1 Introduction
Biodiversity is central for the resilience of ecosystems, as it provides a buffering effect against stochastic or environmental changes affecting some or all of the communities within an ecosystem [1,2]. In agricultural-landscapes, agrobiodiversity provides a similar stabilizing property to agroecosystems created by humans. A straightforward definition of agrobiodiversity includes the number of species and the number of varieties (races) cultivated (reared) in a production basin or on a farm, and also encompasses all the wild species associated with domesticated ones. Such an associated biodiversity may include a wide variety of organisms, from birds, plants and insects present in fields to worms and microorganisms living in the soil, both those considered pests and those considered beneficial to humans [3].
However, this narrow definition can be broadened to encompass all mechanisms maintaining this diversity, in particular, local knowledge associated with crop species that contribute to anchor a specific diversity in a specific landscape [4,5]. Indeed, resilience for one component of agrobiodiversity (i.e. the number of species and varieties cultivated) was found to be positively correlated with farm profitability [6,7], as biodiversity provides ways to cope with the uncertainties of highly variable environments [8]. Diversity is also maintained by farmers for cultural and aesthetic purposes [9–11], indicating that cultural and social diversity is another driving force that contributes to agrobiodiversity conservation.
Therefore, the management of genetic variability within each cultivated crop is a first point of leverage when managing agrobiodiversity. The importance of intra-specific variability has been stressed in the context of developing countries in which the control of agro-climatic hazards by agricultural practices is not practical due to economic or other constraints [12]. However, with the development of low-input agriculture in industrialized countries, for economic and environmental reasons, the need to cope with more variable conditions also becomes critical. In this context, it has been suggested that a new approach for a sustainable agriculture should be based on a better use of the ecological services of agroecosystems, including biodiversity [13]. One strategy to enhance within-species variability relies on the dynamic management (DM) of genetic resources. DM aims at conserving a reservoir of genetic variability rather than specific alleles at a locus or some cultivars that are already genetically fixed. Its principle is to maintain the context in which evolutionary forces can act on genetically diverse cultivated populations so that they adapt to changing climatic conditions, emerging diseases and agricultural practices. The efficiency of DM thus relies on the conservation of a large number of populations, distributed over a large range of contrasted environments, maximizing the diversity in local selective pressures [14–18].
Although, DM was first proposed in 1984 [19] outside the context of conservation biology, it can be considered a form of in situ conservation. Here we propose to set this approach in the context of the recognized framework for biodiversity conservation. According to the Convention on Biological Biodiversity, “in situ means the conservation of ecosystems and natural habitats and the maintenance and recovery of viable populations of species in their natural surroundings and, in the case of domesticated or cultivated species, in the surroundings where they have developed their distinctive properties” (UNCED 1992). This latter case is usually considered to be on-farm conservation [20]. An alternative to on-farm conservation is the management of diverse populations cultivated in experimental stations by public institutions where the populations are grown in homogenized agronomic conditions and mainly submitted to natural selective pressures since they are not inserted in an actual agricultural production system.
In this article, we analyse two particular DM systems, which coexist in an industrial agriculture context in France:
- • one institution-led management program (experimental DM);
- • one farmer-led management system (on-farm DM).
The objectives are first to review strengths and weaknesses of both approaches. The findings on population evolution and adaptation from the use of experimental DM will be summarized in the first section of this paper. The second part will discuss current work using an on-farm DM strategy with a network of organic farmers in France. In the last section, we propose a general scheme for agrobiodiversity conservation taking into account ex situ conservation issue.
2 Experimental dynamic management: what can be learned from a 26-year program?
In 1984, the French National Institute for Agronomic Research (Inra) initiated a pilot program of DM of wheat (Triticum aestivum L.) genetic diversity, based on the continuous cultivation of three different segregating populations (PA, PB and PS) in a multi-location French network of research stations and agricultural high-schools (12 locations, Fig. 1). Populations PA and PB, predominantly selfing (6% outcrossing), were obtained through a pyramidal cross of two different sets of 16 pure lines of wheat. The PA parental lines were selected to provide a balance between a high agronomic value in Northern-French conditions and a high disease resistance (yellow rust, oïdium) [21], while PB parents were of a broader genetic base, with more exotic lines. Population PS was derived from the crossing of 62 French and worldwide wheat lines with Probus, a cultivar carrying the ms1b autosomal male sterility mutation and was conducted as outcrossing by harvesting only the male-sterile plants [22]. Dwarfism genes were present in disjunction in all three initial populations. Since then, each population has been grown each year under two farming conditions in the same location, with 5,000 to 10,000 individuals in a 100 m2 plot isolated from other wheat fields and without any conscious human selection. A rapid evolution of some major life-history traits was detected in the network after just a few generations of cultivation.
