1 Introduction
The order Trypanorhyncha Diesing, 1863 is a large group of common marine polyzoic cestode parasites, the unique feature of which is the presence of a rhyncheal apparatus and whose adult stages are typically found in the stomach and spiral valve of elasmobranch fishes (sharks and rays); their larval forms infect a wide variety of marine invertebrates and fishes [1]. In trypanorhynchs, the attachment organ is a scolex which bears two or four bothria [2] and a rhyncheal apparatus consisting of four retractile tentacles armed with a complex array of hooks, which are linked via tentacle sheaths to four bulbs [3]. The rhyncheal apparatus forms a robust synapomorphy that supports the monophyly of this order. The Aporhynchidae Poche, 1926 is unique in the order in that species of its only genus, Aporhynchus Nybelin, 1918, have secondarily lost their rhyncheal apparatus [4,5].
The two important functions of cestode vitellocytes, eggshell formation and nourishment of the embryo [6], can be intensified or reduced, depending on the characteristics of each species in terms of its degree of ovoviviparity and life-cycle. Several TEM studies are available on the structure and differentiation of vitellocytes in the Cestoda [7]. Among the trypanorhynch cestodes, only four species have been examined in this respect: Grillotia erinaceus (Lacistorhynchidae), Dollfusiella spinulifera, Parachristianella trygonis (Eutetrarhynchidae) and Progrillotia pastinacae (Progrillotiidae) [8–11].
Using a member of a previously unstudied group, i.e. the aporhynchid Aporhynchus menezesi Noever, Caira, Kuchta and Desjardins, 2010, a parasite of the lanternshark Etmopterus spinax, the aims of the present study are:
- • to describe the ultrastructural and cytochemical aspects of vitellogenesis and the functional ultrastructure of mature vitellocytes;
- • to compare the results with those of previous reports on the four trypanorhynch species examined previously;
- • to discuss any possible functional, developmental, life-cycle or phylogenetic implications of the results obtained.
2 Materials and methods
Live adult specimens of A. menezesi were collected by Prof. Janine Caira and her co-workers from the spiral valve of a naturally infected velvet belly lanternshark E. spinax (L.) (Elasmobranchii: Etmopteridae) captured off Faial Island (38°31′N, 28°37′W) (Azores Archipelago, Portugal).
Live cestodes were first placed in a 0.9% NaCl solution and then mature proglottids were fixed in cold (4 °C) 2.5% glutaraldehyde in a 0.1 M sodium cacodylate buffer at pH 7.4 for a minimum of 2 h, rinsed in a 0.1 M sodium cacodylate buffer at pH 7.4, postfixed in cold (4 °C) 1% osmium tetroxide with K4FeCn6 in the same buffer for 1 h, rinsed in a 0.1 M sodium cacodylate buffer at pH 7.4, dehydrated in an ethanol series and propylene oxide, and finally embedded in Spurr's resin. Ultrathin sections were obtained using a Reichert-Jung Ultracut E ultramicrotome, placed on copper grids and double-stained with uranyl acetate and lead citrate. Ultrathin sections were examined using a JEOL 1010 TEM operated at an accelerating voltage of 80 kV.
The Thiéry technique [12] was used for highlighting the presence of glycogen particles. Gold grids were treated in periodic acid, thiocarbohydrazide and silver proteinate (PA-TCH-SP) as follows: 30 min in 10% PA, rinsed in milliQ water, 24 h in TCH, rinsed in acetic solutions and milliQ water, 30 min in 1% SP in the dark, and rinsed in milliQ water.
3 Results
3.1 General topography of the vitellarium
In the trypanorhynch cestode A. menezesi, the follicular vitellarium is composed of oval follicles, which are circum-medullary and extend almost the entire length of the proglottid but are less dense in the region of the uterus and ovary. Each vitelline follicle consists of cells in various stages of development, from immature cells of the gonial type close to the periphery to mature cells towards the centre of the follicle. Although vitellocyte cytodifferentiation represents a continuous process, in order to facilitate its description, it was subdivided into four discrete stages. These stages are:
- • immature;
- • early differentiation;
- • advanced maturation;
- • mature.
