1 Introduction
Phragmites australis (Cav.) Steud. is one of the most dominant and common species in wetland ecosystems from temperate regions worldwide [1–3]. It is tolerant to a broad ecological range, colonizing different habitat types, such as riverbanks, ditches, littoral zones of lakes, fens, bogs and salt-marshes. This sub-cosmopolite species can grow in oligotrophic to eutrophic conditions, but it seems to be favoured in nutrient-rich sites [4]. The common reed can also withstand different pollutants, including heavy metals. For this reason, it is often used for the treatment of industrial or agricultural wastewaters [5–9], for phytoremediation [10–12] and even for the removal of harmful microorganisms due to its allelopathic effect [13].
In some areas of the world this grass is regarded as an aggressive invasive species [14–17]. The phenomenon is prominent in the USA where the Eurasian subspecies P. australis subsp. australis outcompetes the native, recently described, P. australis subsp. americanus Saltonstall, P.M. Peterson & Soreng, displacing the authoctonous populations [18]. For this reason, in N-America and Canada P. australis subsp. australis was included among the highest priority invasive species [19–24]. On the other side, reed beds represent valuable ecosystems for biodiversity conservation, although they are both usually species-poor when compared with other vegetation types [25–29] and not included in any specific European list of protected habitats [30]. Reed extensive communities play important structural and functional roles in wetland ecosystems, as they work as efficient filters for pollutants and provide habitat for fauna species [31–35]. Large reed beds in Europe are considered valuable and often threatened ecosystems [36] and even in North America some scientists do emphasize the importance of both habitat and non-habitat ecosystem services provided by reed [37,38].
In the last decades, in Europe P. australis (in all likelihood the Eurasian subspecies, although there is often no explicit indication of the subspecies) has suffered a dramatic decline, known in literature as ‘common reed dieback’ [39–42]. Among the reported symptoms affecting this species, the most frequently observed are: clumped habit, weaker culms with reduced height and diameter, dead rhizomes and buds, flowering delay, abnormal lignification and suberization, presence of callus, and an evident retreat especially from deep waters [for a general overview: 39,41]. Several hypotheses were proposed to explain this process [43–46]. Increasing eutrophication and artificial changes in the water table, together with anoxic conditions and methanogenesis processes in the sediment, were regarded as primary causes of this phenomenon [47–50].
For a long-time, reed dieback was considered as a Central European issue as it was not occurring in relatively warm areas such as the Mediterranean Basin, likely due to different biogeochemical processes developing in this climatic environment [41,51,52]. However, in recent years this phenomenon was detected and described also in some Mediterranean localities, from both brackish and freshwater wetlands [53–57].
The Italian ecosystem where reed decline was most extensively detected is Lake Trasimeno, the largest lake in Peninsular Italy [27,28,54–56,58,59]. The reed-dominated macrophytic vegetation along the shores of this shallow lake showed a progressive deterioration during the last 50 years. It became evident not only for the remarkable reed retreat and dieback, but also through a progressive decline of the water conditions and a general loss of biodiversity [54,60–62]. According to a number of macro- and micro-morphologic and hysto-anatomic traits, some hypotheses were formulated in order to understand the processes driving reed decline at Lake Trasimeno [54,56].
In the wake of such a baffling detection, macroscopic symptoms of dieback were observed in other freshwater ecosystems from Central Italy, such as Lake Chiusi in Tuscany. Differently from the nearby Lake Trasimeno, Lake Chiusi has always maintained relatively good floristic conditions, preserving plant species already disappeared from Lake Trasimeno [60,63,64]. However, a visible retreat of reed beds from the waterfront in several parts of the lakeshore and an evident discontinuous, clumpy growth of the reeds could be observed.
This study is part of a general effort to understand the physicochemical processes affecting delicate ecosystems at high risk of degradation and loss, often caused by human-driven changes to land use [65–70]. Our main driving hypotheses are that:
- • the reed dieback should be considered also as a Mediterranean issue;
- • multiple ecologic traits might co-occur with, and concur to, reed decline, thus, suggesting that this dramatic phenomenon should be approached with an integrated, multidisciplinary investigation.
This approach takes the cue from similar outcomes in Central Italy, suggesting that permanent flooding might be associated with reed decline by inducing chemical reactions in the sediment [54,58,59]. In this light, the main goals of our survey were those to:
- • verify and quantify the actual reed bed retreat at Lake Chiusi, with reference to its spatial distribution, and identify the most affected areas;
- • investigate whether the observed macroscopic symptoms of decline can be related to the dieback syndrome;
- • assess whether some ecological (including chemical) characteristics of sediments and interstitial waters play a major role in reed dieback and determine their relationship with the presence/absence of macromorphological symptoms.
2 Materials and methods
2.1 Study site
Lake Chiusi (surface 3.6 km2, average depth ∼ 2.70 m, maximum depth 5.70 m) is located in the Chiana Valley (southern Tuscany, Central Italy; Fig. 1), at an average altitude of 251 m a.s.l. [71], and 10 km SW from Lake Trasimeno, the first lake in Peninsular Italy where the reed dieback syndrome was detected [54]. From a geological point of view, the study area is characterized by Quaternary sands, pebbles and muds [72]. The climate of the area is humid Mediterranean [73].