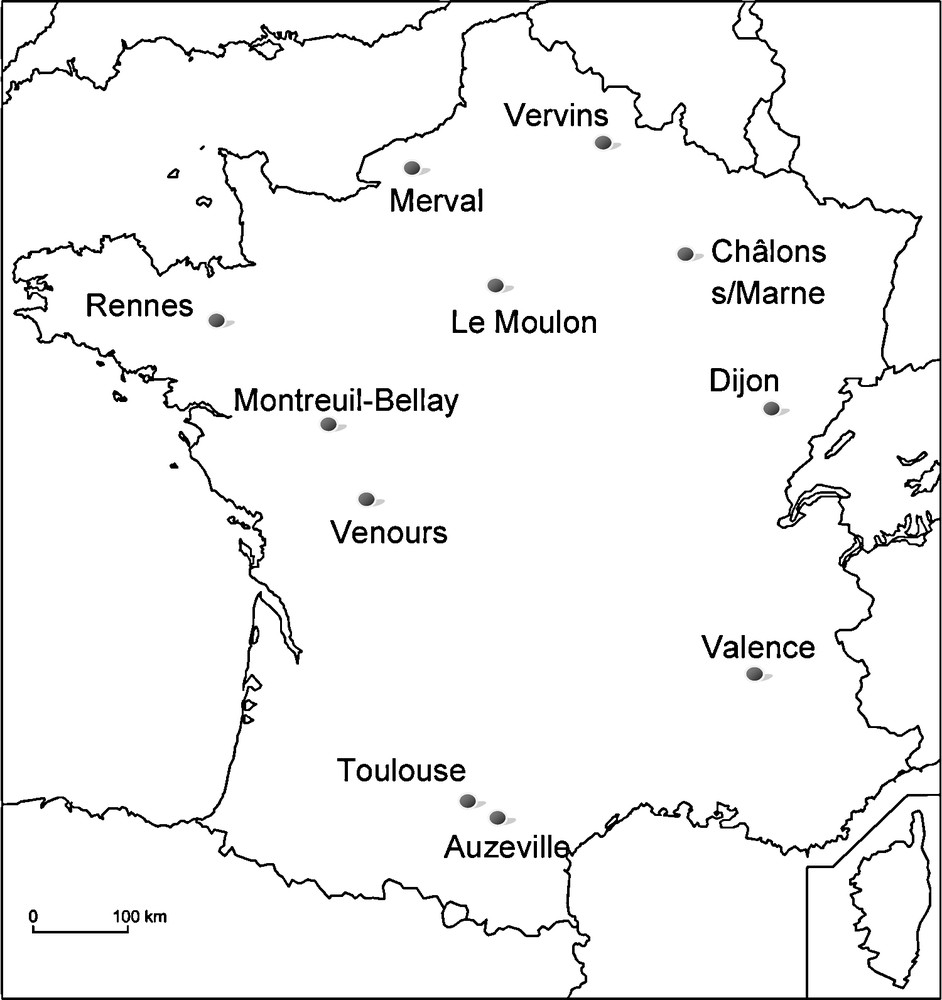
Network of sites initially involved in the experimental dynamic management of wheat genetic resources. The 12 sites were composed of Inra research stations and agricultural high-schools.
2.1 Evolution for adaptive traits in wheat experimental dynamic management populations
2.1.1 Uniform evolution of plant height
One of the most drastic evolutions in the experimental DM program was the increase in plant height. In all populations of all sites, and in the two PA and PB pools, a significant increase in mean plant height was observed when compared to the initial populations [23–25]. After only four generations of cultivation, the mean plant height increased by 10 cm [23], and by 20 cm after eight generations [26]. This increase was interpreted as the result of competition among individuals for light in each local population. This evolution was rapid because of the segregation of dwarfing genes in the two initial populations, which meant there was a large genetic variance in these populations for plant height.
2.1.2 Divergent evolution for earliness
Earliness assessed through flowering time or heading date is a major adaptive trait that allows plants to synchronize their reproduction with appropriate climatic conditions. Spatio-temporal evolution of earliness and its components was investigated in this long-term multi-location experiment [27]. The three components studied were: partial vernalization sensitivity, day length sensitivity and earliness per se (which determines flowering time independently of environmental stimuli). The heading time of six PA populations was evaluated in the tenth generation under different vernalization and photoperiodic conditions. Populations of generation ten were found to be genetically differentiated along a North–South latitudinal trend for two components out of the three studied: partial vernalization sensitivity and narrow-sense earliness. The populations cultivated in the South became earlier (by ∼45 °C days, i.e. 2.5 days) than the populations in the North; the frequency of winter type plants (with high vernalization requirement) was lower in the southern populations (less than 50%) than in the northern ones (around 90%), indicating that local climatic conditions greatly influenced the evolution of population earliness traits. This result also suggested that diversity was on average conserved during evolution but was differently distributed by natural selection and possibly subject to drift [27].
The evolution of flowering time was studied more finely in three PA populations grown in contrasting environments (in Northern and Southern France) for 12 generations, by comparing the distribution of phenotypic and presumably neutral variation (QST vs FST contrast). Divergent selection was detected on flowering time after seven and 12 generations. From non-significant phenotypic differentiation in generation two (QST = 0.12 vs FST = 0.08), differentiation became significant in generation seven (QST = 0.31 vs FST = 0.08) and was the largest in generation 12 (QST = 0.48 vs FST = 0.14) [28].
Reciprocal transplant experiments between populations from three sites (Le Moulon, Rennes and Toulouse; Fig. 1) associated to migrant-resident models have shown that populations from Toulouse were locally adapted for kernel weight, which is one component of individual plant fitness [29]. Toulouse populations had, on average, higher values for this trait when they were evaluated in Toulouse compared to other populations in the same site and compared to their own trait values in other sites. Flowering time was negatively correlated with kernel weight in the southern site (Toulouse), while positively correlated in the northern sites (Le Moulon and Rennes). Late flowering in the South implies that kernel fill occurs during the dry period at the end of summer, which leads to a less efficient kernel maturation. We therefore hypothesize that earlier genotypes were positively selected in the southern site due to this constraint on kernel maturation.