In fact, the gradual and continuous changes involved in this process occur clearly within each of the stages delineated herein (compare, for example, Fig. 1A and B, illustrating changes in the volume of the cytoplasmic layer and the number of its cell organelles which are already present at the gonial stem cell stage [I] and indicate the very beginning of the early maturation stage [II]). The interstitial cells were not examined in detail, but their elongate cytoplasmic processes surround the periphery of the vitelline follicles and penetrate deeply between the differentiating vitellocytes (Figs. 1B and 3B). The long, ramified cytoplasmic processes of interstitial cells of A. menezesi (Figs. 1B and 3B) contain heavy accumulations of glycogen particles and several lipid droplets and mitochondria.
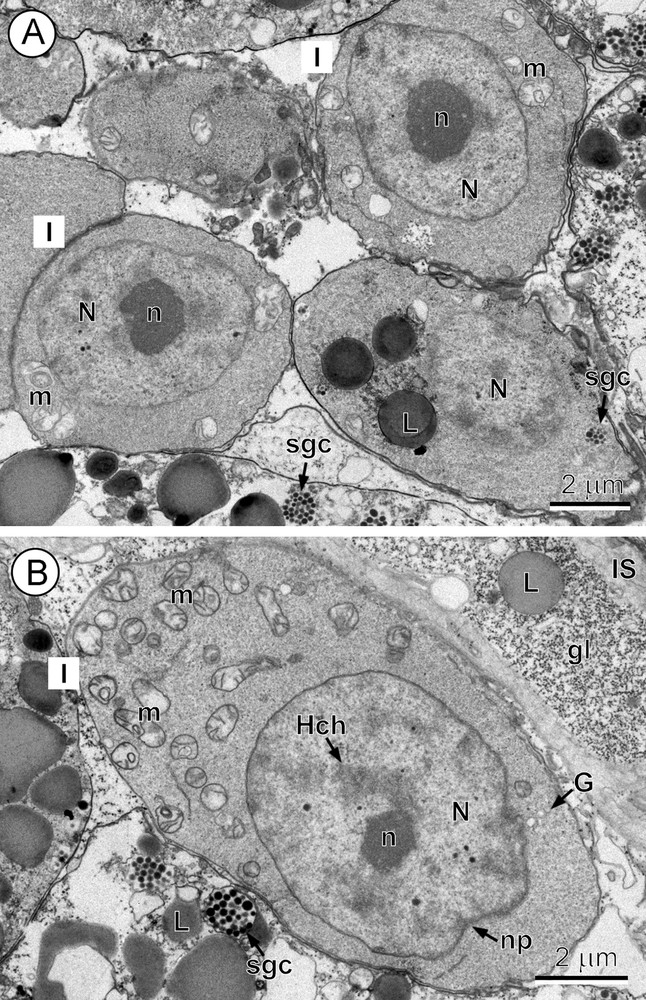
A-B. Undifferentiated stem cells of the gonial type or stage I. A. Undifferentiated stem cells of the gonial type (stage I) situated at the periphery of the vitelline follicles. Note: (1) the high nucleo-cytoplasmic ratio of these precursor cells with a large nucleus containing a prominent, electron-dense nucleolus (n); and (2) a thin layer of granular cytoplasm surrounding the nucleus (N), which contains a very few small mitochondria (m). L: lipid droplets; sgc: shell-globule clusters. B. A distinct increase in cell size observed at the end of stage 1 (compare with Fig. 1A). Note: (1) a much larger volume of cytoplasm containing numerous mitochondria (m), a few short profiles of GER and Golgi complexes (G); and (2) a large nucleus (N) with an electron-dense nucleolus (n) and several islands of heterochromatin (Hch). gl: glycogen; IS: interstitial syncytium; L: lipid droplet; np: nuclear pore; sgc: shell-globule cluster.
