1 Introduction
Clestobothrium Lühe, 1899 comprises five species, C. crassiceps, the types-species, C. neglectum, C. gibsoni, C. splendidum and C. cristinae. Formerly in the suppressed order “Pseudophyllidea”, Clestobothrium was transferred to the newly erected order Bothriocephalidea [1,2]. Morphological, molecular and ecological data showed that the order “Pseudophyllidea” consisted of two unrelated clades [1,2]. The members of the Bothriocephalidea are parasites of teleost fishes and comprise 46 genera distributed into four families, the Bothriocephalidae, the Echinophallidae, the Philobythiidae, and the Triaenophoridae [2]. Clestobothrium is a member of the family Bothriocephalidae, which includes seven other genera.
Numerous transmission electron microscope (TEM) studies have been published on the ultrastructure of cestode embryonic development, the important role of vitellocytes and the nourishment of cestode embryos, as well as on the great diversity of mature tapeworm eggs [3–15]. As far as we are aware, there have been TEM studies of the embryonic development and eggs of only four bothriocephalidean species, i.e. Bothriocephalus clavibothrium [5,12], B. gregarious and B. barbatus [16], and Eubothrium salvelini [8,17].
The aims of the present study are to describe the functional ultrastructure of the eggs, the associated vitellogenesis and the early intrauterine embryonic development of the bothriocephalidean cestode C. crassiceps (Rudolphi, 1819), to compare the results with those of similar studies on other lower cestode taxa, and in particular bothriocephalideans, and to consider any possible phylogenetic implications.
2 Materials and methods
Live adult specimens of C. crassiceps were collected from the intestine of the hake Merluccius merluccius (L., 1758) (Gadiformes: Merlucciidae) caught off Roses, Girona, Spain.
2.1 High-pressure freezing
The live cestodes were examined under a stereomicroscope and pieces of uterus were excised into small Petri dishes in PBS with 20% BSA and transferred into the cavity of a 200-μm-deep flat specimen carrier. The specimen holder was then inserted into the rapid transfer system, and high pressure frozen using a Leica EM PACT and stored in liquid nitrogen.
2.2 Freeze substitution and infiltration with resin
For freeze substitution, sample holders were transferred into pre-cooled cryovials (−120 °C) and freeze substitution was performed in anhydrous acetone containing 2% of osmium tetroxide. Using a Leica EM AFS, the samples were maintained for 24 h at −90 °C. Hereafter, the temperature was raised at a rate of 2 °C/h to −60 °C and then to −30 °C. The samples were maintained at each level for 9 h in the original substitution medium. Specimens were then washed three times for 10 min in fresh anhydrous acetone. After washing, the temperature was gradually raised to room temperature and the specimens were infiltrated with Spurr resin (one part resin/three parts acetone) overnight; followed by 1:1 for 4 h; 3:1 for 4 h and 100% resin for 4 h and then overnight. Polymerization was carried out using heat at 60 °C for 72 h. Ultra-thin sections were cut using a Reichert-Jung Ultracut E ultramicrotome, placed on copper grids and post-stained with uranyl acetate (2%) in methanol for 5 min and lead citrate for 4 min. Finally, ultra-thin sections were examined using a JEOL 1010 TEM operated at an accelerating voltage of 80 kV.
The terminology of cestode eggs and embryonic envelopes in different developmental stages is after Conn and Świderski [18].
