1 Context and goals
The exploration of the structure of organs has remained descriptive for a long time. At the cellular scale, histology has allowed for a large amount of information about tissues structure, mainly achieved by the use of specific staining procedures. Nevertheless, the need for tissue modeling is in constant growth and requires a volume three-dimensional (3D) vision of the tissue structure at various scales. Histological techniques are not the most appropriate for this. Indeed, they associate a high in plane spatial resolution with a subsequent thickness of the slices, which makes the use of the obtained image series difficult for 3D modeling. Besides, the cutting of the sample achieved to build the slices can deform the geometries, as shown in the works of Verbeek et al. [1] and of Dauguet et al. [2,3]. These deformations are incompatible with modeling since the model has to be as close as possible to the realistic geometries. Therefore, using a technique, which avoids cutting the sample for its structure observation, is mandatory.
Modeling the behavior of tissues does not only require information about the global structure of organs, but is also demanding for data about its microscopic constitution. In particular, it is usually accepted that collagen is the constituent which influences at most the mechanical behavior of living tissues as noted by Holzapfel and Ogden [4]. For modeling purposes, investigating the structure of the collagen network within tissues is therefore essential. In a recent work by our research group [5], the collagen network structure of cerebral bridging veins was investigated using histological slices. This work showed that the geometry of the vein was complex and not straight, which led to the impossibility of determining fiber orientations inside the vein wall on the prepared bi-dimensional slices. A 3D observation technique allowing the visualization of collagen fibers could give an answer to these issues encountered using histological slices.
Experimental techniques providing volume 3D images of the collagen structure of tissues do already exist in the literature. For example, we note the multiphoton fluorescence microscopy techniques [6–8], which have the advantage to avoid any preparation of the sample for the observation of collagen due to its autofluorescence. Another technique allowing such observations is second harmonic imaging microscopy [9–11]. Both of these techniques allow imaging the collagen network at the fiber scale (around one micron in resolution), but are restricted to small three-dimensional fields of view (at the most 500 × 500 × 200 μm3), which do not allow for a global view of the organ geometry.
In the current work, we propose to use X-ray micro-CT (X-ray microtomography) for the observations of soft tissues. Such 3D observations have already been proposed in the literature by Metscher et al. [12–14], Johnson et al. [15], Wirkner and Prendini [16] but these studies focused on larger scales and on different applications. Pauwels et al. [17], Aslanidi et al. [18–20], Jeffery et al. [21] and Mizutani et al. [22] used high-Z element staining to investigate the structure of soft tissues at larger scales compared to those addressed by the present observations. Our work aims at the investigation of the collagen structure at the collagen fiber scale. Besides, it considers a piece of porcine vascular wall whereas most previous works focused rather on bigger organs or organisms.
Our technique, based on micro-CT, provides an isotropic 3D volume observation of the collagen structure in biological tissues. This technique focuses on a scale which is larger than those addressed by multiphoton fluorescence imaging and second harmonic imaging microscopy, but smaller than those previously studied using micro-CT. It does allow for fields of view of around 1500 × 1500 × 1500 μm3, which enable a global overview of the tissue geometry. Therefore, our new observations technique fills a gap in the range of various techniques allowing volume 3D observations of soft tissues at multiple scales. Besides, thanks to the cone-beam geometry, the micro-CT imaging device provides images with isotropic voxels. This allows cutting the observed volume at a post-processing step without any deformation or interpolation computation. This point constitutes a real advantage in comparison to other imaging techniques. By keeping an intact sample, it avoids any deformation, which could be induced by a slicing procedure. The minimum voxel size of around one micron allows for the observation of collagen fibers.
Imaging the structure of tissues requires some contrast within the tissue. In the present work, we first checked if there was any native X-rays contrast between collagen and the other surrounding constituents of the vascular wall. We then used and compared three different stains through a preliminary observation using a preclinical micro-CT system. Even if the minimum voxel size of 25 μm reached by this system is unsuited to the observation of collagen fibers, it allows for a fast selection of relevant staining procedures. The most relevant staining procedure was then used to provide a detailed observation of the structure of the collagen network within a porcine vein wall sample.