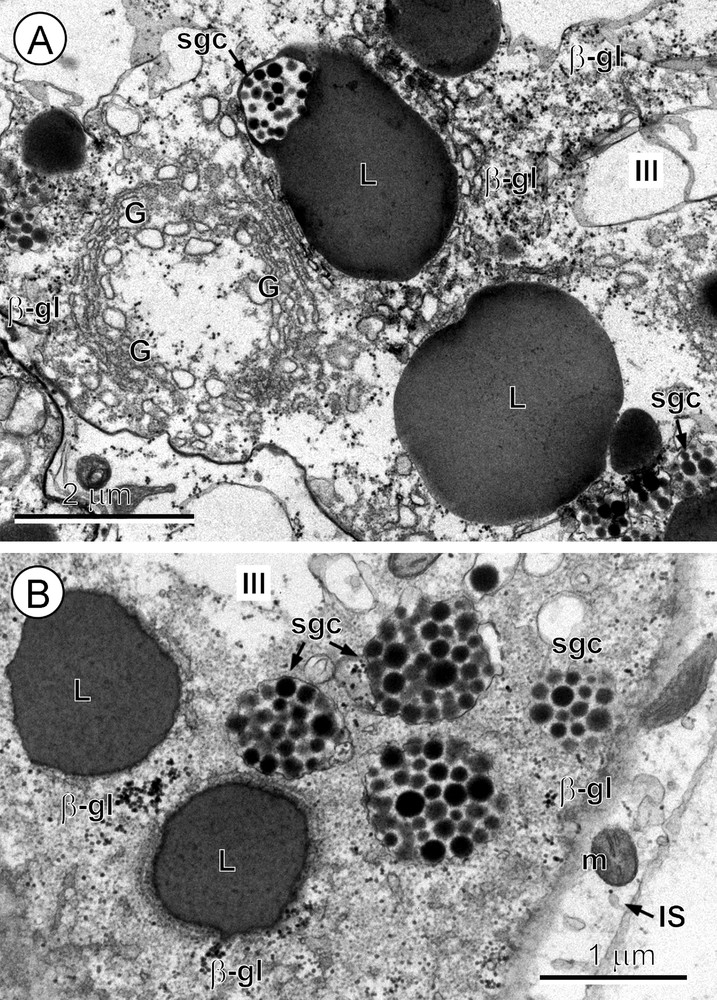
A-B. Advanced maturation of the vitellocyte or stage III. A. The peripheral region of the vitellocyte cytoplasm showing concentrically arranged Golgi complexes (G) composed of numerous vesicles at various degrees of dilation; adjacent to them are shell-globule clusters (sgc), large lipid droplets (L), one with a closely adjacent shell-globule cluster, and several β-glycogen particles (β-gl) in the upper right corner of the micrograph. B. Details of the vitellocyte cytoplasm (stage III). Note several shell-globule clusters (sgc), lipid droplets (L) and β-glycogen particles (β-gl). The elongate cytoplasmic process of the interstitial cell (IS), with a few mitochondria (m), is shown in the lower right corner of the micrograph.
3.2 Stage I. Immature stem cells of the gonial type
The undifferentiated cells of the gonial type (Fig. 1A), situated at the periphery of the vitelline follicles, represent the precursors of vitellocytes. They have a high nucleo-cytoplasmic ratio. Their large nuclei measure ∼5 μm in diameter, whereas the diameter of the entire cell is ∼6.5 μm. At the end of this stage, however, some increase in cell size takes place (compare Fig. 1A and B), reaching ∼7–8 μm in diameter, especially in the volume of cytoplasm (Fig. 1B). The cell nucleus exhibits the presence of several electron-dense islands of heterochromatin in addition to the nucleolus and numerous pores in its nuclear envelope (compare Fig. 1A and B). The much thicker layer of granular cytoplasm contains numerous mitochondria and free ribosomes, a few Golgi complexes and a very few short profiles of granular endoplasmic reticulum (GER) (compare Fig. 1A and B).
3.3 Stage II. Early differentiation: glycogenesis, lipid accumulation, shell-globule formation
Early cytodifferentiation of vitellocytes (Fig. 2A–C) is characterized by:
- • a distinct increase in cell size;
- • an increase in the number of mitochondria and both GER and smooth endoplasmic reticulum (SER) cisternae;
- • the formation of the first β-glycogen particles, which are progressively grouped into small glycogen islands (Fig. 2B).

