1 Introduction
Over the past decades, diverse attempts have been made in order to fight plant diseases through the development of synthetic fungicides [1]. Thus, various synthetic chemicals have been used as antifungal agents to inhibit the growth of plant pathogenic fungi. However, the widespread use of these chemicals has several drawbacks, including handling hazards, pesticide residues, cost, and threats to human health and environment [2]. Moreover, the intensive use of these chemicals creates imbalances in the microbial community, which may be unfavourable to the activity of the beneficial organisms and may also lead to the development of resistant pathogen strains [3], increasing environmental degradation. Owing to the limitations of chemical control measures, it seems appropriate to seek a more suitable control method [4]. Biological control appears as the most promising strategy, environmentally safe and cost-effective method for controlling the agricultural phytopathogens [4–6]. Recently, there has been a growing interest in the research of the possible use of functional biomolecules for pest and disease control in agriculture. Indeed, enzymes are gaining importance and popularity in the industry as an environmentally friendly alternative to the traditional chemical treatments, especially when it becomes more cost-effective to produce.
Glucose oxidase (β-d-glucose: oxygen-oxidoreductase EC 1.1.3.4) is a flavoprotein belonging to the oxydo-reductase family. The GOD catalyses the oxidation of β-d-glucose into gluconic acid and hydrogen peroxide using molecular oxygen as an electron acceptor [7]. This enzyme has been produced by various fungal sources, mainly from the genus Aspergillus [8,9] and Penicillium [10,11]. GOD was widely used as a potent antibacterial and antifungal agent in the food industry [12–14]. It is capable of inhibiting the growth of microorganisms through naturally produced hydrogen peroxide and gluconic acid [15]. Thus, the H2O2 cytotoxicity and the lowering of pH by the production of d-gluconic acid may influence the growth of some microorganisms [16]. This enzyme exhibits a potential antagonistic effect against different food-borne pathogens like Staphylococcus aureus, Salmonella infantis, Clostridium perfringens, Bacillus cereus, and Listeria monocytogenes [17–19].
Although the wide-ranging antibacterial and antifungal GOD activity was reported more than 70 years ago, no study has been conducted so far, to the best of our knowledge, to evaluate the effect of glucose oxidase on agricultural phytopathogen strains and plant disease control. Accordingly, in this study, we aimed not only to isolate and identify a new fungus producing glucose oxidase, but also to investigate the antifungal effects of the partially purified glucose oxidase against Fusarium solani in vitro and in vivo on tomato plants. To develop a cost-effective and an environmentally friendly treatment system to control pests on plants, we aimed at improving the GOD production level using a statistical experimental design.
2 Materials and methods
2.1 Isolation and screening of fungi for glucose oxidase enzyme producing strain
More than 75 different fungi strains were isolated from a variety of contaminated and decaying cereal samples. The samples were homogenized in sterile distilled water and microbes were isolated using spread plate and streaking methods on a Czapek medium [20]. The fungi were isolated from the plates and subcultured on potato dextrose agar (PDA). Subculturing was continued until pure isolates were obtained. The latter were screened for GOD enzyme production. Initially, the isolates were incubated in a solid screening medium at 30 °C for 72 h. After the fungal growth, a solution containing 20 mM sodium phosphate buffer, pH 7.0, 0.1 M glucose, and 20 μg/mL of horseradish peroxidase (HRP) were added [21]. Then, the diameter of the brownish–red halos around the fungi (D) and the diameter of the fungal growth (d) were measured.
The fungi strains with major D/d ratios were selected for further studies. Selected strains were harvested from the plates, dislodged under aseptic conditions and then tested in the liquid medium.
2.2 Morphological and molecular identification of the selected fungi
The fungi strain was morphologically and physiologically characterized according to universally accepted keys for fungus identification [22,23]. The identification was confirmed by molecular methods [20]. For the latter, the K38 strain was cultivated in 100 mL of liquid medium at 30 °C under continuous agitation at 150 rpm. Mycelia from 48-h cultures were harvested and DNA was extracted.
The partial sequence of the fungal rDNA ITS gene was submitted to PCR amplification via forward primer ITSl (5′-TCCGTAGGTGAACCTGCG-3′) and reverse primer ITS4 (5′-TCCTCCGCTTATTGATATG-3′).
The PCR was initiated by incubating the reaction mixture at 94 °C for 3 min, followed by 35 cycles of 1 min at 94 °C. The reaction was annealed at 55 °C for 1 min and terminated with extension and final steps consisting of 1 min at 72 °C and 10 min at 72 °C. The PCR products were analysed on an agarose gel and purified by the PEG–NaCl method. The nucleotide sequences were determined using the Big-Dye Terminator v3.1 Cycle Sequencing Kit and the automated ABI Prism1 3100-Avant Genetic Analyser (Applied Biosystems). The sequence obtained for the intergenic region rRNA ITS was deposited at the GenBank in NCBI under accession number KM594388.
Fungi were identified by searching the homology of ITS genes sequences of the isolate with the sequences using BLAST search algorithm.
2.3 Plant pathogenic fungi
The pathogen F. solani was isolated and then identified by the Olive Tree Institute of Sfax, as described by Hammami et al. [24] and kindly provided by Dr. Mohamed Ali Triki. The fungus was isolated from tomato plants exhibiting symptoms of tomato damping-off. The isolate was maintained on PDA slants, grown at 25 °C and then stored at 4 °C in tubes containing PDA at −20 °C in a tryptone salt medium.