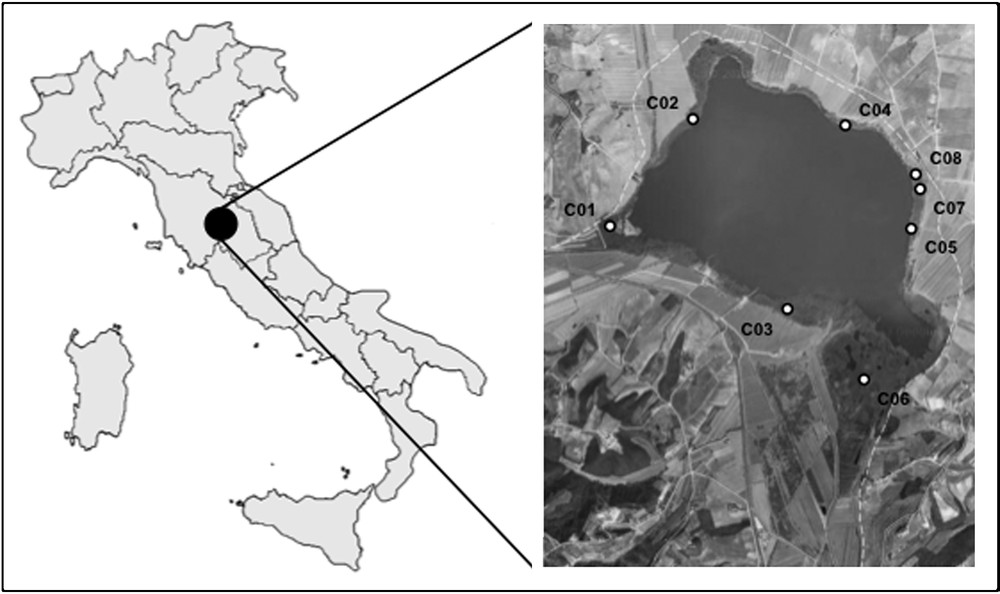
Location of Lake Chiusi with the indication of the studied plots (white circles) and their ID. The white dashed line indicates the administrative border between Umbria and Tuscany Regions.
The only tributary of the lake is the Tresa Stream; waters are drained by an artificial outlet to Lake Montepulciano and from there to the Arno River. Water levels of the lake were taken into account to point out whether flooding or drought significant events took place in the past, which might have had an effect on the reed stands. Unfortunately, only the yearly maximum levels were recorded at Lake Chiusi. According to the data, kindly provided by the Hydraulic Office (Soil Protection Service) of Arezzo Province, the maximum level of the water table in the period back to 1988 was around 249.01 ± 0.11 (av ± se) m a.s.l., reaching 249.11 ± 0.08 m a.s.l. when data back to 1973 are taken into account.
Chiana Valley has been subjected to a long-time reclamation history that has deeply modified the landscape of this territory [74,75]. The Lake Chiusi and Montepulciano represent the two major wetlands of the Chiana Valley. Together with a series of human-made minor wetlands, such as ponds and canals, they are dispersed in a landscape-matrix with strong agricultural vocation [76,77]. The study site is included in a Natural Protected Area of Local Interest (ANPIL); according to the European 92/43/EEC ‘Habitats’ Directive, it was designed as Site of Community Importance (SCI).
2.2 Detection of reed retreat
In the last decade, worrying signs of reed retreat at Lake Chiusi were noticed by fishermen and site managers. We carried out a diachronic analysis comparing the reed bed front in different periods to check and quantify the actual occurrence of reed retreat. On the basis of 1988 and 2008 digitized aerial images (orthophotos), available as WMS Service on the National Geoportal of the Italian Ministry of the Environment and Protection of Land and Sea (http://www.pcn.minambiente.it/GN), both including the study area at a scale of 1:10,000, we could perform a comparison over 20 years. The images were processed by using the Open Source Geographic Information System Quantum GIS (QGIS 2.0.1), licensed under the GNU General Public License (GPL) v.2, Free Documentation Licencse v.1.3 [78]. In order to estimate the reed bed surface in the 2 periods, we used heads-up digitizing, that is a manual digitization by mouse for vectorizing raster data. The measured surfaces in the two periods (1988 and 2008) were compared in order to quantify the reed bed spatial variation. Furthermore, the retreat of the reed bed waterfront over the considered period was measured in 24 spots (including the eight sampling sites later described) regularly scattered along the lakeshore, positioned at about 400–450 m from each other. For each spot, the retreat was measured along a straight line orthogonal to the reed bed front. The performed measurements allowed to estimate the average, minimum and maximum shift back of the reed bed waterfront.
2.3 Sampling sites
Following the experimental design proposed for similar studies in Central Italy [54–58], eight survey sites were set along the shore of Lake Chiusi (Fig. 1). For the site selection, we adopted a stratified-random criterion, considering permanently dry/permanently flooded/partially flooded reed stands (named ‘D’, ‘F’ and ‘M’, respectively). The sites have been considered as permanently flooded (F) if they stayed covered by water (at least 10 cm depth) even in the driest season of the year (end of summer). The partially flooded sites (M) refer to the so-called ‘floating mats’ originated by a spontaneous entanglement of reed rhizomes colonized by new reed and kept afloat in fluctuating water levels. The whole set of sites included both stands without any evident macroscopic sign of decay and stands seemingly affected by decline and/or retreat, as also resulting from the cartographic analyses. In particular, macroscopic signs of decay were observed in the Northern and Southeastern sectors of the lake.