A–C. An early differentiation stage: stage II and the beginning of stage III. A. Details of two vitellocytes at an early stage (stage II) situated in the left and right side corners, separated by a cell at a more advanced stage of differentiation (stage III). Note: (1) numerous parallel cisternae of smooth endoplasmic reticulum (SER) in the perinuclear cytoplasm of the two cells at stage II, and (2) mitochondria (m), granular endoplasmic reticulum (GER), Golgi vesicles (G) and a large, irregularly shaped lipid droplet (L) apparently undergoing fusion in the middle cell at stage III. β-gl: beta-glycogen; N: nucleus. B. Details of vitellocytes at the early differentiation stage (stage II) after Thiéry's cytochemical test for glycogen. Note: (1) a few heterochromatin islands (Hch) in the nucleoplasm; and (2) in the cytoplasm: (a) several accumulations of β-glycogen particles (β-gl); (b) numerous mitochondria (m); and (c) electron-dense lipid droplets (L). G: Golgi complexes; N: nucleus; v: vacuole. C. Details of the vitellocyte cytoplasm (stage III). Note: (1) the development of parallel, concentrically arranged cisternae of the GER surrounded by a Golgi complex (G) and dilated Golgi vesicles that produce dense, proteinaceous shell-globule clusters (sgc) situated in the central part of the cell; (2) several lipid droplets (L), mitochondria (m) and two shell-globule clusters closely adjacent to the cell plasma membrane. Inset: higher-power detail of the shell-globule clusters and β-glycogen particles (β-gl) adjacent to cell plasma membrane.
Initiation of secretory activity by both the GER and SER results in:
- • the appearance of lipid droplets associated with SER cisternae;
- • the formation of shell-globules within membrane-bound vesicles of Golgi origin within the numerous concentrically arranged Golgi complexes (Fig. 3A) which are in turn surrounded by concentric GER cisternae (Figs. 2C and 3A).
Fusion of small shell-globules into much larger shell-globule clusters starts very rapidly and has already begun by this stage (Fig. 2C). The glycogen islands are frequently situated around and between the lipid droplets (Figs. 2B and 3A, B).
3.4 Stage III. Advanced maturation: rapid formation of shell-globule clusters and accumulation of lipid droplets
During the advanced phase of vitellocyte maturation (Fig. 3A, B), the cell doubles in size due to a great accumulation of large lipid droplets and numerous shell-globule clusters, accompanied by a progressive vacuolization of its cytoplasm. Adjacent shell-globule clusters frequently become fused into much larger clusters (Figs. 2C and inset, and 3A, B). At the same time, the nucleus begins to change in form to the semi-lunar shape characteristic of the mature vitellocytes of A. menezesi. Vitellocyte maturation is characterized as a period of a very high secretory activity, resulting in shell-globules and their rapid fusion into shell-globule clusters (Fig. 3A, B).
3.5 Stage IV. Mature vitellocyte
The mature vitelline cells (Fig. 4A) are ovoid or spherical in shape and measure ∼12–14 μm in diameter. Their nuclei are semi-lunar or reniform and measure ∼7 × 3.5 μm in diameter (Fig. 4A). The karyoplasm contains an elongate nucleolus and a few irregularly shaped heterochromatin islands of moderate electron density. The highly vacuolated cytoplasm contains numerous large lipid droplets of moderate electron density, being moderately osmiophilic, and great amounts of large, membrane-bound shell-globule clusters composed of numerous shell-globules separated by a lucent phenolase component. Both of these cell inclusions (Fig. 4A, B) accumulate mainly in the peripheral layer of the cytoplasm of mature vitellocytes.