3 Results
3.1 General egg topography
In C. crassiceps, the uterine coils are median and terminate in a large uterine sac; the uterine pore is medioventral. Intrauterine and released eggs are smooth, relatively thick-shelled and operculate. The total number of blastomeres in these early embryos, enclosed within electron-dense eggshells, can be up to ∼ 20 and of various sizes and ultrastructural characteristics. Mitotic division of early blastomeres was frequently observed at both LM and TEM levels. Intrauterine eggs contain numerous vitellocytes at the egg periphery, just beneath the eggshell, with the early embryos generally being localized in the central parts of the egg (Fig. 1A,B). A well-defined operculum was observed in both semi-thin sections, by LM (Fig. 1A), and in ultra-thin sections (Fig. 2B), using TEM. Numerous vitellocytes were in the process of a progressive fusion, thus, forming a vitelline syncytium (Figs. 2A,B and 3A). However, many of them were still surrounded by their individual plasma membrane and contained a degenerating nucleus and very high accumulation of large lipid droplets embedded in an amorphous, agranular cytoplasm of very low electron-density. The degenerating lipid droplets (Figs. 2A,B and 3A) generally exhibit all levels of gradation in their electron-density, reflecting very different degrees of chemical saturation, i.e. from very osmiophilic, and thus black in the TEM micrographs, to very osmiophobic, and showing as white. As seen on Figs. 2A,B and 3A, important differences occur between the cytoplasm of the blastomeres and that of the surrounding vitellocytes. The blastomeres of the early embryos are characterized by a highly granular cytoplasm rich in free ribosomes and several small mitochondria; their nuclei contain prominent electron-dense nucleoli and numerous heterochromatin islands randomly dispersed in the karyoplasm.
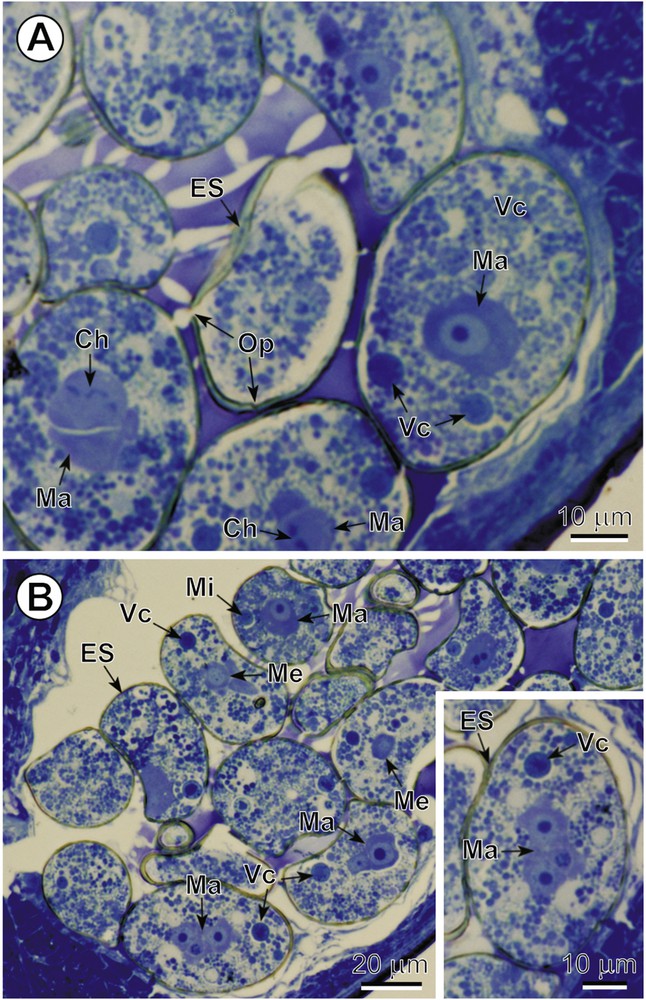
A–B. General topography of the intrauterine eggs. Two LM micrographs and inset of semi-thin sections illustrating the cellular composition and general topography of intrauterine eggs. Note: (1) the numerous vitelline cells (Vc) and several early blastomeres of the three different types, macro- (Ma), meso- (Me) and micromeres (Mi), of various sizes and cellular characteristic; (2) the mitotic cleavage divisions visible in some blastomeres; and (3) the readily visible operculum (Op) in the eggshell (ES) situated in the centre of Fig. 1A. Ch: chromosomes. Colour available on the web. Masquer