For each site, one plot (1 m2) was stratified-random sampled, georeferenced and named by an ID, from C01 to C08 (WGS84 lat., long. of the eight plots, expressed as degrees/minutes/decimal minutes: C01: 43°03.313′, 11°56.954′; C02: 43°03.745′, 11°57.397′; C03: 43°02.962′, 11°57.811′; C04: 43°03.704′, 11°58.273′; C05: 43°03.298′, 11°58.651′; C06: 43°02.657′, 11°58.457′; C07: 43°03.400′, 11°58.732′; C08: 43°03.434′, 11°58.731′). Group M includes C01 and C05 plots, group F includes C02, C03, C04, C06 plots and group D is formed by C07 and C08 plots. The flooding conditions, on which the subdivision of the D-M-F groups was based, were measured in the eight plots. Those belonging to the F group (P02, P03, P04, P05) were covered by 37.2 ± 18.2 cm water (average ± sd) in the driest season of the year (end of summer). The P01 and P05 plots (group M), corresponding to the floating mats, were at surface all year long, although the lower parts of the root system were freely floating in the water column below the mat. The plots P07 and P08 (group D) and their soil were totally dry at the end of the summer.
For each site, two different sets of parameters, macromorphological and environmental, were measured.
2.4 Macromorphological parameters
The macromorphological parameters were measured in the fields on reed individuals in each plot. As reed is a clonal species, with the term ‘individual’, we refer to the concept of ‘ramet’, a potentially independent functional unit of a clonal plant that can properly be used for demographic studies [79–81]. The considered parameters include: stem height (cm), stem diameter (mm), stem density (number of stems m−2), clumping habit (number of clumped stems m−2 and %), presence of inflorescences (number of flowering heads m−2 and %), presence of dead apical buds (number of dead buds m−2 and %). The stem height was measured with a metric tape, including the portion between the ground and the base of the inflorescence; when the stand was submerged, the underwater portion of the stem was added. The diameter was measured with a gauge at about 120 cm above ground, in analogy with similar studies carried out at Lake Trasimeno [54]. Height and diameter were measured in each plot on 10 reed stems randomly chosen, for a total amount of 80 individuals. The density was measured in each plot by counting the total number of stems. The presence of clumping habit, caused by breaking of apical dominance and resulting in uncontrolled outgrowth of dormant buds [41], was quantified by counting the number of clumped stems in each plot. This value was related to the total stem density, in order to obtain the rate of clumped stems. The presence of inflorescences and dead apical buds was also surveyed as both absolute number and rate.
All measurements were carried out in late August/early September 2011, at the end of the reed vegetative season, corresponding to the reaching of the maximum standing crop [82].
2.5 Environmental parameters
The environmental parameters include: water depth, total vegetation cover, chemical parameters related to interstitial waters and sediments. They were surveyed in the same period of the acquisition of the macromorphological parameters. The water depth was measured in cm in the middle of the plot, by a metric tape with a lead weight. The total vegetation cover was estimated as the projection on the ground of the reed bed foliage, by using a percentage scale according to Braun-Blanquet [83]. One sediment sample for each plot, including interstitial waters for each of the submerged ones, was collected in the shallower portions of the sediments (5-cm depth), minimizing any contamination by superficial water. Samples were stored in sterile plastic bags (500 mL) and conserved at about 5 °C for 24 h before the preparation for the chemical analyses. In the laboratory, water and sediment were left in their plastic containers for 1 day, allowing the solid and liquid phase to separate. Interstitial water was then removed with a syringe and filtered at 0.45 μm to obtain three aliquots, the first of which was transferred into 125-mL polyethylene bottles for the analysis of pH, electrical conductivity and the main anions (HCO3, Cl, SO4, NO3, F, and Br). The second aliquot was transferred to 50 mL polyethylene bottles and acidified with 0.5 mL of suprapure HCl (30%) for the analysis on the main cations (Ca, Mg, Na, K and NH4). Finally, a third aliquot was transferred to 50 mL polyethylene bottles and acidified with 0.5 mL of suprapure HNO3 (70%) for the determination of trace elements (Al, Sb, Ba, B, Cd, Cs, Co, Cr, Cu, Fetot, Hg, Mn, Ni, Ptot, Pb, Rb, Se, SiO3, Sr, Y, Tl, U, and Zn).
The lake sediments were dried at 40 °C and then sieved at 2 mm. The < 2-mm fraction was then grinded with a planetary mill equipped with agate mortars. Sample dissolution was carried out with a mixture of HNO3–HF–HCl in PTFE containers for the determination of As, Cd, Co, Cr, Cu, Fe, Hg, Ni, Pb, and Zn.
Main anions and cations were analysed by using Metrohom 761 and Metrohom 861 ion-chromatographers, respectively, whilst HCO3 was analysed by acidimetric titration with 0.01 M HCl and methyl-orange as indicator. The analytical error was < 3%. Trace elements of the interstitial waters and sediments were analysed by ICP-MS with a CP-MS 7500ce (Agilent Technologies) at the Gruppo CSA Ltd. Environmental Chemistry and Microbiology laboratories. The analytical error was < 10%.
2.6 Statistical treatment of the data
The statistical significance of the differences for the macromorphological variables among the three considered types of reed stands (D: permanently dry; F: permanently flooded; M: partially flooded) was analysed by applying nonparametric statistical methods (Kruskal–Wallis Test and Mann–Whitney U-Test) to point out the pairs showing higher differences [84–86]. Changes in the measured reed traits from the 8 plots were related to the chemical parameters, both in the interstitial waters and sediments, by applying a regression analysis based on Spearman's rank correlation coefficient. In this model, reed traits represented the dependent variables, and the chemical parameters were used as individual explanatory variables [87]. The interstitial water parameters were computed only for the F and M groups (6 plots), since the D group refers to dry plots.