2 Rationale
X-ray images contrast is based on a difference of attenuation within the several constituents of the tissue of interest. The attenuation law of an electromagnetic wave is exponential. Its expression is given, locally (for a limited area corresponding to a pixel of the detector for example), by:
The contrast is generated by the differences of the attenuation coefficient μ from one point to another. This coefficient has been showed to depend principally on three parameters: the mean value of the atomic numbers of the target atoms, the energy of the incident photons (linked to their wavelength), and the density of the constituents of the tissue (also linked to their atomic numbers).
In case of soft tissues, the atoms composing the material have atomic numbers (Z), which are close from one to each other (the elements characteristics are given by Hubell and Seltzer [23]). Indeed soft tissues are mainly composed of hydrogen (Z = 1), carbon (Z = 6), oxygen (Z = 8) and nitrogen (Z = 7). Therefore, it is unlikely that a native difference of atomic number between the constituents can generate a sufficient contrast within the tissue.
In literature, some disparities exist between the density values commonly given for collagen and for the other constituents of vein walls. Densities ranging from 0.76 to 2.68 g/cm3 (average of 1.6 g/cm3) have been measured by Hashemi et al. [24] for collagen fibers. Similar values have been determined in the works of Hellmlich and Ulm [25], and Holmes and Kadler [26]. The density of the other constituents is closer to the one of water as noted by Anber et al. [27] and by Lillie and Gosline [28]. This difference of density from one constituent to another may generate a sufficient contrast between collagen fibers and the surrounding media when observed using X-rays. If it is not the case, contrast stains could be added to the sample to generate a sufficient contrast.
To investigate this point, different staining procedures were used in the present study. First, an unstained specimen was considered. This sample is used as reference. Then we used three different stains, based on those proposed by Metscher et al. [12–14] for the observation of small animals. These three stains are phosphotungstic acid (PTA), phosphomolybdic acid (PMA) and iodine potassium iodide (Lugol). We note that PTA and/or PMA intervene in the preparation of the Masson's trichrome solution classically used for the staining of collagen in histological slices. This denotes that these substances bind to the collagenous component of the soft tissue. This affirmation has also been proven in different works from the literature, for example in the works of Nemetschel et al. [29], Puchtler and Isler [30] or Watson [31]. Interestingly, these substances could also slightly color the elastin component of the tissue, as noted by Greenlee et al. [32].
Based on these considerations, we propose in the following an analysis of different sample preparations for the observation of the collagenous structure of the vein walls using micro-CT.
3 Methods
3.1 Micro-CT imaging systems
For the preliminary observation aiming at a selection of the relevant staining procedures, a pre-clinical X-ray micro-CT system (eXplore CT 120, GE Healthcare, Waukesha, USA) was used. This system can reach a minimum voxel size of 25 × 25 × 25 μm3.
For the detailed observation of a stained vein wall sample, a commercial system (Phoenix Nanotom s, GE Measurement and Control, Wunstorf, Germany) was used. This system can reach a minimum voxel size of 0.3 × 0.3 × 0.3 μm3, but its physical resolution is about 1 μm. We will present, in the second part of this paper, the results of an observation dealing with the maximum capabilities of this micro-CT system.
The acquisition parameters for both of these observations are summarized in Table 1.
Micro-CT systems and acquisition parameters.
Preliminary observation | Detailed observation | |
X-ray Micro-CT system | GE Healthcare eXplore CT 120 | GE Measurements and Control, Phoenix Nanotom |
Considered field of view | 10 × 10 × 10 mm3 | 1.3 × 1.3 × 1 mm3 |
Current | 32 mA | 260 μA |
Voltage | 80 kV | 100 kV |
Exposure time | 100 ms | 1000 ms |
Averaging | 1 | 3 |
Number of projections | 1200 | 1440 |
Reconstructed voxel size | 25 × 25 × 25 μm3 | 1 × 1 × 1 μm3 |
3.2 Contrast stains and sample preparation
The proposed observations focus on the structure of vein walls. For a reason of ease of obtaining and of manipulation, we considered porcine iliac veins of around 6 mm in diameter. These samples were taken directly at a slaughterhouse. They were put in warm uncontrolled water during transportation (at an initial temperature of 37 °C, decreasing until ambient temperature during transportation) to keep the sample hydrated. The surrounding conjunctive tissue was dissected within one hour after extraction. The samples were then fixed in a 10% formalin solution for one night and the different staining procedures were applied.