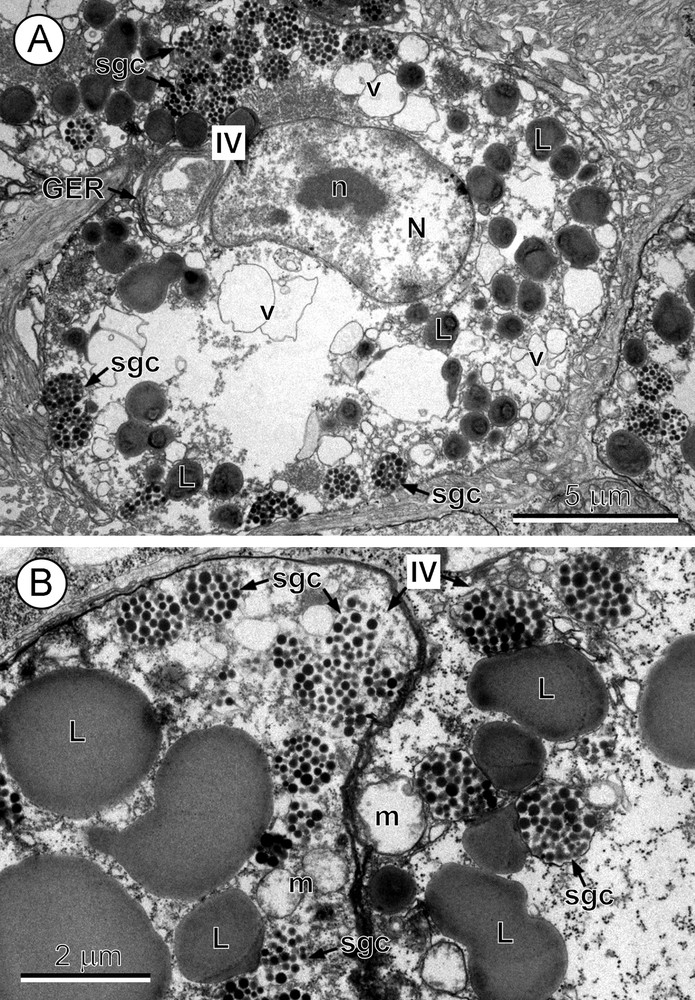
A-B. Mature vitellocyte or stage IV. A. A mature vitellocyte. Note: (1) the nucleus (N) with a semi-lunar shape and prominent nucleolus (n); (2) the highly vacuolated cytoplasm with a few parallel cisternae of granular endoplasmic reticulum (GER) in the perinuclear region, and numerous lipid droplets (L) and shell-globule clusters (sgc) grouped mainly in the peripheral cytoplasm. v: vacuole. B. High-power micrograph of the peripheral cytoplasm of two mature vitellocytes. Note: (1) numerous very large, moderately saturated lipid droplets (L); (2) a few large mitochondria (m); and (3) numerous membrane-bound shell-globule clusters (sgc) grouped mainly in the peripheral region; each cluster is composed of numerous electron-dense but rather small individual shell-globules, and all are embedded in an electron lucent phenolase component.
4 Discussion
Early TEM studies on cestode vitellogenesis, published during the 20th Century, were reviewed and analysed in relation to egg production, different types of embryonic development and a variety of parasite life-cycles [7]. TEM results in more recent studies on this subject have been dealt with in both the “Discussion” and “Table I” of a paper on vitellogenesis of the bothriocephalidean cestode Clestobothrium crassiceps [13].
A. menezesi has been attributed to the trypanorhynch family Gilquiniidae [1,4,14] and, more recently, the Aporhynchidae [15–17]. The general pattern of vitellogenesis in this species is essentially similar to that reported for other species of lower cestodes, i.e. gyrocotylideans [18], amphilinideans [19], spathebothriideans [20–22], caryophyllideans [23–27], diphyllideans [28], trypanorhynchs [8–11], rhinebothriideans [29] and bothriocephalideans [30–32].
During vitellogenesis in all of these cestode taxa, the synthetic activities of differentiating vitellocytes involve three processes:
- • protein synthesis, producing important material for eggshell formation;
- • glycogenesis, which assures glycogen storage and nutritive reserves for the developing embryos;
- • lipid accumulation in the form of large lipid droplets of a more or less saturated chemical nature, which are considered to be another source of nutritive reserves for the embryo.