Ordination analysis through Detrended Correspondence Analysis (DCA) [88] was performed in order to investigate the major gradients in the measured macromorphological parameters from all plots. As a consequence, a linear method, the Principal Component Analysis (PCA), was adopted to analyse the data set because of the relatively short length of the main gradient (0.7 SD) [89]. This unconstrained ordination was applied to find the axis with maximum variation and thus describing the general patterns in reed traits distribution along the gradients [89]. The PCA diagram was subsequently passively projected with the sediment chemical parameters to show their variations across the main gradient. The Pearson's Correlation Test was performed to examine relationships between sample scores and sediment chemical variables on PCA axes 1 and 2, respectively. As the reed traits and sediment chemical data were expressed in incompatible units, prior to ordinations, they were reduced to a common scale (the interval 0–1) by first subtracting the minimum observed for each variable and then dividing by the range [90]. This method allowed the simultaneous adjustment of the magnitude and the variability of the descriptors.
All the univariate statistical analyses were performed by Analyst Soft StatPlus©: mac v2009, while the ordinations were run with Canoco 4.5 for Windows [91].
3 Results
3.1 Retreat of the reed bed
The measurements aimed to detect any reed bed retreat at Lake Chiusi, based on a diachronic analysis of aerial photos, showed that in two decades (1988 to 2008) a remarkable spatial variation backwards of the reed front took place along the whole lakeshore. The reed bed front moved back of 49.2 ± 14.3 (av ± se, n = 24) m on average. The strongest variation affected the SE sector of the lake, where the retreat reached its maximum value (274.9 m). There are no sectors of the lake where the reed bed waterfront did not retreat: the minimum variation was 10.5 m.
Cartographic results showed that the total reed surface was 121.38 ha and 58.14 ha in 1988 and 2008, respectively, with a loss of 63.24 ha in two decades, corresponding to more than 52% (Fig. 2). It can be noticed that the largest loss affected the waterside of the reed bed and it was particularly dramatic in the southeastern sector of the lake.
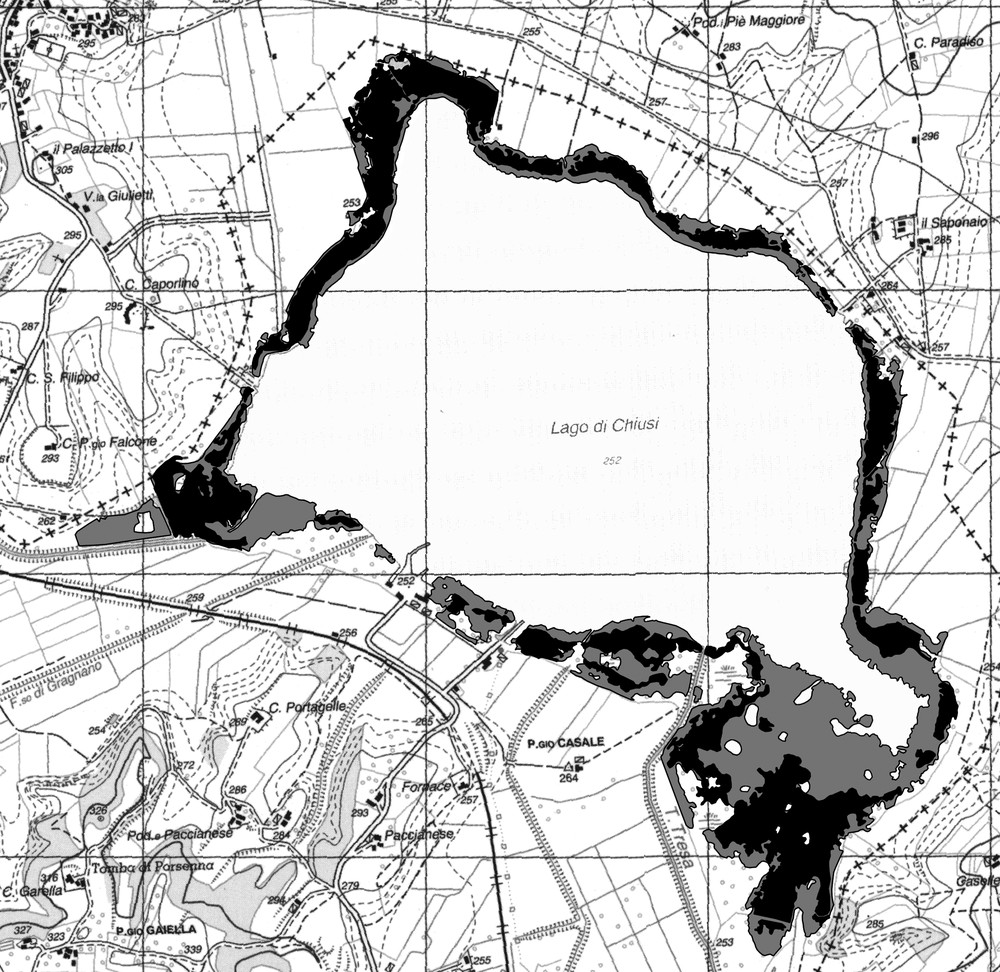
Surface of the reed bed at the Lake Chiusi in 1988 (grey) and 2008 (black), as resulting from a process of heads-up digitizing that produced vectorized data from raster digitized orthophotos; the grid side corresponds to 1 km (the reported cartographic base has been redrawn from CTR Technical Regional Map, scale 1: 10,000, Umbria Region).