A–B. General topography of the intrauterine eggs. Two LM micrographs and inset of semi-thin sections illustrating the cellular composition and general topography of intrauterine eggs. Note: (1) the numerous vitelline cells (Vc) and several early blastomeres of the three different ... Lire la suite

A–B. Low-power ultrastructure of the intrauterine egg. Note: (1) that Fig. 2A–B represents two low-power TEM micrographs showing two opposite poles of the same egg composed of numerous vitellocytes (Vc) and the two blastomeres of the macromere type (Ma); (2) compare important differences that occur between the cytoplasm of these blastomeres and the cytoplasm of surrounding vitellocytes; (3) note that the blastomeres are characterized by a highly granular cytoplasm rich in free ribosomes and several small mitochondria (m); their nuclei (N) contain prominent electron-dense nucleoli (n) and numerous heterochromatin islands randomly dispersed in the karyoplasms; (4) note the numerous degenerating vitellocytes at different stages of apoptosis that exhibit a very electron-lucent cytoplasm containing their nuclei and a high accumulation of lipid droplets (L), both undergoing various phases of autolysis. AF: artefact of fixation; ES: eggshell; Op: operculum. Masquer
A–B. Low-power ultrastructure of the intrauterine egg. Note: (1) that Fig. 2A–B represents two low-power TEM micrographs showing two opposite poles of the same egg composed of numerous vitellocytes (Vc) and the two blastomeres of the macromere type (Ma); ... Lire la suite
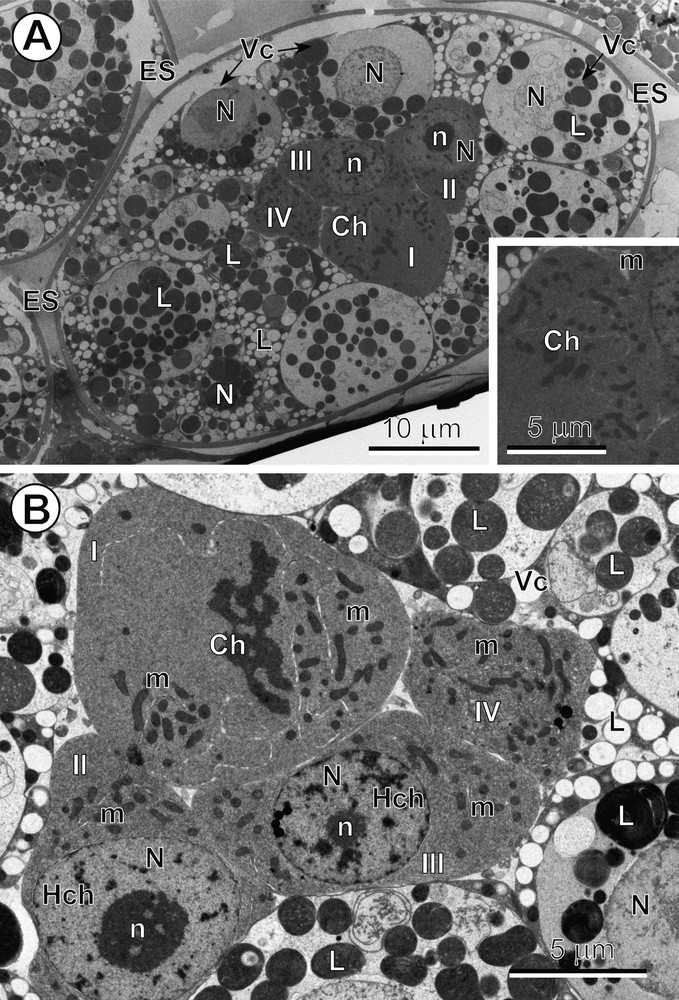
A–B. Mitotic cleavage divisions of blastomeres. (A) Entire egg composed of numerous vitellocytes (Vc) and four blastomeres (I to IV), three of which represent typical macromeres and one of which is undergoing a mitotic cleavage division in metaphase. Inset: see enlarged detail of the mitotic cleavage division at metaphase. (B) Central part of the egg showing an early embryo composed of four blastomeres, two of which are typical macromeres at interphase, each one with a large, characteristic nucleus (N) containing a prominent nucleolus (n) and the numerous heterochromatin islands (Hch), and another macromere of a larger size, showing a mitotic cleavage division at early anaphase. Ch: chromosomes; ES: eggshell; L: lipid droplets; m: mitochondria. Masquer