These staining procedures as well as the preparation of the staining solutions are detailed in Table 2. We note that PTA and PMA stains require the sample's dehydration, which is not the case of the Lugol stain [12–14]. The staining times were shown not to be critical as far as these times are sufficient for the staining solution to penetrate in the whole tissue. Nevertheless it has already been shown that the Lugol stain diffuses in most specimens in a few hours [12,17] whereas PTA and PMA include larger molecules, which need more time to penetrate into the tissue [17,33–36]. Our considered vein samples were small and thin (thickness of less than 1 mm). Taking into account the observations made by Pauwels et al. [17], who showed that PTA penetrates over a distance of 2.08 mm within the specimen for a staining duration of 24 h, we consider that stain penetration over duration of one night was sufficient for our samples.
Samples preparation and staining procedures.
Contrast stain | PTA (phosphotungstic acid) | PMA (phosphomolybdic acid) | Lugol (iodine potassium iodide) | No staining (reference sample) |
Fixation solution | Formalin | Formalin | Formalin | Formalin |
Storage until staining | Progressive dehydration until 70% alcohol | Progressive dehydration until 70% alcohol | Water | Progressive dehydration until 70% alcohol. Kept into alcohol until observation |
Preparation of the stain solution | Mix 30% of 1% phosphotungstic acid with 70% absolute ethanol | Mix 30% of 1% phosphomolybdic acid with 70% absolute ethanol | 1% Iodine metal + 2% potassium iodide + 97 mL of water. Dilute to 10% before use |
– |
Staining procedure | 70% alcohol Staining during one night Wash in 70% alcohol Conservation and observation in 70% alcohol |
70% alcohol Staining during one night Wash in 70% alcohol Conservation and observation in 70% alcohol |
Water Staining during one night Wash in water Progressive dehydration in ethanol Conservation and observation in 70% alcohol |
– |
Two vein samples extracted from different pigs were considered for a preliminary observation, leading to a comparison of three staining solutions (each vein was cut into four parts stained separately). The two samples associated with PTA staining were then cut and considered for the detailed observation.
3.3 Samples mounting
All of the samples were observed in an aqueous media composed of 70% alcohol. For the preliminary observation, 5 mm long portions of stained vein walls were inserted into Microtainer tubes of 8 mm in diameter (Becton, Dickinson and Company, USA) and then placed into the pre-clinical micro-CT system.
For the detailed observation, the need to reach a voxel size of around one cubic micron imposed reducing the sample size (see Fig. 1a). To achieve this, pieces of vein walls were cut lengthwise using a thin sharp blade. These specimens, composed of a 5-mm-long strip of vein wall, were inserted into a laboratory-made tube of 2 mm in diameter stuck on a steel rod using epoxy resin. This preparation is represented in Fig. 1b and is close to the one proposed by Mizutani et al. [22].