3.2 Reed macromorphology
The data deriving from the macromorphological measurements in the reed population at Lake Chiusi are reported in Table 1, where the average, maximum and minimum values for the whole data set (n = 80) are indicated. The individual size is widely variable for both height (from 0.70 to 4.05 m) and diameter (from 1.08 to 12.30 mm) of the stems. The presence of prematurely dead apical buds was detected in all the plots, ranging from a rate of 3.40 to 43.93% of the total number of stems per plot (corresponding to a net density of 5 to 47 stems m−2). The clumped habit is affecting 37.5% of the plots, ranging from nil to 100% of the stems (34.78% on average). Stem density resulted also largely changeable, ranging from 65 to 222 stems m−2. The flowering rate ranges from 11.20 to 56.92% and never involves the total number of stems. This can be attributed to an obvious consequence of the constant presence of a certain rate of dead summits.
Minimum, maximum and average values of the measured macromorphological parameters and vegetation cover in the analysed plots for the whole data set; average values ± standard errors for the 3 groups (F, D, M, respectively: permanently flooded/permanently dry/partially flooded reed stands).
Groups | Whole data set | F | D | M | ||
av | min | max | av ± se | av ± se | av ± se | |
Stem height (m) | 2.29 | 0.70 | 4.05 | 2.13 ± 0.13a* | 2.19 ± 0.12a* | 2.70 ± 0.15b* |
Stem diameter (mm) | 5.96 | 1.08 | 12.30 | 5.31 ± 0.29a** | 5.48 ± 0.31a** | 7.75 ± 0.56b** |
Dead buds (n m−2) | 24.1 | 5.0 | 47.0 | 26.0 ± 10.1 | 26.0 ± 6.0 | 18.5 ± 8.5 |
Dead buds rate (%) | 20.79 | 3.40 | 43.93 | 21.00 ± 8.42 | 21.73 ± 3.87 | 19.43 ± 4.05 |
Clumping rate (%) | 34.78 | 0.00 | 100.00 | 67.39 ± 21.34 | 0.00 ± 0.00 | 4.35 ± 4.35 |
Stem density (n m−2) | 120.1 | 65.0 | 222.0 | 136.0 ± 32.9 | 118.5 ± 6.5 | 90.0 ± 25.0 |
Flowering heads (n m−2) | 32.6 | 14.0 | 53.0 | 37.3 ± 6.3 | 26.0 ± 12.0 | 30.0 ± 7.0 |
Flowering heads rate (%) | 30.80 | 11.20 | 56.92 | 31.10 ± 7.17 | 22.56 ± 11.36 | 38.46 ± 18.46 |
Total vegetation cover (%) | 90.0 | 60.0 | 100.0 | 80.0 ± 7.4 | 100.0 ± 0.0 | 100.0 ± 0.0 |
With reference to the 3 groups of plots (F, D, M), average values ± standard errors are listed in Table 1 and the statistical significance of the differences detected among the groups, when acceptable (P < 0.05 at least), is also reported. The main differences among the groups were detected with reference to the stem height (P < 0.05) and diameter (P < 0.01), based on the Kruskal–Wallis Test and the Mann–Whitney U-Test (Table 1). Remarkably higher values were measured in the M group, whose plots always show higher and more robust individuals. The lowest average values were recorded in the permanently flooded plots (F) for both height and diameter, while the dry stands (D) presented intermediate values. As far as the other investigated traits are concerned, they show similar, although not statistically significant, differences. The clumping habit was detected in all the F plots with high rates (more than 67% on average), while it resulted absent in the dry stands (D) and only seldom observed in the floating mats (M), with very low rates (never more than 9%). Dead buds are rarer in the plots of the M group, where the stem density is lower and the flowering rate is the highest on average.
3.3 Ecological features
The total vegetation cover, estimated by percentage scale, resulted always higher than 60%, reaching its maximum (100%) in half of the considered plots. Its values resulted remarkably lower in the permanently flooded group of plots, where the reed bed generally appeared thin and sparse (Table 1).
The results of the interstitial water and sediment chemical analyses did not point out any abnormal condition. The average values of the measured parameters in the sediment are reported in Table 2. The regression analysis based on Spearman's rank correlation coefficient highlighted the presence of correlations between some reed traits (considered as dependent variables) and some chemical variables in the sediment. The presence of copper in the sediment appears negatively correlated to the reed stem height. Zinc, nickel, total chromium, cobalt and iron in the sediments are negatively correlated to the reed stem diameters. Iron seems to be directly correlated with the occurrence of clumping when only the flooded and partially flooded plots (F and M) are considered (Table 3).
Average values of the measured chemical parameters (dm: dry matter) in the sediment of the eight analysed plots, grouped according to the three groups (F, D, M, respectively: permanently flooded/permanently dry/partially flooded reed stands).