2.1.3 Evolution of disease resistance
Resistance to four diseases (powdery mildew, stripe rust, leaf rust and eyespot) was characterized in PA populations after ten generations [15]. Adult mean resistance to powdery mildew, stripe rust and leaf rust were maintained at the global scale (Fig. 2). The genetic variance for these traits evolved slightly (Fig. 3), but none of the observed variations were significant. The evolution of adult plant resistance towards powdery mildew (caused by Blumeria graminis f. sp. tritici) was investigated in 11 populations that showed spatial differentiation. The populations with the highest resistance level originated from sites where powdery mildew pressure is known to be high (the North and West of France), whereas populations with the lowest adult plant resistance corresponded to areas with no or very low powdery mildew pressure (the South and South-West of France).
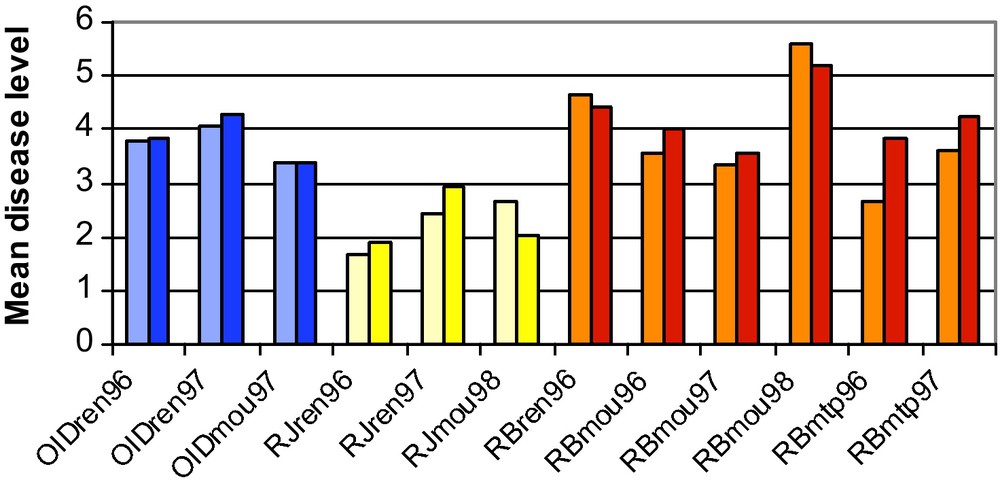
Comparison of average levels of disease incidence in the initial PA0 population (light colours) and the evolved populations (dark colours) for different diseases in different year of experimentation. The experiment codes are as follows: OID: powdery mildew, RJ: yellow rust, RB: leaf rust; ren, mou, mtp: experimental sites of Rennes, Le Moulon and Montpellier, respectively; 96, 97, 98: year of experimentation.
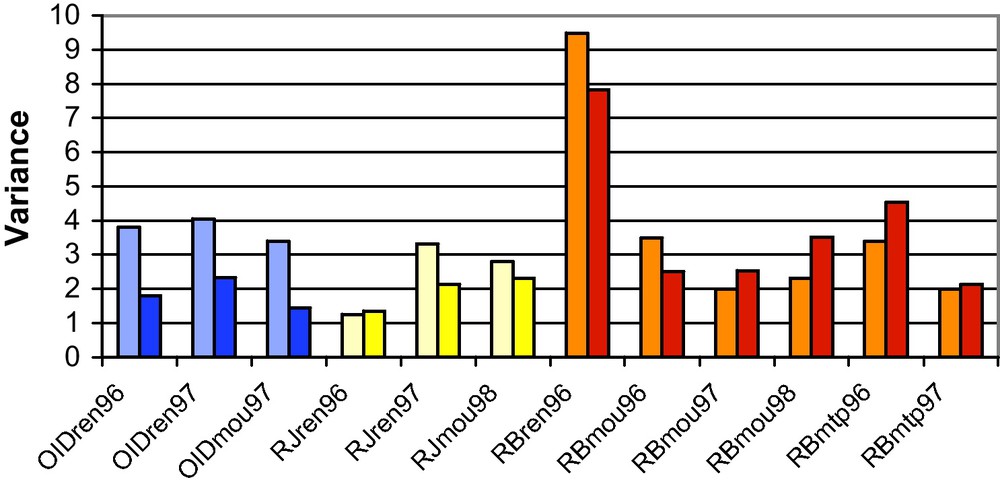
Comparison of genetic variances for levels of disease incidence in the initial PA0 population (light colours) and the evolved populations (dark colours) for different diseases in different years of experimentation. The experiment codes are as follows: OID: powdery mildew, RJ: yellow rust, RB: leaf rust; ren, mou, mtp: experimental sites of Rennes, Le Moulon and Montpellier respectively; 96, 97, 98: years of experimentation.
2.2 Structure and maintenance of genetic diversity at neutral markers and candidate genes
Both inter-individual competition and local adaptation led to rapid and strong evolution for major adaptive traits such as plant height, earliness traits and disease resistances. Depending on the genetic architecture of these traits, selective pressures may have varying consequences on the allelic frequencies of major genes involved in their determination. Selective pressures are also expected to affect neutral genetic diversity, both around genes under selection due to hitch-hiking effect [30], and at the whole genome scale as a consequence of the reduction in genetic effective population size [25].