A–B. Mitotic cleavage divisions of blastomeres. (A) Entire egg composed of numerous vitellocytes (Vc) and four blastomeres (I to IV), three of which represent typical macromeres and one of which is undergoing a mitotic cleavage division in metaphase. Inset: see ... Lire la suite
3.2 Vitellocytes
A progressive degeneration of the vitellocytes, which begins immediately following egg formation, is accompanied at a later stage by a progressive degeneration of the micromeres within the egg. Such forms of apoptosis likely provide nutritive substances for the developing embryo. The numerous degenerating vitellocytes, at different stages of apoptosis, always exhibit a very translucent, rather electron-lucent cytoplasm containing degenerating nuclei and a high accumulation of lipid droplets undergoing various degrees of autolysis.
3.3 Blastomere fine structure and change
As indicated above, the total number of blastomeres in the early embryos, enclosed within the electron-dense eggshells, can be as many as ∼ 20 and these may vary in size and ultrastructural characteristics. Mitotic division of early blastomeres was frequently observed at both LM and TEM levels. Early intrauterine embryos are composed of three types of blastomeres, i.e. micromeres, mesomeres and macromeres (Figs.1A,B, 2A,B, 3A,B and 4A,B).
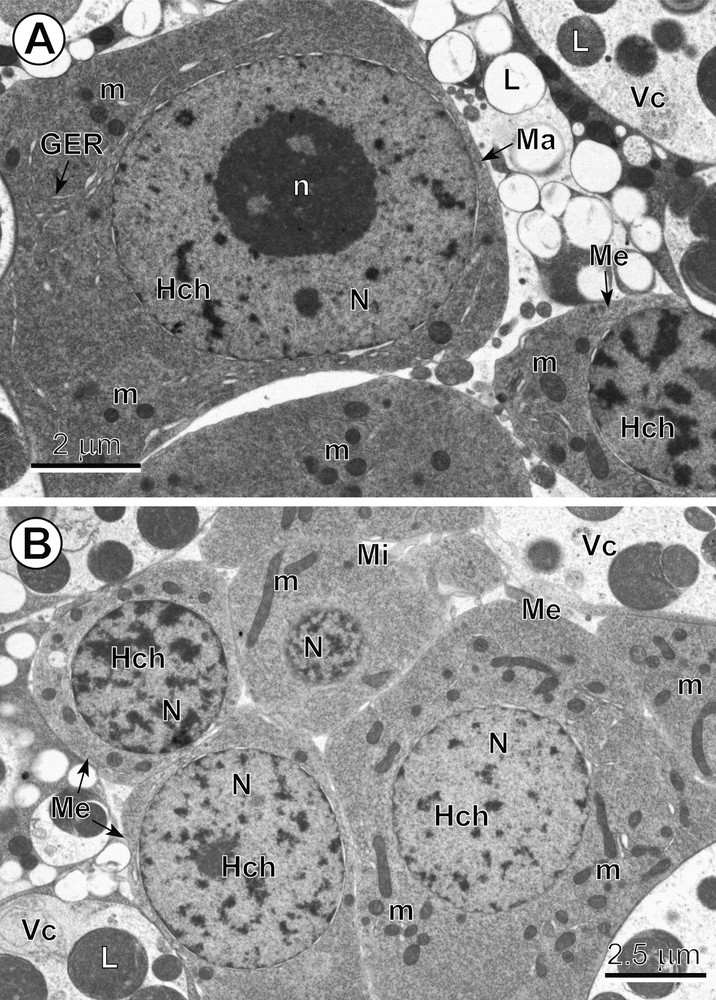
A–B. Comparative ultrastructure of three types of blastomeres. (A) Note: (1) a typical macromere (Ma) at interphase, with a large, characteristic nucleus (N) containing a prominent nucleolus (n) and numerous heterochromatin islands (Hch), surrounded by a relatively thin layer of highly granular cytoplasm rich in free ribosomes, with a few short profiles of granular endoplasmic reticulum (GER) and several small mitochondria (m). (B) Central part of the egg with an early embryo composed of six blastomeres, three of which have very numerous randomly dispersed heterochromatin islands in their nuclei, which represent typical mesomeres (Me), and the smallest blastomere, situated in the upper part of the micrograph, which represents an early micromere (Mi); Vc: vitellocytes. Masquer
