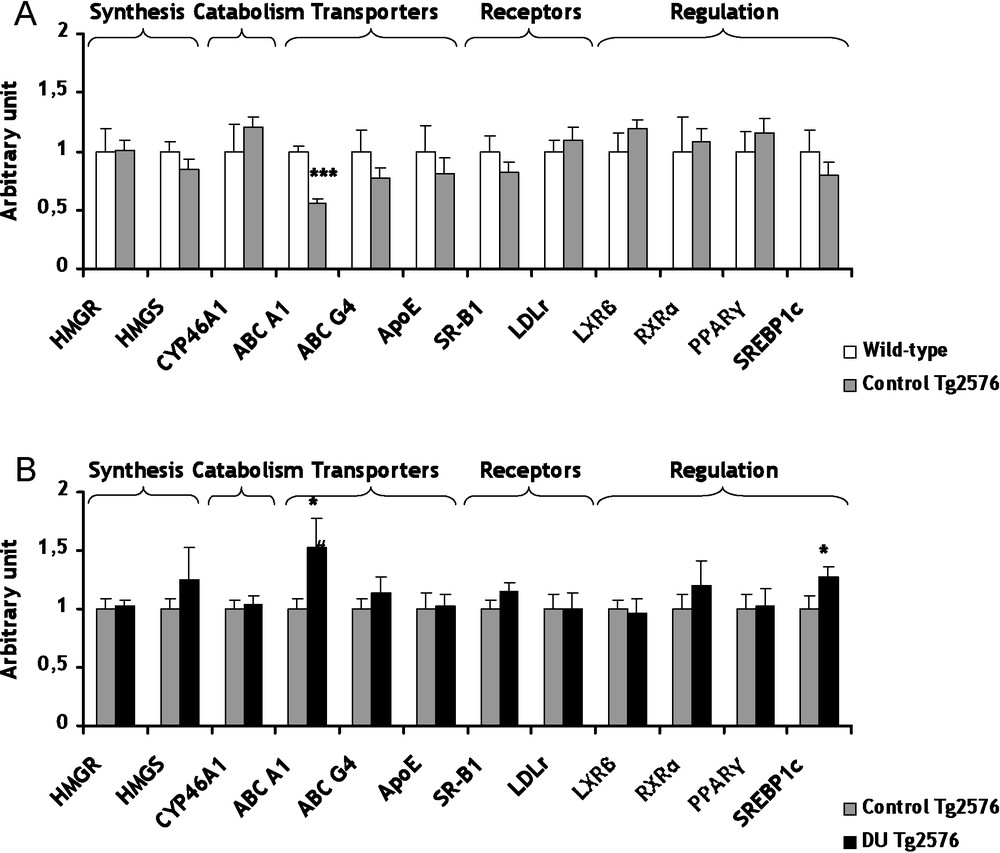
mRNA levels of biosynthesis and catabolism enzymes, transporters, lipoprotein receptors and transcription factors/nuclear receptor involved in cerebral cholesterol metabolism of control Tg2576 mice (A) and DU Tg2576 mice (B). Results are expressed as a ratio to HPRT mRNA level. A. The levels of wild-type mice were arbitrarily set at 1. B. The levels of control Tg2576 mice were arbitrarily set at 1. Data are expressed as means ± SEM (n = 8 for each group), with ***p < 0.001, significantly different from wild-type mice and *p < 0.05, significantly different from control Tg2576 mice; DU: depleted uranium.
The development of AD could be perhaps linked to some heavy metals [5–7]. In light of this observation, we have considered to study the possible adverse consequences of a chronic exposure to DU in Tg2576 mice. Concerning the cholinergic system, the mRNA levels of ChAT and VAChT are increased (+189 and +120% respectively, p < 0.01) in Tg2576 mice exposed to DU in comparison with the control Tg2576 mice (Fig. 1B). Two enzymes participate to the catabolism of ACh, i.e. the acetylcholinesterase (AChE) and the butyrylcholinesterase (BuChE). The exposure of Tg2576 mice to DU did not induce significant modifications of mRNA levels of AChE and BuChE, but their enzymatic activities remain to be determined (Fig. 1B). Earlier studies performed on rats exposed to uranium produced apparently contradictory results on AChE. An increase of AChE activity was observed after a 1 mg/kg per day intramuscular injection of DU over a 7-day period [12], whereas a decrease of AChE activity was shown in entorhinal cortex [14] and no modification in the hippocampus [13] after chronic contamination of drinking water with DU (40 mg/L). The different contamination protocols (specific uranium salts, dose, exposure, duration, cerebral structure) used in these studies may explain this discrepancy. Concerning the gene expression of cholinergic receptors (α5nAChR, β2nAChR and m1AChR), none was significantly modified in Tg2576 exposed mice in comparison with control Tg2576 mice (Fig. 1B). Nevertheless, few investigations showed genic modulations of nicotinic and muscarinic receptors by heavy metals, such as mercury chloride and zinc exposures [27–29]. The results obtained in the present study suggest for the first time that chronic ingestion of DU in Tg2576 mice induces some modifications of the cholinergic pathway. This hypothesis must be confirmed in the future with other time of exposure and/or other DU doses.