2.2.1 The neutral genetic structure
Neutral diversity evolution was assessed in the populations using both RFLP and microsatellite markers located throughout the entire genome [13,31–33]. As expected, we found a high level of differentiation between populations, due to drift and isolation, showing that overall, a high level of genetic diversity is maintained in the network of populations. Depending on the populations studied, the FST ranged from 0.04 (estimated with 24 RFLP markers, between three PA populations at generation 10) to 0.14 (estimated with 19 microsatellites markers between three different PA populations at generation 12) (Table 1). These values were much higher than expected in diverging populations evolving during 12 generations in isolation under drift alone (FST = 1–e−t/2N = 0.002 where t = 12 generations and N = 2650 individuals, demographic effective population size). The difference between observed and expected genetic differentiation suggested that selective pressures acting on phenotypic traits affected the entire genome diversity. The effect of selection was also illustrated by the low genetic effective sizes (from Ne = 31 to Ne = 187, Table 1) estimated from temporal variation at RFLP and microsatellite markers in several populations compared to the expected demographic effective size (Ne = 2650). Since the number of individuals in a given population was constant over generations, only variation in the contribution of each individual to the next generation can explain such a reduction in genetic effective population size. Moreover, we showed that the variation in progeny number should be inherited, i.e. progeny number should be correlated over generations [25]. These selection effects at the whole genome level were strengthened due to the predominant self-fertilisation of wheat. In PA and PB pools, heterozygosity due to the initial pyramidal crosses was rapidly lost and each population consisted mainly of a mixture of homozygous lines after a few generations of evolution. Such a structure has been also described in natural selfing species such as Medicago [34]. In such a population structure, the quasi absence of genetic segregation between parents and offspring leads to a high heritability in phenotypic traits and thus to a strong response to selection.
Range of genetic divergence FST and effective population size Ne estimated in the DM populations with different markers (RFLP and microsatellites (SSR)) and for different number of generations.
Populations | Number of populations | Generation | Markers | F ST | N e a | Reference |
PA | 3 | 10 | RFLP | 0.039 | 56–187 | [31] |
PB | 3 | 10 | RFLP | 0.114 | 37–150 | |
PA | 3 | 12 | SSR | 0.14 | 40–150 | [28] |
PA | 3 | 7 | SSR | 0.08 | 37–186 | |
PA | 3 | 2 | SSR | 0.08 | 9–92 | |
PA | 6 | 12 | SSR | 0.08 | 52–134 | [33] |
PA | 1 | 17 | SSR | – | 167 | [32] |
a Range of Ne estimated in the different populations, based on temporal variation of allelic frequencies between initial and the considered generation.
2.2.2 Genetic diversity at and around candidate genes
2.2.2.1 Plant height
The rapid increase in plant height was associated with a large reduction in the frequency of dwarfing genes. When studying the evolution of the reduced height locus Rht-B1 (Rht1), the frequency of the dwarf allele dropped from 34 to 1% over 17 generations in the PA population of Le Moulon [32]. This selection against the dwarf allele explained 15% of the total evolution of the trait but interestingly reduced genetic diversity around the gene mildly and only in the close vicinity of Rht1 (3cM, [32]). This limited hitch-hiking effect due to selection on Rht1 locus was explained both by the low linkage disequilibrium in the initial recombined population, and also by the high initial frequency of the tall allele, leading to a soft selective sweep [35].
2.2.2.2 Flowering time
In contrast to the directional selection on Rht1 loci, flowering time experienced divergent selection in response to climatic conditions. In a study of candidate gene polymorphisms in three PA populations evolving in contrasted climatic conditions, Rhoné et al. [13] found a high level of genotypic variation within the three homeologous copies of the VRN-1 gene associated with rapid phenotypic changes observed after 12 generations of evolution. In each studied environment, different allelic combinations were selected, which maintained a high level of inter-population diversity [36]. A large diversity was also observed within two populations out of three in relation with the emergence of new alleles not detected in the parental lines. This high intra-population diversity could also be due to balancing selection pressures resulting from spatio-temporal variations or frequency-dependant selection.
2.2.2.3 Disease resistance
The Yr17 (resistance towards yellow rust, Puccinia striiformis fsp tritici) and Lr37 (resistance towards leaf rust, Puccinia triticina) genes are tightly linked and were introgressed from Aegilops ventricosa, a wild relative, into the wheat genome. The frequency of the Yr17-Lr37 introgression increased not only at the global level but also in nearly all the studied populations. Compared to the initial population, a significant increase in the frequency of these resistance genes was observed in the experimental DM populations, in particular within two populations that had been cultivated in Toulouse (Southern France) and Vervins (Northern France). The increase in the frequency of these genes can reasonably be explained by yellow rust (Yr17) and/or leaf rust (Lr37) selective pressures (Fig. 4). Indeed, neither of these two genes was defeated in France from 1984 to 1994, and field trials confirmed the importance of the effects of these genes in adult field resistance (in particular, Lr37 explained more than 40% of the phenotypic variance for the leaf rust resistance trait in nearly all the field trials) [37]. In Northern France, both diseases were present and both pathogen populations could possibly contribute to the selection of the Yr17-Lr37 introgression, whereas in Toulouse, only leaf rust pathogen pressure could select for the introgression, because no yellow rust epidemic occurred in this part of France between 1984 and 1994. A more recent study confirmed that the Yr17 gene had been selected between generations five and 10 in the Moulon population (selection coefficient = 0.13) and demonstrated a significant correlation between the evolution of the gene frequency and the evolution of pathogen populations across 17 generations [38].