A–B. Comparative ultrastructure of three types of blastomeres. (A) Note: (1) a typical macromere (Ma) at interphase, with a large, characteristic nucleus (N) containing a prominent nucleolus (n) and numerous heterochromatin islands (Hch), surrounded by a relatively thin layer of ... Lire la suite
Micromeres are characterized not only by their small size, but also by their spherical nuclei, which contain numerous small islands of highly condensed heterochromatin randomly dispersed in their electron-lucent nucleoplasm (Fig. 4B). Their granular cytoplasm, which is rich in free ribosomes, includes several elongate mitochondria (Fig. 4B). Some micromeres were observed undergoing a rapid apoptosis and were visible as dense pycnotic nuclei when observed in semi-thin sections (Fig. 1A,B).
Medium-sized blastomeres, i.e. mesomeres, contain spherical nuclei with numerous heterochromatin islands, which are sometimes adjacent to the nuclear envelope but also randomly dispersed in the nucleoplasm (Fig. 4B). Their nuclei are embedded in a granular cytoplasm along with numerous elongate mitochondria of various sizes (Fig. 4A,B).
The two macromeres were the largest blastomeres (Figs. 2A,B, 3A,B and 4A). They contain prominent nuclei with spherical nucleoli and numerous small heterochromatin islands more or less randomly dispersed in a moderately electron-dense nucleoplasm (Figs. 2A,B and 4A). Their granular cytoplasm is rich in free ribosomes and exhibited only a few small mitochondria and several short profiles of granular endoplasmic reticulum (GER) (Fig. 4A).
Typical mitotic division of the blastomeres was observed in intrauterine eggs in both semi-thin (Fig. 1A,B) and ultra-thin sections (Fig. 3A,B). Different phases of mitotic cleavage division were most frequently observed in typical macromeres (Fig. 3A,B).
4 Discussion
The present study shows clearly that the bothriocephalid cestode C. crassiceps produces polylecithal eggs, which at the intrauterine stage, are operculate and exhibit various stages of early embryonation, i.e. this species appears ovoviviparous.