There is, however, evident variation in the chronology of these three types of synthetic activity, as it is only very seldom that they take place simultaneously.
Protein synthesis is the predominant synthetic activity, which usually occurs in the very early stages of vitellogenesis in the great majority of tapeworms; however, in A. menezesi it is evidently delayed. Proteinaceous globules, which are progressively grouped together into larger subunits, such as shell-globules and then small globule clusters, are synthesised in the GER and packaged into small globules via the Golgi complex; this is a general feature of platyhelminth vitellocytes [7] and also of other cell types involved in protein synthesis for external utilization [33].
However, variation in the formation and storage of embryonic nutritive reserves in the various cestode groups has been observed in relation to differences in the proportion and amounts of glycogen and lipids [7,13,28,34]. There are extreme cases, such as the caryophyllideans, where glycogen only is accumulated in both the vitellocyte cytoplasm and its nucleus [23,24,26,27]; another such extreme example occurs in rhinebothriidean vitellocytes [29], where generally lipids only are accumulated in both the cytoplasm and the nucleus. A recent comparison of both types of embryonic nutritive reserves in the vitellocytes of lower cestodes has been presented [13].
In the present study, particular attention was paid to comparing the ultrastructural and cytochemical aspects of vitellogenesis and the functional ultrastructure of the mature vitellocytes of A. menezesi with those of the four other studied species of the Trypanorhyncha [8–11], which belong to three other families of this order. It appears that ultrastructural details of vitellogenesis, and in particular details of the mature vitellocytes, exhibit a great diversity in all five of these trypanorhynch species (Table 1). Great variation was observed in the sequence of the two main synthetic activities, namely shell formation and the development and storage of nutritive reserves for the developing embryos; the latter occur in the form of glycogen and lipids, and are usually represented in different proportions in the five species. Both types of synthetic activity can take place at both the early and the advanced stages of vitellocyte differentiation, although they do not coincide in Parachristianella trygonis [11], slightly overlap in D. spinulifera [9] and occur simultaneously in Progrillotia pastinacae [10]. Nevertheless, regardless of the group, proteinaceous globules (shell-globules) are evidently synthesized in the GER and packaged as small globules via the Golgi complex, as occurs in all studied platyhelminth species [7].
Comparison of the ultrastructural characters of vitelline material in five trypanorhynch species.
Trypanorhynch families and species | Host species and families | Host locality | Glycogen: amount and type | Membrane-bound glycoproteins | Lipid droplets: amount and chemical nature | Reference |
Aporhynchidae | ||||||
Aporhynchus menezesi Noever et al., 2010 | Etmopterus spinax (Etmopteridae) |
Horta, Faial, Azores Islands (Atlantic Ocean) | Moderate amount of β particles, mainly in immature vitellocytes | Not studied | High amount; moderately saturated character | Present paper |
Lacystorhynchidae | ||||||
Grillotia erinaceus (van Beneden, 1858) |
Raja clavata (Rajidae) |
Irish Sea | High amount; both α-rosettes and β particles | Not studied | Moderate amount; moderately saturated character | [8] |
Eutetrarhynchidae | ||||||
Dollfusiella spinulifera (Beveridge and Jones, 2000) |
Rhinobatos typus (Rhinobatidae) |
Heron Island, Australia (Coral Sea) | Only very few β particles, mainly in immature vitellocytes | Attached to all membraneous structures such as GER, Golgi, mitochondria, nuclear and cell plasma membrane | Very large amount and large size of unsaturated, highly osmiophilic droplets | [9] |
Parachristianella trygonis Dollfus, 1946 | Dasyatis pastinaca (Dasyatidae) |
Sidi Mansour and Zarzis, Tunisia (Mediterranean Sea) |
High accumulation of α-rosettes and β particles | Not studied | High amount; moderately saturated character | [11] |
Progrillotiidae | ||||||
Progrillotia pastinacae Dollfus, 1946 | Dasyatis pastinaca (Dasyatidae) |
Sidi Mansour, Tunisia (Mediterranean Sea) |
Only few traces of β particles adjacent to lipid droplets | Not studied | Very high acumulation; highly saturated, osmiophobic droplets | [10] |