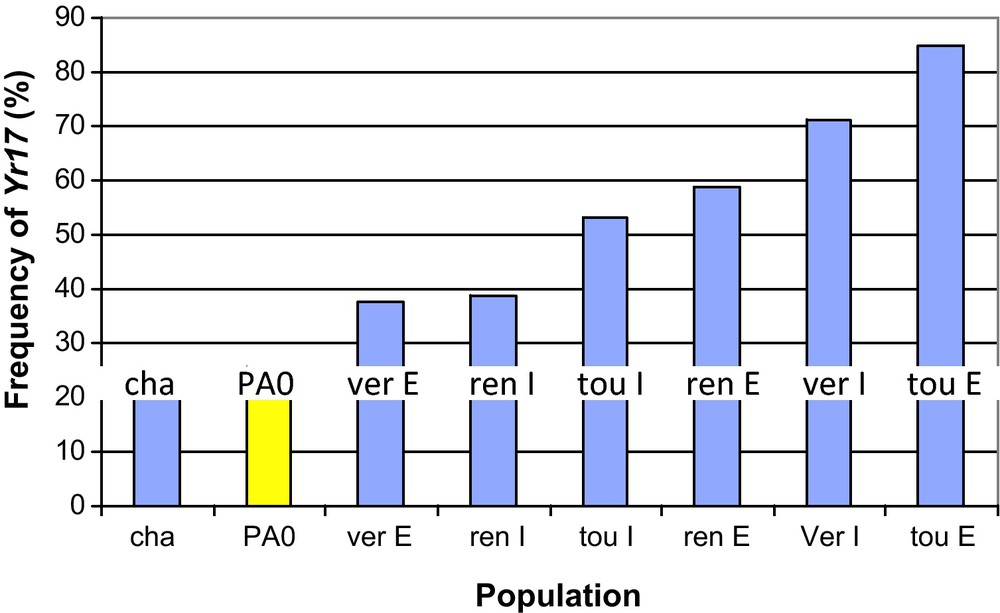
Frequency of Yr17 in the initial population (PA0, yellow) and the final populations (blue) after 10 years of cultivation. Stars indicate frequencies significantly different from the initial population frequency (P < 0.05). The experiment codes are as follows; cultivation sites: cha: Chalons, ren: Rennes, tou: Toulouse, ver: Vervins; cultural practices: E: extensive, I: Intensive (intermediate for Chalons site).
2.3 Are seed migrations necessary to maintain adaptive potentials in experimental dynamic management?
Despite the recent nature of the experimental DM program, with only 26 generations of cultivation compared to the scale of natural evolution, a highly significant evolution was observed for major adaptive traits. Local adaptation is one of the expected mechanisms driving the phenotypic divergence of sub-populations of the experimental DM program, but its expected effect in the long term is a local reduction in genetic diversity. While this reduction is expected to be reinforced by the high homozygosity of selfing populations, no drastic decrease in variability was observed within the experimental DM populations. This is probably due to the low number of generations of evolution, as well as to the residual outcrossing rate of wheat (6%, [39]) and to mutations, which contributed to the maintenance of intra-population diversity.
In order to maintain the evolutionary potential within each population in the mid- to long-term, migration could be necessary to renew genetic variability or to counteract the accumulation of deleterious mutations (i.e. inbreeding depression and genetic load). Crossing experiments within and between the experimental DM populations showed that after 12 generations there was a significant inbreeding depression within populations but no genetic load [33]. Therefore, we concluded that no migration between populations was needed at this stage of divergence.
While experimental DM led to advances of knowledge in the field of evolutionary biology and conservation sciences [13,27,32,33,36,38,40] and proved to be very effective at conserving genetic diversity important for agronomic performance, the lack of political support for biodiversity educational activities and the effort of maintaining such a network led research stations and schools to gradually discontinue the experimental DM populations. The project started with an initial 12 sites in 1984, and increased to include 19 sites in 1996, but there remained only three research stations in 2010. As these stations usually consist of homogeneous agricultural systems with near-optimal environmental conditions, this drastic reduction in the number of sites with contrasting agro-climatic conditions questions the ability of the current experimental DM program to maintain efficiently wheat genetic diversity.
Another important lesson drawn from the experimental DM program is that when populations are only submitted to natural selection, this results in a release of selective pressures on important agronomic traits, as exemplified by the global increase of plant height and its consequences for lodging. Maintaining population with good agronomic value therefore requires the deployment of human selection for some key traits. On-farm DM with participatory breeding approach appears to be a more sustainable strategy in the management of agrobiodiversity because directly enrolled in a socio-economical framework, which is more realistic than an experimental framework.
3 On-farm dynamic management of agrobiodiversity
Although traditional farming systems in developing countries are known to maintain genetic diversity [41–43], similar systems in industrialized context have not yet been acknowledged. However, recently there has been increasing interest among low-input and organic farmers in on-farm conservation and breeding [44], because they face many challenges not present in conventional systems, including more heterogeneous environmental stress, the absence of adapted varieties [45], a lack of interest by public institutions and the commercial seed sector in producing varieties for these systems [46] and a restricted legislative framework [47,48]. While there has recently been interest in developing varieties for organic systems on the part of some public sector breeders and seed companies, such programs usually start with parents or breeding lines developed in conventional breeding programs, and released varieties must meet European catalog requirements for distinction, genetic uniformity and stability. This type of variety meets the needs of certain organic farmers but there are many others whose systems are too heterogeneous for this type of variety to be appropriate. Over 95% of crop varieties grown in organic systems come out of conventional breeding programs, even though traits required in organic systems may be quite different from those selected for under high-input conventional management [49].