4.1 The operculum and embryonation
There are conflicting reports concerning the presence or absence of an operculum in the eggs of the species of Clestobothrium. According to the literature, an operculum is listed as absent in the generic diagnoses [19–22], an observation probably based on the description of the type species, C. crassiceps, by Cooper [23]. This fact was reflected by Tadros [24,see p. 87], who amended the diagnosis of this genus to include only species with anoperculate eggs. With regard to other species, an operculum was described in C. neglectum as “not seen” [25], present in C. gibsoni [26], and present but inconspicuous in C. splendidum and C. cristinae [27]. According to the generic diagnosis in the chapter of Bray et al. [22] in the CABI Keys to the cestode parasites of vertebrates, the eggs are not only anoperculate but also unembryonated. While there are different opinions concerning the presence or absence of an operculum among bothriocephalidean species, there is frequent agreement that their freshly released eggs are usually unembryonated, as described for C. splendidum and C. cristinae [27]. However, a clear exception to this is C. gibsoni, where the eggs are fully developed in the distal uterus [26]. Furthermore, in view of our observations above and the recent redescription of C. crassiceps [28], where operculate eggs were found, it is clear that the generic diagnosis of Clestobothrium, such as that by Bray et al. [22] needs to be amended to include species with either anoperculate or operculate and oviparous or ovoviparous eggs. This is, however, complicated by the fact that Azzouz Draoui and Maamouri [28] claimed to have observed that freshly laid eggs of C. crassiceps are initially anoperculate and unembryonated, when released into the external aquatic environment, contradicting our observations. They further claim that the operculum appears only after 11–13 days of development in water, and only about three days before the hatching of the coracidial larva. In the present study, we have demonstrated that the intrauterine eggs of C. crassiceps are operculate and clearly exhibit various stages of early embryonation, i.e. ovoviviparity.
4.2 Vitellogenesis
One of the aims of the present study was not only to describe the ultrastructure of the intrauterine eggs of C. crassiceps, but also to relate this to the vitellogenesis of this parasite [29]. Vitellocytes in cestodes have two important functions, i.e. eggshell formation and the nourishment of the early embryo [13,14,30]. During cestode evolution, either one of these two functions have been intensified or much reduced in different taxa, depending on their embryonic development, degree of ovoviviparity and life cycle [11,13,14,31–35]. In the polylecithal eggs of C. crassiceps, which contain ∼ 30 vitelline cells, both functions of the vitellocytes appear much intensified. In our previous study on vitellogenesis in this species, cytochemical staining with periodic acid-thiocarbazide-silver proteinate for glycogen indicated a strongly positive reaction for β-glycogen particles and α-glycogen rosettes, which formed several large glycogen accumulations around the large, saturated lipid droplets of maturing and mature vitellocytes [29]. It seems rather surprising that no trace of this glycogen was observed in the eggs or eggshell-enclosed vitellocytes, which contain only a very high accumulation of large lipid droplets exhibiting different degrees of chemical saturation. The only possible explanation for this is a very rapid utilization of this glycogen in the earliest stages of egg formation. However, the heavy accumulation of lipid droplets in the vitellocytes of intrauterine eggs may represent important nutritive reserves for the developing embryo [36]. These droplets are generally considered as important energy reserves, although this may not always be the case in cestodes [37]. Indeed, two hypotheses prevail, i.e. they represent (1) an energy source or (2) the waste products of metabolism [29]. Ultrastructural studies on the coracidial larva of B. clavibothrium showed that they functioned as important energy reserves [12]. A marked decrease in the amounts of lipids was observed also in the coracidia of T. nodulosus after prolonged swimming [38,39], which was confirmed by similar observation of a marked decrease in the number of lipid droplets in the ciliated envelopes of coracidia of T. nodulosus, after three days of active swimming [40]. According to these authors, the large lipid droplets in the ciliated envelopes of coracidia are phospholipids [39,40]. Moczoń [41], using the cysticercoid metacestode of the cyclophyllidean Hymenolepis diminuta, endorsed opinion on the important role of lipids in cestode morphogenesis. The latter author stated that: “the utilization of neutral lipids proves both the presence of a lipase-type enzyme(s) and of an operative β-oxidation pathway in the cells of the cysticercoids, the latter feature being highly indicative of oxidative metabolism of these larvae.” The very heavy accumulations of large lipid droplets, which are very abundant in the intrauterine eggs of C. crassiceps, may be associated with the physical and physiological adaptations that enhance the parasite's transmission to the next host. Until we have more information on the cestode life cycles and both the variety of egg types and their adaptations to different hosts and environments, it will be difficult to judge to what extent the ultrastructure of the vitellocytes, and particularly, those with a large accumulations of lipids, is a reflection of host influence, ecological adaptation or phylogenetic relationships.