Concerning cholesterol, a chronic internal contamination with DU during 8 months induced no significant effect on gene expression of two biosynthesis enzymes, 3-hydroxy-3-methylglutamyl Coenzyme A Synthase (HMGCoA Synthase) and HMGCoA Reductase (Fig. 2B). To prevent its excessive accumulation of cholesterol in the brain, cholesterol is converted into 24(S)-hydroxycholesterol by cholesterol-24-hydroxylase (CYP46A1), a brain-specific enzyme. This oxysterol being more hydrophilic than cholesterol, it can cross the blood-brain barrier more rapidly and join the general bloodstream [30]. A strong relationship between CYP46A1 and AD has been demonstrated [31]. After DU exposure, gene expression of CYP46A1 was unchanged in Tg2576 mice in comparison to control (Fig. 2B). Among proteins involved in transport of cholesterol between glial and neuronal cells, only the mRNA level of ABC A1 increased significantly (+52%, p < 0.05) in Tg2576 mice exposed to DU in comparison to control Tg2576 mice (Fig. 2B). This increase of ABC A1 is in agreement with a previous study performed on wild-type rats after 9 months of exposure to DU [15]. Gene expression of low-density-lipoprotein receptor (LDLr) and scavenger receptor class B type 1 (SR-B1), which both take up the cholesterol into the cell, were not modified in Tg2576 exposed mice compared to control Tg2576 mice (Fig. 2B). A previous work has demonstrated a significant increase of mRNA level of SR-B1 after chronic exposure in rat [15]. Further studies are necessary since this discrepancy between our data and those of Racine et al. cannot be explained by the only difference of species. Cholesterol metabolism is submitted to a very fine and intricate regulation at transcriptional level implying a number of nuclear receptors. In Tg2576 mice, a chronic exposure to DU increased mRNA levels of Sterol Regulatory Element-Binding Protein 1c (SREBP 1c) by 27% (p < 0.05) compared to non-exposed Tg2576 mice (Fig. 2B). This result is in accordance with previous studies showing that some nuclear receptors are affected by DU chronic internal exposure [32,33]. In our experimental conditions, other nuclear receptors, i.e. RXRα and PPARγ, were not significantly modified in Tg2576 exposed mice in comparison with control Tg2576 mice (Fig. 2B). All these results suggest that the global homeostasis of cholesterol in the brain is maintained in Tg2576 mice after DU exposure. Only gene expression of ABC A1 was significantly modified by DU. ABC A1 seems to be a target gene in since its expression is modified both in the pathological phenotype and after DU exposure, as for CAT and VAChT.
The homeostasis of essential metals such as copper, iron, selenium and zinc may be altered in the brain of subjects with AD [34]. The decreases of VAChT, ChAT and ABC A1 observed in Tg2576 mice in comparison to wild-type mice are reversible when Tg2576 mice were exposed to DU. A strong link between iron homeostasis and uranium existed [35]. Previous studies have described transferrin and ferritin as uranium transporters [36]. Thus, iron and uranium are suspected to be transported by the same transporters. These studies suggest a possible competition between uranium and iron at the cellular level and could participate to the effects observed in the present study.
In conclusion, this study demonstrated for the first time that a chronic exposure by ingestion of a low dose of DU induces changes in the expression of genes implicated in the physiopathology of AD. ChAT, VAChT and ABC A1 mRNA levels are lowered in the pathological model and are modulated by DU exposure. These modulations induced by DU did not lead to the increased disturbances that are characteristic of declared AD, suggesting that chronic DU exposure did not amplified this pathology. The link between uranium and iron could perhaps participate in these effects. All these results were obtained from gene expression analyses only, and must be confirmed by assays of protein levels and enzymatic activities. Chronic ingestion of uranium did not induce any change in the size and density of senile plaques (personal data), suggesting that contamination did not worsen the pathology. In order to conclude more definitively on the harmlessness of low-dose chronic DU contamination on the development of the disease, it appears necessary to launch further experiments with longer contamination times. Finally, these results raise other issues, such as the effects of natural uranium, which is naturally present in drinking water and more radioactive than DU, on the development of the disease.
Acknowledgements
The authors thank Karine Ser-le-Roux for mice euthanasia and cerebral cortex harvest, and Cédric Baudelin and Thierry Loiseau for their technical help. This work is part of the ENVIRHOM-Health research program supported by the Institute for Radiological Protection and Nuclear Safety.