The most important differences observed in the mature vitellocyte of D. spinulifera, Para. trygonis and Pro. pastinacae [9–11] relate to glycogenesis (Table 1). Of these three species, only Para. trygonis has large amounts of glycogen. In D. spinulifera, cytochemical overstaining with periodic acid-thiocarbohydrazide-silver proteinate for polysaccharides indicated a strongly positive reaction for membrane-bound glycoproteins in all membranous structures, such as GER, mitochondria, Golgi complexes, nuclear and cell plasma membranes, where there were only membrane-bound polysaccharides and very few granules of β-glycogen. Similar staining in A. menezesi revealed β-glycogen particles scattered in the cytoplasm of maturing vitellocytes. Typical cytoplasmic β-glycogen particles appeared only seldom during early vitellocyte maturation and were rarely visible in mature vitelline cells of D. spinulifera [9] and Pro. pastinacae [10]. Another important difference between the four species is the lipid content and the chemical nature of the lipid droplets (Table 1). In Para. trygonis, there are massive concentrations of lipids which are, like those in Grillotia erinaceus and Pro. pastinacae, of the saturated type. In all five of the trypanorhynch species, lipid droplets were localised only in the vitellocyte cytoplasm, never inside the cell nucleus as reported for the tetraphyllidean Echeneibothrium beauchampi [29] or the spathebothriidean Didymobothrium rudolphii [22]. Studies by Smirnov and Bogdan [35] have shown that the differences in the level of lipid saturation between the former pseudophyllideans Eubothrium crassum (Bothriocephalidea) and Diphyllobothrium dendriticum (Diphyllobothriidea) are related to the body temperature of their definitive hosts. Although the temperature difference among the hosts in the present study is not as great as between these two cestodes, it is of interest that highly unsaturated lipids were present only in D. spinulifera (Table 1), which is from a warm, semi-tropical marine environment. The ultrastructural examination of the early cytodifferentiation of the vitellocytes (Fig. 2A) in A. menezesi exhibits numerous largely dilate cisternae of SER, which are usually associated with metabolism and the transport of lipids [33]. Lipids represent a highly diverse and heterogeneous group of chemical compounds, with a great variety of cellular functions. They are generally considered as important energy reserves, although this may not be always the case in cestodes [36]. In relation to the function of large lipid deposits, two theories prevail, i.e. they represent either an energy source or a waste product of metabolism. Studies on the ultrastructure of the free-swimming coracidial larva of Bothriocephalus clavibothrium [37] provide strong arguments for their function as important energy reserves. The significance of such unusually high accumulations of lipids and the wide variety of their chemical nature in cestode vitellocytes appears somewhat difficult to ascertain. In TEM studies, however, lipid droplets in cestode vitellocytes exhibit different degrees of saturation, which are manifest as differences in their affinity for osmium in ultrathin sections, e.g. unsaturated lipids are highly osmiophilic and appear black in the ultrathin sections, whereas saturated lipids are highly osmiophobic and appear white in these sections. The studies of Buteau et al. [38] and Beach et al. [39] on lipids of tetraphyllidean and trypanorhynch cestodes from sharks may offer some insights into the origin, metabolism and role of the lipids in these parasites. Since cestodes are incapable of de novo synthesis of non-volatile saturated and unsaturated fatty acids, the biosynthesis of lipids depends on the host for the fatty acids [38,40]. The fatty acid composition of cestodes is, therefore, similar to that of their immediate environment in the host [39] and the origin of these fatty acids in the host is the host's food chain. It can be concluded that the fatty acid patterns in all trypanorhynch lipids is host-related. According to these data, it is therefore not surprising that there is so much variation in the qualitative and quantitative aspects of lipids in the vitellocytes of trypanorhynchs (Table 1), because those cestodes examined came from five different hosts and from four different marine environments, the Irish Sea, the Atlantic Ocean off the Azores, the Mediterranean Sea and the Coral Sea.