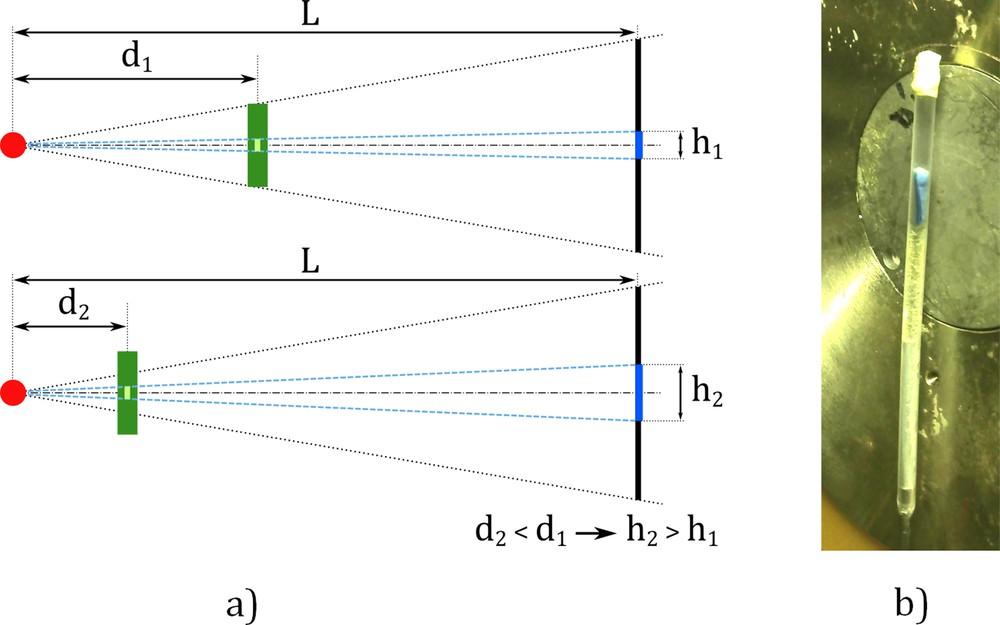
(Color online) (a) Influence of the distance between the source and sample on the physical voxel size. Reducing the voxel size imposes to move the sample closer to the source: d2 < d1 leads to h2 > h1. Since the sample must rotate during acquisition, its size (or diameter) is limited. (b) Mounting arrangement of the tubes including the samples within the micro-CT device, and correspondence with (a). Detail of the tube of 2 mm in diameter for the detailed observation. The sample is positioned by gravity at the bottom of the tube.
4 Results and discussion
First, a preliminary observation with a voxel size of 25 × 25 × 25 μm3 using a pre-clinical micro-CT system was conducted. This observation provides a global view of the considered vein preparations. The results are shown in Fig. 2.

Comparison of the different proposed staining procedures. The voxel size for these observations is 25 μm (cubic voxels). For each sample, a circumferential (left image) and a longitudinal cut (right image) of the vessel are given. (a) Reference sample (unstained). (b) Lugol staining. (c) PMA staining. (d) PTA staining. The scale bars are 2 mm. The dotted lines deal with the grey level profiles showed in Fig. 3.
On the images given in Fig. 2a, we notice that the unstained reference sample does not show a strong contrast. The native difference of densities between the constituents of the tissue as well as with reference to the surrounding liquid are not strong enough to provide any useful contrast. Therefore some staining of the tissue is required. Fig. 2b shows a sample stained with Lugol's solution. On these images, we can distinguish two layers in the vein wall. These layers will be noticed again on the following images. PTA and PMA stained samples are shown respectively in Fig. 2c and d. The appearances of the images coming from these two stained samples are similar. Again, we notice two layers in the vein wall. These two layers deal with portions of the vessel wall, which have different collagen contents. They correspond with the media and adventitia composing most vessel walls. The intima layer is not visible since it is too thin. A thin layer of conjunctive tissue surrounding the vein can also be observed.
The contrasts obtained within the vein wall and with reference to each staining procedure are analyzed quantitatively in Fig. 3 and Table 3. These profiles are plotted along an abscissa corresponding to a radius of the vein considered in circumferential cut after application of a Gaussian filter (smoothing radius of 3, filter implemented in Microview software, GE Healthcare). We notice on this figure that the differences of grey levels are much higher in the case of PMA and PTA staining. Since PTA and PMA staining seem to lead to similar contrasts, we will only focus on the PTA stained sample in the following.