Units | F (n = 4) | D (n = 2) | M (n = 2) | |
Copper | mg·kg−1·dm | 38.40 | 36.30 | 35.50 |
Lead | mg·kg−1·dm | 24.50 | 18.00 | 49.50 |
Zinc | mg·kg−1·dm | 97.88 | 115.15 | 75.15 |
Nickel | mg·kg−1·dm | 76.00 | 84.65 | 35.10 |
Total chrome | mg·kg−1·dm | 119.15 | 134.10 | 61.55 |
Cobalt | mg·kg−1·dm | 15.65 | 17.40 | 5.25 |
Iron | μg·kg−1·dm | 34.95 | 40.44 | 15.28 |
Cadmium | mg·kg−1·dm | 0.18 | 0.17 | 0.26 |
Arsenic | mg·kg−1·dm | 12.75 | 7.50 | 6.50 |
Mercury | mg·kg−1·dm | 2.51 | 0.99 | 16.30 |
Significant relationships between reed traits and sediment chemical parameters, analysed by linear regressions (Spearman's rank correlation coefficient), with reed traits as dependent variables and the chemical parameters as individual explanatory variables (n = 8).
Slope | r 2 | P | |
Reed stem height | |||
Copper | −0.0807 | 0.61 | 0.02 |
Reed stem diameter | |||
Zinc | −0.0673 | 0.58 | 0.03 |
Nickel | −0.0596 | 0.72 | 0.01 |
Chrome (total) | −0.0407 | 0.72 | 0.01 |
Cobalt | −0.2363 | 0.66 | 0.01 |
Iron | −0.0001 | 0.61 | 0.02 |
Clumping ratea | |||
Ironb | 0.0037 | 0.68 | 0.04 |
a The correlation is significant only for the flooded and partially flooded plots.
b As concerns iron, the reported data refer only to the flooded and partially flooded plots, since the correlation is not significant for the dry ones.
As concerns the interstitial water chemical data, only those parameters that show significant correlations are listed in Table 4. Manganese, Br−, NH4+ and HCO3− are negatively correlated with the reed stem diameters, while Co and pH show a positive correlation with this trait. The clumping habit is more pronounced when high concentrations of Rb occur, while the stem density is negatively correlated with the contents of Ni. The rate of dead buds positively correlates with the contents of barium, and NO3− is negatively linked to the flowering rate (Table 4).
Significant relationships between reed traits and interstitial water chemical parameters (ions and metals), analysed by linear regressions (Spearman's rank correlation coefficient), with reed traits as dependent variables and the chemical parameters as individual explanatory variables (n = 8).
Slope | r 2 | P | |
Reed stem diameter | |||
Cobalt | 7.2233 | 0.84 | 0.03 |
Manganese | −0.0953 | 0.81 | 0.04 |
Bromine | −18.1543 | 0.88 | 0.02 |
NH4+ | −19.2896 | 0.89 | 0.02 |
HCO3− | −2.5911 | 0.98 | 0.00 |
pH | 8.2989 | 0.82 | 0.03 |
Clumping rate | |||
Rubidium | 100.6746 | 0.82 | 0.03 |
Stem density | |||
Nickel | −49.4255 | 0.94 | 0.01 |
Dead buds rate | |||
Barium | 0.4084 | 0.86 | 0.02 |
Flowering rate | |||
NO3− | −19.3345 | 0.88 | 0.02 |
On the basis of dissimilarities in macromorphological traits, PCA ordination (Fig. 3) agreed with the univariate analysis results. The plot distribution along the first axis, explaining 86.1% of the total variance, exhibits a gradient from tall/thick (on the negative side of the axis) to short/narrow reed stem height and diameter (on the positive side) and from low to high percentage of clumped stems. This biological gradient corresponds to an increase in the content of heavy metals, as emerged from axis 1, where positive correlations with the great majority of the chemical parameters measured in the sediments are observed. It is worth to mention the highly significant correlation with Cu and As (Table 5). There is also a gradient of increasing water depth, which corresponds to a rather distinct separation between the D and F plots within the space of the PCA on the first ordination axis (Fig. 3). The second axis, explaining only 7.4% of the total variance, separates stands with different percentage in dead buds (Fig. 3) and can be considered as a gradient of decrease in dead buds rate and zinc and increase in lead, as suggested by the statistically significant correlation with axis 2 (Table 5).