To address this lack of suitable varieties, and because of their concern over the loss of crop biodiversity, networks of farmers have emerged in many European countries, including the Réseau Semences Paysannes (RSP) in France, the Red de Semillas in Spain, the Rete Semi Rurali in Italy [50], Arche Noah in Austria [51]. A recent European survey of these seed networks as part of the project Farm Seed Opportunities (FSO) identified 68 separate initiatives in 17 countries [52]. In many European countries, well-known landraces [53] or historic varieties [54] bring added value to producers, both in terms of lower input costs and higher market value because they are sought out by organic consumers. Organic farmers also observe that these historic or landrace varieties are more adapted to their systems of cultivation and that their heterogeneity provides buffering capacity against unpredictable environmental stress. Many farmers practice some type of selection, either to maintain desirable characteristics in these varieties, or to increase the adaptation and performance of these populations in their specific conditions.
3.1 On-farm dynamic management: farmers practices in on-farm conservation and participatory breeding programs in France
Farmers involved in on-farm DM practice a range of activities, from the maintenance of local varieties (on-farm conservation) to participatory plant breeding (on-farm PPB). In the following, we will give an overview based on a case study with the wheat group of the RSP in France. The central activity can be described as the maintenance of on-farm collections of different types of wheat varieties: Triticum aestivum and Triticum sp. relatives, landraces, traditional and historic varieties from the early period of plant breeding in the late 19th and early 20th centuries, more modern varieties, varietal mixtures…). Contrary to participatory varietal selection (PVS), which are programs in which farmers generally receive varieties from institutions [55], here farmers develop their own approach to find and to choose the germplasm they want to evaluate on their farm. This work allows farmers to observe a large range of wheat varieties under local farming conditions, increasing their knowledge of these varieties and helping them to make choices as to which varieties would be better adapted to their own environment, utilization and practices. They usually cultivate these collections in small plots (one to ten square meters). Based on their observations, they may decide to multiply some varieties in larger plots to increase seed stock before growing them in production fields.
While on-farm DM is not institution-led, farmers are highly aware of the risks that individual populations may be lost due to stochastic events or that varieties may cross-pollinate with each other and they take steps to prevent the loss of populations or undesirable cross-pollination. This approach includes the development of variety inventories and collective stocks so that each variety is maintained by multiple farmers to prevent its complete loss. Arche Noah, an Austrian NGO, conserves agricultural biodiversity in such a way [51]. One approach that appears to be very similar and successful is the development of community seed bank in several countries where traditional farming systems is still predominant. These initiatives were recently reviewed by Jarvis et al. [56]. Farmers also know each variety based on phenotypic characteristics and carefully cultivate each variety in reproductive isolation if necessary. For example, wheat plots are often 1 m apart to prevent harvesting heads of adjacent plots accidentally, and farmers remove any plants that do not belong to the particular variety. For inbred species, when cultivating mixtures in their production fields, farmers will usually maintain separate small plots of each component so that they can reconstitute their mixture if necessary, or experiment with different mixtures of their preferred varieties. These practices are possible because inbred species can be more easily separated into identified types or varieties. For open-pollinated crops such as maize, farmers in the network will divide up the varieties so that each farmer is responsible for one or two that they grow in isolated plots, often surrounded by trees or another buffer zone to protect the population from pollen of neighbouring hybrid maize fields. They conduct selection on plants and ears each year both to improve their populations and to remove chance contamination from other populations.
While most studies on on-farm conservation and breeding activities have been conducted in developing countries, in discussions among farmers from developed and developing countries, it is apparent that there are many opportunities for mutual learning. In particular, on-farm conservation methods used by European farmers have also been described in a traditional farming context, for example when farmers cultivate many varieties in a small collection or garden to evaluate and select varieties for their production fields [57]. In the RSP network, farmers often evaluate many varieties in small plots, and may conduct mass selection of new wheat types, leading to the creation of “modern landraces”. Based on their observations and their needs, they can also decide to make mixtures of different varieties (from just a few to several hundred varieties), as already described, to insure a sustainable productivity [58]. Some farmers experiment with planting certain wheat varieties in association with other species to increase the potential of the mixed culture (for instance with alfalfa). The most recent activity concerns the creation of new crosses between varieties previously observed on their farms. This initiative is specifically associated with a participatory research program described below. The involvement and expertise of RSP farmers in on-farm conservation and breeding is heterogeneous: each farmer decides on a strategy best adapted to his or her needs and environment. While some farmers only cultivate a specific mixture; others can maintain several hundred wheat varieties in on-farm living collections, and others test new populations and selections from the crosses described above.
3.2 On-farm conservation: the case study of the “Rouge de Bordeaux” revival in France
It is important to consider that these on-farm activities are strongly linked with seed diffusion between farmers but also with genebanks, in particular the French national genebank. Motivations leading farmers to exchange varieties are multiple but their main objective is to observe the behaviour of these varieties in several environments. Based on an ethno-botanic survey [54], it was shown that “Rouge de Bordeaux” (RdB), an historical population-variety derived from mass selection in the population-variety Noe, has been frequently grown and exchanged by the RSP farmers. Because RdB was selected and grown before the existence of the modern seed system linked with the homogenisation of varieties, this RdB variety is assumed to have historical level of diversity, which is probably not entirely conserved today. Relying on an interdisciplinary study between sociologists and geneticists, two independent dynamic processes of diffusion for this variety were detected: one within the informal sector as a direct historical diffusion among farmers; another from the formal sector with a sample sent by a breeding company to the national genebank around 1980s, which then provided some farmers with seed samples on request, primarily in the 1990s. A molecular study based on different populations of this RdB variety maintained by different farmers showed that differentiation between populations from the two identified groups is quite high (0.49 < Fst < 0.95), and that genetic diversity is greater in the group of populations derived from the informal sector (Nei index ∼0.3 compare to 0.03 for the formal component). These results illustrate that, up to now, the intra-varietal diversity of RdB has been poorly conserved in the ex situ collection. However, this study highlights the importance of considering the complementarity of ex situ and in situ conservation to better manage agrobiodiversity as already shown in a maize study [20,42]. For the RSP and farmers involved in the study, it was also the first time that their role in the management of crop diversity was recognized.