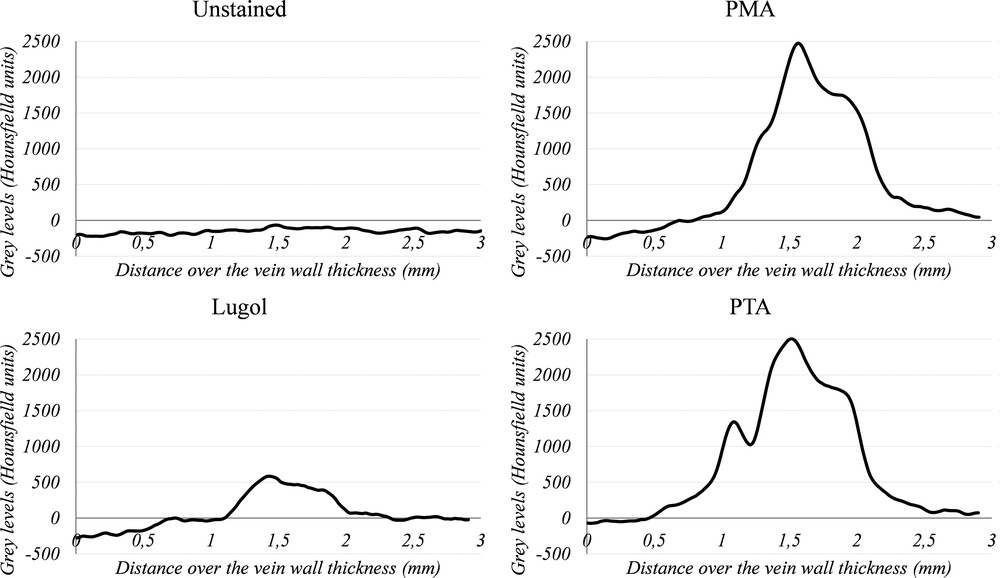
Grey level profiles (in Hounsfield units) along the thickness of the vein wall showing the contrast obtained with the different stains. The profiles are plotted after applying a Gaussian filter on the considered images to avoid the contribution of noise. The Hounsfield scale was obtained by a specific calibration procedure performed on the micro-CT. The lines defining the profiles associated with these curves are showed in Fig. 2 (0 mm deals with a point outside the vessel and 3 mm deals with a point inside the lumen of the vein).
Attenuation coefficient difference between two structures in Hounsfield units.
Values in HU | Unstained | Lugol | PMA | PTA |
Media vs. background | 61 | 411 | 1753 | 1840 |
Adventice vs. background | 61 | 502 | 2299 | 2452 |
Adventice vs. media | 0 | 91 | 546 | 613 |
Fig. 4 shows a detailed observation of a sample stained with PTA. The voxel size for the observed volume is of 1 × 1 × 1 μm3. In this figure, a three-dimensional visualization of the vein wall sample is given. Three orthogonal slices associated in different planes are also proposed. The medial and adventitial layers, previously detected in Fig. 2b, c, d, are clearly visible. A thin piece of conjunctive tissue, which has not been removed during the vein dissection, surrounds the vein wall.

Different views of a small sample of PTA stained vein wall. This sample is included in a tube of 2 mm in diameter. We notice clearly fiber orientations in the different views. 1. Lumen of the vein. 2. Media. 3. Adventitia. 4. Vasa vasorum. 5. Surrounding conjunctive tissue. The observed volume has approximately 1300 × 1300 × 1000 μm3.
Small black dots are also visible in the adventitial layer of the vein wall, which correspond to the vasa vasorum. This vasa vasorum has already been observed at a larger scale by the research team of Ritman et al. [37–39]. It constitutes a network of small vessels that supplies the walls of larger blood vessels. The geometry of the vasa vasorum network was extracted and is shown in Fig. 5.

(a) Highlighting of the vasa vasorum in the vein wall. This cut is inclined with reference to the vein axis. The two layers composing the vein wall could be distinguished and the vasa vasorum is only seen in the adventitial layer. The numbers deal with those defined in Fig. 4. The scale bar is 500 μm. (b) Three-dimensional geometry of the vasa vasorum network extracted from the images of the PTA stained sample.
The voxel size of one cubic micron allows for the observation of small collagen fibers, which are mainly visible in the adventitia in the X–Z view. We show in Fig. 6a a tangential slice that uses a Minimum Intensity Projection (MinIP) over 40 images (corresponding to images over a thickness of 40 μm), which shows the vasa vasorum. MinIP projection consists of a projection of a volume of interest to generate a bidimensional image, where the projections of the voxels keep only the lowest attenuation value on every view. In Fig. 6c, which is another tangential slice, we notice that the medial layer includes collagen fibers, which are oriented circumferentially whereas these fibers are longitudinally arranged in the adventitia. Nevertheless, these orientations can correspond to helically arranged collagen fibers with respectively small and high (close to 90°) helix angles. The orientations of collagen fibers resulting from this observation are shown in Fig. 7. The obtained fiber orientations are in accordance with observations, performed by other research teams on different vessels, for example by Watts et al. [40] on rats aortas and vena cava.