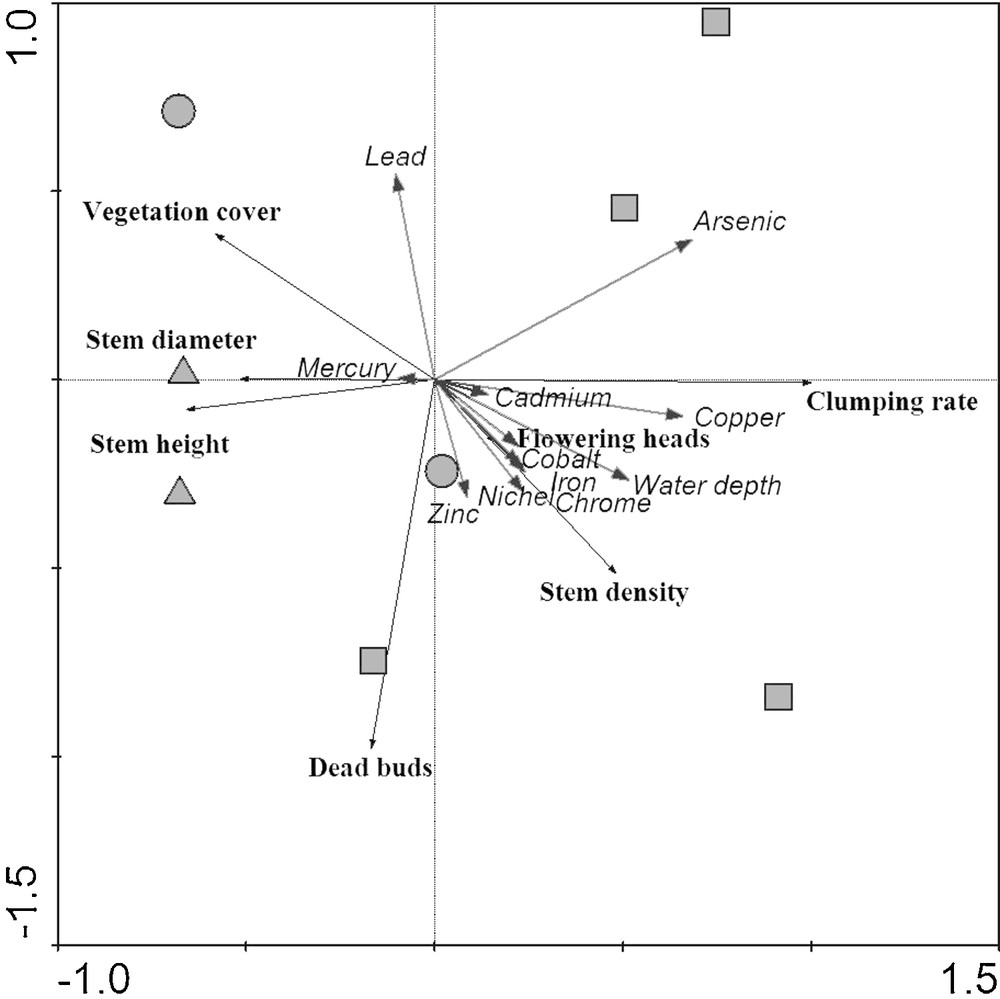
Principal Component Analysis ordination diagram of macromorphological parameters (in bold) with sediment chemical and environmental features superimposed. Symbols refer to reed stand types: squares: permanently dry (D); circles: partially flooded (M); triangles: permanently flooded (F).
Pearson's correlations (r) among sediment chemical and environmental variables (n = 8) and first PCA ordination axes.
Axis 1 | Axis 2 | |
Copper | 0.6575** | 0.0603 |
Lead | −0.1134 | 0.5561** |
Zinc | 0.0680 | –0.3228* |
Nickel | 0.2501 | 0.0139 |
Chromium | 0.2372 | −0.0003 |
Cobalt | 0.2330 | 0.0661 |
Iron | 0.2252 | 0.0843 |
Cadmium | 0.1258 | −0.0564 |
Arsenic | 0.6963** | 0.0870 |
Mercury | −0.1247 | −0.0791 |
Water depth | 0.5223** | −0.2664 |
4 Discussion
Besides a dramatically remarkable surface reduction, the investigated reed population showed some traits, which can be clearly referred to the dieback syndrome. Especially a reduced size of the stems and a notably high clumping rate, one of the most symptomatic traits of this phenomenon [41,42], were clearly recognized. Together with the reed retreat, they indicate the occurrence of reed dieback at the Lake Chiusi. It is possible to point out some co-occurrences between the detected reed traits, ecological conditions of the stands and measured data. It appears that the reed stands show the most vigorous traits and absence of typical dye-back symptoms in the partially flooded group of plots (M). The highest presence of symptoms of decay, especially the clumping habit, affects the permanently flooded stands (F), in accordance with the results of analogous investigations carried out in Central Italy by Gigante et al. [54]. With reference to the data recorded at Lake Trasimeno, comparable values for stem diameters were recorded in both the healthy and declining stands at Chiusi. Concerning the stem height, the plots located on the partially flooded floating mats at Chiusi show higher values, indicating that these peculiar floating growth forms of the rhizomes, presently disappeared at Lake Trasimeno, allow an increased stem growth.
Stem density at Lake Chiusi is rather high compared to other reed beds. According to 17 case studies in North America, reed density was ranging between 13–125 culms m−2 [25,38]. Higher maximum densities, about 200 culms m−2, were reported for natural reed beds in northern Poland [92]. However, stem density might be a quite controversial trait in detecting dieback. Data from the literature report that high biomass values can be reached also in reed stands with low stem density [93]. This suggests that low values of stem density are not necessarily a good detective trait for reed dieback. Indeed in the study area, as already noticed in other sites [28,56], stem density is higher in the declining reed stands, where the clumping rate is also higher, due to an increased number of secondary, thin culms produced after the death of the apical bud, representing another symptom of reed dieback [94,95]. Furthermore, the highest stem density values correspond to the lowest values of the total vegetation cover, indicating a generally scarce epigeal biomass production and showing that stem density cannot be used as a proxy for reed healthy growth.
Both high clumping rates and low cover values were recorded in the permanently flooded stands. Although P. australis is generally considered as a flood-tolerant plant [96–98], prolonged flooding, especially when occurring early in the growing season, has indeed been frequently reported as affecting its growth [47,99–105] and even suggested as a tool to control its invasion [106,107]. On the other side, seasonally fluctuating water levels are indicated as being well tolerated by reed [108,109].
It has often been stressed that artificially induced high water level variation may seriously affect natural vegetation in the littoral zone [110–113]. Significantly lower cover values were indicated for reed in diked wetlands with stabilized water levels and abundant organic matter [114]. Furthermore, phytotoxicity increases during litter decomposition processes, especially in anaerobic conditions, such as under stagnant and reducing conditions, under permanent flooding [115,116]. The allelopathic effect should also be taken into account as a possible component of reed decline, further enhanced by prolonged permanence of water and anaerobic conditions [27,117,118].