3.3 On-farm PPB: towards a better communication and material exchange between scientific institutions and farmers’ networks
Until recently, most participatory plant breeding programs were found in developing countries, and their relevance for developed agricultural systems was questioned. Due to the increasing interest in the conservation and use of genetic diversity among organic farmers on the one hand, and among scientists on the other, participatory research approaches have emerged as a relevant and necessary response to the problem of conserving genetic diversity in industrialized countries. At the beginning of some of these participatory research and breeding projects there were tensions among scientists, who need to work with measurable, analysable criteria and farmers, who have a more holistic approach. This does not, however, mean that scientists do not appreciate the knowledge and skills of farmers and vice-versa. Specifically for the participatory wheat-breeding project in France, discussions between Inra scientists and the Réseau Semences Paysannes in the course of on-farm research projects have led participants to a better understanding of each others’ points of view. For more details on the particular research project, the Farm Seed Opportunities European project, see http://www.farmseed.net/home/.
Farmers initially interested primarily in the conservation of landraces without modification have become interested in selection and have made crosses among landraces with the support of scientists. Scientists have recognized the value of historic and landrace varieties and the benefits that their cultivation brings to farmers. When developing the on-farm participatory breeding program, scientists and farmers sought to develop experimental designs and evaluation procedures that correspond to the constraints and needs of farmers as well as the scientists’ needs for precision for a quantitative analysis. Farmers and scientists discuss selection strategies as well as genetic diversity management, and decisions are made together, in the sense of a genuine collaboration. As with the experimental DM populations, phenotypic and genetic measures can be used to monitor the evolution of genetic diversity and the adaptation of varieties developed and/or conserved on-farm. The objective is to provide the farmers’ network with as much information and analysis as they need to evaluate their conservation and selection activities, and to describe the evolution of diversity within these populations. The quantitative data and analysis from on-farm projects complements farmers’ selection, and increases basic scientific knowledge about adaptation and evolution in diverse populations. Both farmers and researchers within this project are concerned with the potential loss of diversity within wheat varieties and the collaboration presents a unique opportunity to understand how in situ networks can best conserve genetic diversity of interest to farmers.
4 Conclusion: a general scheme for crop biodiversity management
We have shown in Section 2 that the ability of the current experimental DM program to efficiently maintain wheat genetic diversity depends on ensuring a sufficient number of sites with contrasting agro-climatic conditions. Thus, such an experimental DM program would require the constant support of institutions to provide an effective contribution to diversity conservation. With appropriate support, experimental DM would constitute a good complement to an on-farm DM approach, making it possible to develop resources adapted to various climatic conditions under homogeneous agricultural systems with near-optimal environmental conditions. By comparison, as on-farm DM is directly enrolled in a socio-economical framework, it allows for the maintenance of populations with good agronomic value by the deployment of human selection for some key traits.
As illustrated in Section 3, our study of RdB variety demonstrated that the diversity (assessed both at phenotypic and molecular levels) conserved on-farm is not a duplicate of that conserved in the genebank: it is both quantitatively and qualitatively different [54]. The amount of diversity present in most on-farm populations was greater than that of the genebank accessions, and the alleles present in the on-farm network were different than those present in the genebank accessions. In addition, results from the FSO study on landrace diversity and adaptation showed that many landraces were of agricultural interest outside their region of origin and that they remained phenotypically distinct even with a high level of phenotypic diversity and changes in the phenotypic response over time when cultivated on-farm under diverse environmental conditions (FSO report, [59]). Both the results on RdB and those on landraces show that historic varieties and landraces cultivated and exchanged among farmers can behave as metapopulations, similar to the experimental DM network of populations in terms of the conservation and creation of genetic diversity. In addition, although on-farm DM is much less controlled than ex situ conservation, we have shown that farmers take steps to prevent the loss of populations or undesirable cross-pollination. This is consistent with conclusions drawn from many studies showing that farmers’ practices in traditional agricultural systems in developing countries maintains genetic diversity while allowing for local adaptation of populations due to the evolutionary processes within and among crop populations [41–43,57,60].
The results of our work on experimental and on-farm DM led us to conclude that it is important to encourage a broad network of on-farm DM activities to complement the genebank activities. The current regulatory situation, however, restricts on-farm conservation and especially the exchange of landraces and historic varieties among farmers. Varieties must be registered in the official catalog to be exchanged, and the registration criteria exclude heterogeneous populations such as landraces. Recent European legislation on conservation varieties is a step in the right direction, as it allows certain landraces to be cultivated in their region of origin, but still imposes certain limits on the allowable heterogeneity of these varieties, and prohibits exchanges and production of seed outside of the region of origin. There is no allowance for farmer-developed varieties and populations that are not recognized as landraces, so most of the populations used in on-farm DM have no regulatory framework [59].
Limiting the legal framework for on-farm conservation to certain landraces cultivated in a narrowly defined region of origin is neither necessary nor desirable. The history of the development of landraces is one of human migration, selection and re-adaptation, and the current and future viability of crop species will depend on preserving this adaptive capacity. Farmers’ role in the maintenance and enhancement of agrobiodiversity needs to be recognized in order to conserve the relevant genetic resources to respond to changing climate conditions.