Variations in vitellogenesis and egg development in the various cestode groups have been reviewed by Świderski and Xylander [14]. However, studies on most groups are still too few to make any definite judgement in terms of their phylogenetic implications. This is especially true in the cases of apparently distinctive features, such as the absence of lipids in mature vitellocytes of the majority of caryophyllidean species [11,42–44]. It seems more likely that vitellocyte development and composition reflects the subsequent nature of the life history of the worm and the length of time that the egg/larva must remain viable outside of the definitive host.
4.3 Oviparity and ovoviviparity
Closer study of the developmental state of intrauterine eggs has shown that embryogenesis may begin before the eggs are shed into the environment, thus, altering our classification of the type of birth evident in some cestodes. For example, the caryophyllidean Khawia sinensis has been characterized, on the basis of LM studies, to be oviparous, i.e. having unembryonated eggs [45], whereas the caryophyllidean Wenyonia virilis, similarly as Archigetes appendiculatus [46], was originally described as having embryonation occurring in intrauterine eggs [47]. This statement, based on LM observations, was subsequently verified with TEM studies on the same species [9,10]. Nevertheless, recent TEM studies on K. sinensis [15] have conclusively demonstrated that this species is in fact also ovoviviparous, since, as in C. crassiceps, there is intrauterine embryogenesis.
4.4 Apoptosis
The degeneration of eggshell-enclosed vitelline cells and several pycnotic micromeres in cestodes represents a good example of apoptosis, or programmed cell death. With respect to vitellocytes, once their two important functions, i.e. eggshell formation and the storage of nutritive reserves for the developing embryo, are completed, they undergo a progressive degeneration and autolysis, which involves their cell organelles and inclusions. With regard to their residual lipid droplets, they exhibit different degrees of chemical saturation as they become reabsorbed by the differentiating embryos [48]. Apoptosis of both vitellocytes and some of the micromeres also takes place during both early and more advanced stages of embryonic development and blastomere differentiation. As reported by Rybicka [49,50] and Świderski [51,52], the degeneration of numerous micromeres results in a great reduction in the numbers of oncospheral cells, a common feature for both lower [5] and higher cestodes [4,51,52].
4.5 Egg developmental types and their phylogenetic implications
Can one ascribe any phylogenetic significance to the different types of egg development? Two types of eggs have been described for bothriocephalidean cestodes:
However, as we learn more about bothriocephalidean egg types, as exemplified by the species of Clestobothrium discussed in this paper, it is likely that a greater variety of egg types will be found in this order. Cestode eggs may exhibit various morphological adaptations that aid transmission to their various intermediate hosts in different aquatic environments [4,6,53]. Furthermore, there are many factors that influence and promote a successful cestode life cycle [54]. Because of the complexity in terms of important and overlapping factors, such as the morphological adaptation of eggs in relation to the nature of parasite's intermediate host(s), the effect of different aquatic environments on the life cycles or variations in life cycles involving different hosts, it appears nigh impossible to distinguish adaptive factors from those that may purely reflect phylogenetic affinities.
Disclosure of interest
The authors declare that they have no conflicts of interest concerning this article.
Acknowledgements
We are grateful to Professor John S. Mackiewicz, State University of New York at Albany, USA and Dr David I. Gibson, Department of Zoology, Natural History Museum, London, UK for kindly commenting on an earlier version of the manuscript. We wish also to express our thanks to the “Unitat de Microscòpia, Facultat de Medicina, Centres Científics i Tecnològics de la Universitat de Barcelona (CCiTUB)”, for their support in the preparation of samples, and particularly to Núria Cortadellas and Almudena García. The present study was partly funded by the Spanish project CTM2009-08602.