At the Lake Chiusi, all the declining sites appeared covered by water at the end of August (water depth around 37 cm on average), corresponding to the driest season and the period of minimum water level of the year. Considering that, according to the data provided by the Hydraulic Office of Arezzo Province, there is no evidence of remarkable changes or trends in the water regime in the last four decades, we may presume that the water column never disappears in the declining stands, keeping the bottom sediments constantly flooded and promoting anaerobic conditions. Indeed, the remarkable retreat and surface loss of the reed bed at Chiusi took place for the vast majority at the waterside. This may suggest a relation of co-occurrence between permanent flooding and reed decline at Lake Chiusi. Similar studies at Lake Trasimeno showed analogous results, reporting the heaviest condition of decline and retreat in areas permanently flooded since 2–3 decades [54].
Considering the chemical parameters measured in the interstitial waters and sediments, it should be stressed that several factors can influence the effective heavy metals plant bioavailability, such as pH, cation exchange capacity, organic matter contents [119,120]; however, metal concentrations in soils are generally seen as the dominant factor [120–122].
Several studies indicate a peculiar tolerance of P. australis towards a number of heavy metals [5,123–126]. In the present study, all the detected metals resulted less abundant in the temporarily flooded stands (M), apart from lead and mercury, while in groups F and D they are more or less comparable. It has already been reported that the degree of reed cover and its health condition influence the chemical features of the water [127]. At Lake Chiusi, reed-reduced growth seems to co-occur with higher amounts of copper, zinc, nickel, total chromium, cobalt, and iron, the latter being more abundant in the stands affected by clumping, although the concentrations of these metals are far from their threshold values of toxicity [128]. Moreover, as reported by many authors, P. australis can tolerate high concentrations of several heavy metals, acting as a bioaccumulator [126,128,129]. In this frame, the detected correlations between some heavy metals and reed-reduced growth might be accidental. On the other side, Weisner [50] indicated that the presence of ferrous iron in the sediment might inhibit growth in wetland plant species. Armstrong and Armstrong [115] suggested that with reduced aeration around the underground organs, e.g., with raised water levels, reed may be overwhelmed by the intake of iron.
Regarding N-bearing species, such as NO3−, in interstitial waters, it is reported that reed mechanical stability is negatively influenced by high concentrations of this ion [130,131], and that reed stands well supplied with nitrogen show high shoot loss [132]. However, the detected values in all the plots (2.26 ± 0.65 mg·L−1) are far below the values indicated as upper limit for an optimal growth of reed (5.0 mg·L−1) [133,134]. It should be noted, however, that Boar and Crook [135] suggest a possible correlation between NO3− in water (concentration > 2 mg·L−1) and decline of reed-swamps.
With respect to other chemical parameters in the interstitial waters manganese, bromine, NH4+ and HCO3− are also related to reed-reduced growth, while the clumped habit is more pronounced with higher values of rubidium (Table 4). As far as NH4+ is concerned, our results match those obtained in the frame of other investigations, which reported that the measured rate of dissimilatory nitrate reduction to ammonium (DNRA) was largely higher in sediments from declining than from healthy reed stands [136]. Reed decline, by inducing a lack of oxygen supplied via convection, might increase the DNRA acting to increase N availability in the sediments [25]: the rate of nitrate reduced to ammonium will be higher at declining sites [136].
5 Conclusions
The multidisciplinary approach presented in this study indicates that clear symptoms of reed bed decline occur at Lake Chiusi. These symptoms match the typical traits of the dieback syndrome. This suggests that:
- • the distribution area of this phenomenon should be extended to other sites in Central Italy, where the only known affected area is presently represented by Lake Trasimeno;
- • P. australis populations might be affected by decline on the large scale also in the Mediterranean basin, underlining the importance of detailed and widespread studies in this area.
To the best of our knowledge, such a combined analysis of interstitial water and sediment chemical parameters in correlation with reed decline traits is the first one to be carried out in a Mediterranean lake.
Only few of the considered ecological and chemical features seem to play a clear role in reed dieback; in particular, long-lasting flooding is always occurring in the declining stands. Dissolved species (main and trace components) in interstitial waters and heavy metals in sediments do not show any anomalous values and the observed correlations are still too weak to point out any clear pattern.
Compared with other studies, our work confirms that reed decline stands as a complex, multi-factor process whose causes are still not clearly understood. However, the correlations emerging in our investigation suggest that the co-occurrence of some chemicals (e.g., heavy metals) and stressing conditions (e.g., permanent flooding) may affect the growth even of an efficient metal accumulator, as reed is generally considered.
On the basis of the current state of knowledge, no appropriate action to contrast the further spread of this harmful phenomenon for species and habitats can be suggested. However, the present study represents a first step ahead to assess a robust explanation for serious threats to wetland conservation in the Mediterranean basin and to comprehend the real cause–effect processes underpinning the reed dieback.
Acknowledgements
We wish to thank Mario Meloni, from ‘Vite d’acqua’ Fishermen's Cooperative, for his kind availability to carry the study group by his boat and for the useful information provided about the ecosystem modifications he observed at Lake Chiusi in the last two decades. We also thank Franco Boschi, from the Hydraulic Office (Soil protection Service) of Arezzo Province, who kindly provided data about the water level of Lake Chiusi. A special thank is addressed to Matteo Nocentini for his help during water analyses.