The encouragement of such networks of conservation and exchange does not need to be in opposition to conservation in ex situ collections. These two methods are complementary in terms of their goals, strengths and weaknesses as mentioned in the fourth technical conference paper on plant genetic resources in 1996 [61]. Genebanks have appropriate facilities for long-term conservation of a great number of accessions, while farmer networks can conserve populations in their evolutionary context, to maintain and enhance the genetic diversity we will need to respond to future environmental challenges [20,62–64]. The best way to prevent genetic erosion is to maintain public collections in genebanks that are accessible to the farming community, to expand the cultivation and selection of diverse populations in a wide range of environmental conditions, and to maintain a robust network of many sub-populations cultivated in on-farm condition and exchanged among farmers, with reciprocal exchanges between in situ and ex situ collections.
In conclusion, DM is one of the strategic options available to manage crop biodiversity, and on-farm DM provides a unique solution for the development of varieties locally adapted to marginal areas and cropping systems, or varieties used for small specific markets, where seed companies cannot make any profit with regard to the needed investments. The fact that selection is non-centralized and multi-actor maximizes the maintenance and creation of variability. The existence of this participatory approach to selection therefore does not threaten the domain of private seed companies, which, often with their own stock of genetic resources, can continue to select homogeneous cultivars for high-input agricultural systems. Genebanks have the charge of both safeguarding representative samples of the genetic diversity of a species, and providing users access to genetic resources. Different exchanges can exist and are necessary between the three entities: private and public sector breeders and farmer-breeders can use genebanks as a source of genetic variation, while genebanks can in turn store their new cultivars/populations (Fig. 5).
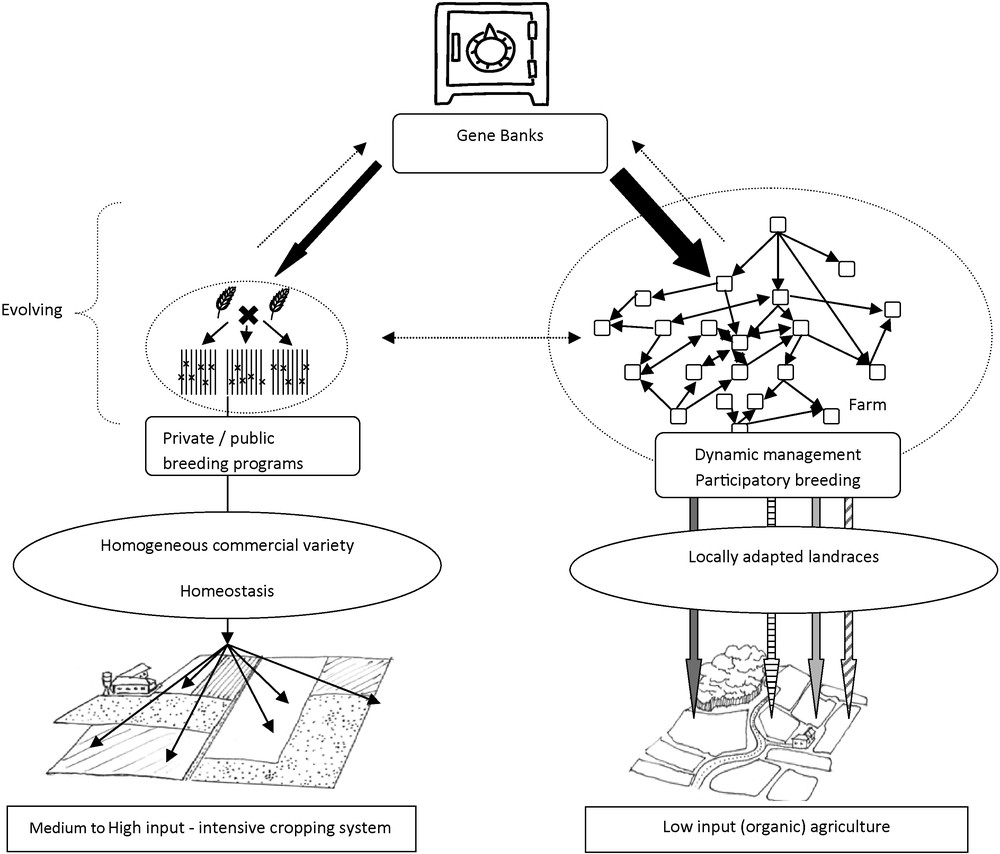
Schematic representation of the links between the three components managing plant genetic resources: genebanks, public and private breeding Programs and dynamic management/participatory breeding initiatives.
Another important feature of these three components of the use of genetic resources is that they rely on three different organizational systems, which reinforces their complementarities. Genebanks are centralized structures mainly based on public or state structures, seed companies rely on a market system, with usually few actors, and participatory breeding is a non profit activity realized in a decentralized network of farmers. This diversity of structures and organizations therefore reduces the risks of simultaneous loss of genetics resources from all three components of the system.
DM is therefore an important strategy for the management of genetic resources, giving back to farmers a role in the evolution of the cultivated species. If spontaneous initiatives have already started in France, a more extended and significant development of DM in industrialized agriculture will necessitate a better integration of this activity in the formal seed system, with a real consideration of its long-term interest for agriculture.
Disclosure of interest
The authors declare that they have no conflicts of interest concerning this article.