Lack of data on the life-cycles and developmental biology of the Trypanorhyncha [8,15,41] makes it difficult to speculate on the functional ultrastructure of their vitellogenesis and mature vitellocytes. No doubt, there is a close interrelationship between the pattern of vitellogenesis in parasitic platyhelminths and the type of embryonic development, the poly- or oligolecithality of their eggs and the different degrees of ovoviviparity [7,24,42–44]. Very little is known about trypanorhynch life-cycles due to difficulties in maintaining them alive and creating effective exposure conditions in the laboratory. In general, their life-cycles are heteroxenous, involving several different invertebrate or vertebrate intermediate hosts and an elasmobranch definitive host [8,15,41]. The gravid segments may remain attached to the rest of strobila or may detach progressively from the mature proglottids. Two developmental pathways have been reported:
- • hexacanth larval development within the egg is arrested in the uterus after the first few cell divisions, i.e. at an early embryo stage, representing different degrees of ovoviviparity; development resumes almost immediately on contact with seawater and infective hexacanths, surrounded by a ciliated envelope, are produced within 5–8 days;
- • intrauterine eggs contain fully-formed oncospheres [8,15,41,45]. Further studies may show a greater variety of trypanorhynch egg types than are known at present.
According to Noever et al. [46], the eggs of A. menezesi differ conspicuously from those of Aporhynchus norvegicus. In their opinion, the illustration of eggs published by Dollfus [3], which he attributed to A. norvegicus, closely resembles the eggs of A. menezesi, which they examined. In their view, Dollfus’ material consisted of a mixture of specimens of both A. norvegicus and A. menezesi, and that he in fact illustrated the eggs of A. menezesi rather than A. norvegicus. Our diagram of the egg of A. menezesi (Fig. 5) is a modification of Dollfus’ illustration [3]. As described by Dollfus [3], the fusiform eggs of this species are very unusual. They are so delicate that they were only successfully examined and measured by him when observed directly in seawater. Any kind of fixation, dehydration or embedding resulted in their complete deformation and numerous artefacts. The fusiform eggs, measuring 155–165 μm in length by 45–55 μm in width, are surrounded by a very thin outer envelope, which is prolonged at one pole to form a short filament. Inside each egg (Fig. 5), there appear to be five or six compartments in line, and the embryo occupies only one, apparently any single one, of these compartments. Such an early embryo is composed of several blastomeres surrounded by a cluster of three or four entities, interpreted as being vitelline cells, arranged usually in tandem on either side of the embryo. The presence of such eggs with early embryos may suggest that hexacanth development within the eggs is arrested in the uterus after several initial cleavage divisions, and that it resumes almost immediately upon contact with seawater, infective hexacanths typically being produced within a few days [46]. Differing quantitative and qualitative concentrations of glycogen and lipids, as described in the present study, may be associated with physical and physiological adaptations that enhance transmission. However, until we have more information on the life-cycle of these cestodes, the variety of egg types and their adaptations to different hosts and environmental conditions, it will be difficult to judge to what extent the ultrastructure of the vitellocytes, and in particularly those with lipids, is a reflection of host influence, ecological adaptations or phylogenetic relationships.

Diagram of the egg of Aporhynchus menezesi. Modified after Dollfus [3]. Bl: blastomeres; C: capsule; EC: egg compartment.
Disclosure of interest
The authors declare that they have no conflicts of interest concerning this article.
Acknowledgements
Adult specimens of A. menezesi used for present study were kindly collected, fixed and sent us by Prof. Janine Caira. The collecting was partly supported by funds from NSF PBI award Nos DEB 0818696 and 0818823 to Janine N. Caira, Timothy J. Littlewood, Jean Mariaux and Kirsten Jensen. The authors also wish to thank Núria Cortadellas and Almudena García from the “Unitat de Microscòpia, Facultat de Medicina, Centres Científics i Tecnològics de la Universitat de Barcelona (CCiTUB)” for their support in the preparation of samples. Adji Mama Marigo benefits from MAEC-AECID doctoral grants (2009-10 0000448124 and 2010-11 0000538056.