(Color online.) (a) MinIP projection of 40 tangential slices at different locations in the vein wall. Tangential cut of the adventitial layer showing the vasa vasorum structure. (b) Localization of the two slices of Figs. (a) and (c). Tangential cut of the medial layer of the vein wall. Collagen fiber circumferential orientations are visible. This slice shows also the structure of the adventitial layer corresponding to rather longitudinal collagen fibers. The numbers deal with those defined in Fig. 4.

Schematic representation of the observed portion of iliac vein wall. The collagen fibers embedded within the specimen stained with PTA are represented with circumferential orientations in the medial layer and longitudinal orientations in the adventitia. A piece of conjunctive tissue surrounds the vein wall.
By comparing the average grey levels in the different layers of the vein wall (see Figs. 2, 4 and 6), it is possible to assess qualitatively the relative proportion of collagen in each layer. By this way, it is noticeable that the adventitia has a larger collagen density compared to the media. This observation is in accordance with the work of Holzapfel [41] focused on arterial walls.
Our study also allowed assuming the stability of the PTA staining. The same sample was observed several times within 10 months leading no significant loss of contrast on the obtained images. Therefore, we can affirm that the PTA staining remains stable. This observation is in accordance with the results of Pauwels et al. [17], which showed no contrast variation after 4 days of conservation of the sample in water.
We note that the Masson's trichrome staining procedure involves PMA/PTA. This allows observing a Masson's trichrome stained sample embedded in paraffin using micro-CT. However, in our experience, we noticed that the surrounding paraffin degrades the global contrast by adding on the images a uniform non-negligible grey-level all around the sample (data not shown).
In our work, we used the staining solutions previously used by Metscher et al. [12–14]. Our results showed as well that PTA and PMA staining were satisfactory in the case of vein walls, and for staining and observing the microscopic collagen network structure. Iodine potassium iodide (Lugol) staining led to good results in the works of Metscher et al. [12,13], even if the structures of the tissue were differently contrasted in comparison with PTA stained samples [12]. In our case, we showed that Lugol did not lead to a satisfactory contrast between the layers of the vessel wall. Therefore we can suppose that this solution does not stain collagen at most, but also stains other constituents of the tissue, like for example muscle fibers as showed by Jeffery et al. [21].
Pauwels et al. [17] obtained satisfactory contrast within the tissue using PTA and PMA staining. This observation is in accordance with our work, even if our study focuses on smaller scales. We note that Pauwels et al. [17] observed some slight precipitation at the surface of their samples. They were attributed to the high concentration of the staining solutions, which were used. In our work we used lower concentrations and did not observe these precipitations.
5 Conclusion
In the present work, a three-dimensional volume observation of the collagenous structure of a vascular soft tissue was achieved using micro-CT. We used fresh porcine iliac veins, which were stained with PTA, PMA and Lugol, which act as contrast agents, revealing the collagenous structures. The comparison of these three staining solutions showed that PTA and PMA lead to the best contrast within the tissue. Our work allowed the observation of the media and adventitia in the vein wall. The intima layer, which remains too thin, could not be observed. In the two observed layers of porcine iliac veins, collagen fibers were visible. They were shown to be circumferentially arranged in the media and to be longitudinally orientated in the adventitia. The vasa vasorum was also visible in the acquired images and the architecture of its network was extracted from these images. Our observation also allowed for a qualitative evaluation of the volume fraction of collagen from one part of the tissue to another.
Finally, the proposed observation technique and staining procedures allow imaging the collagen structure of the tissue with a voxel size of around one micron. One of the advantages of this technique relies in the fact that it does still allow for fields of view of several cubic millimeters and for isotropic voxels, which make post-processing easier. By this way, it is situated at a larger scale than multiphoton microscopy, but does still allow detailed three-dimensional observations of the structure of the tissue.
Disclosure of interest
The authors declare that they have no conflicts of interest concerning this article.
Acknowledgements
The authors would like to thank J.-M. Hiver and O. Ferry from the École des mines de Nancy as well as G. Fargier from the École centrale de Lyon (Equipex IVTV) for their help to the observation using the microtomographs. They would also thank A. Sayeh for her help using the preclinical micro-CT system.