A conidial suspension of F. solani strain was prepared by culturing the fungus on PDA medium until sporulation for one week at 25 °C. The agar surface was then rinsed with 10 mL of distilled water containing 8.5 g/L of NaCl and 1 mL/L of Tween 80. The concentration of spores was determined using a Malassez cytometer (Dutscher 140501), adjusted to 1.0 × 108 spores/mL and used to infect tomato roots.
2.4 Media
Five different media were used in the current work: Czapek medium, solid screening medium, liquid medium, PDA and Tryptone salt medium. The use and composition of these media were as follows:
- • Czapek medium generally allows the isolation and differentiation of Aspergillus genus [20]; it contains 2 g/L of NaNO3, 1 g/L of KH2PO4, 0.5 g/L of MgSO4·7H2O, 0.5 g/L of KCl, 0.01 g/L of FeSO4·7H2O, 30 g/L of sucrose, and 20 g/L of agar-agar. The pH was adjusted to 5.0 and the medium was supplemented with chloramphenicol (0.1 g/L) to inhibit bacterial growth;
- • the solid screening medium used for the preliminary selection of GOD producing strains, contains 10 g/L of glucose, 6 g/L of NaNO3, 0.5 g/L of KCl, 0.5 g/L of MgSO4·7H2O, 1.5 g/L of KH2PO4 with traces of CuSO4, ZnSO4, MnCl2 and FeSO4, 20 g of agar and 2.5 mM o-dianisidine [21];
- • the liquid medium used for GOD production contains 10 g/L of glucose, 1 g/L of peptone from casein, 5 g/L of yeast extract, 6 g/L of NaNO3, 0.5 g/L of KCl, 0.5 g/L of MgSO4·7H2O, 1.5 g/L of KH2PO4 with traces of CuSO4, ZnSO4, MnCl2 and FeSO4 [21];
- • Potato Dextrose Agar (PDA, Oxoid, Johannesburg) was used for pre-growing the new isolated fungi GOD production and the pathogen F. solani [24];
- • the tryptone salt medium utilized for the storage of the pathogen F. solani contains 1 g/L of tryptone, 8.5 g/L of NaCl, 1% of Tween 20, and 15% of glycerol [24].
2.5 Culture growth
The inoculums were prepared by transferring the spores into 50 mL of a liquid medium contained in a 250-mL conical flask. The sterilized Erlenmeyer flask was kept on a rotary shaker at 150 rpm at 30 °C for 24 h in order to obtain homogenous spore suspensions.
GOD production was carried out by inoculating the liquid medium with 4% (v/v) of the inoculums preparation. The pH was adjusted to 6.5 using 0.1 N NaCl. The flasks were then incubated at 30 °C and 150 rpm in an orbital shaker. After 48 h of fermentation, the cultures were centrifuged for 15 min at 8000 g and 4 °C. The clear supernatant was used to determine enzyme activity.
2.6 Aspergillus tubingensis CTM 507 GOD preparation
Following 32 h of cultivation in the optimized medium (sucrose 20 g/L, yeast extract 4 g/L, corn steep liquor 2 g/L, peptone 2 g/L, KCl 0.5 g/L, MgSO4·7H2O 0.5 g/L, KH2PO4 1.5 g/L with traces of CuSO4, ZnSO4, MnCl2 and FeSO4), the extracellular GOD enzyme was separated from the culture medium by filtration and centrifugation. The enzyme preparation was then partially purified by heat treatment (10 min at 50 °C) and 70% ammonium sulfate fractionation steps.
2.7 Effect of GOD on mycelial growth
To study the activity of A. tubingensis CTM 507 GOD against F. solani mycelia growth, GOD was incorporated into the fungal growth medium (PDA) at different concentrations from 5 AU to 125 AU during six days. The GOD samples were serially diluted twice and the reciprocal of the highest inhibitory dilution was used to calculate the arbitrary activity units (AU) per millilitres [25]. After mixing, the amended PDA was dispensed into 9-cm-diameter Petri dishes and allowed to cool. An agar plug of fungal inoculum (6 mm diameter) was removed from young pure culture of F. solani and placed upside down in the centre of the Petri dishes. The plates were incubated at 25 °C and the radial growth of mycelium was measured at 24-h intervals for a week. The plates without GOD were used as negative controls. The plates were used in triplicate for each treatment. The relative growth inhibition of the treatment compared to the negative control was calculated as a percentage, using the following formula:
2.8 Effect of GOD on spore germination
For spore germination assays, F. solani spore suspension (1.0 × 108 spores/mL) was inoculated with various concentrations of GOD (5 AU to 125 AU). For each concentration, 10-μL aliquots of spore suspension were placed on separate glass slides in triplicate. The slides containing the spores were incubated in a moisture chamber at 25 ± 2 °C for 4 h. Each slide was then fixed in lactophenol cotton blue and observed under the microscope for spore germination. The spores that had generated germ tubes were counted and the percentage of spore germination was calculated.
2.9 Microscopic observations
The morphological aspects of F. solani mycelia treated by GOD including fragmentation, vacuolization and lyses were microscopically examined.
2.10 Effect of GOD on disease development in vivo conditions
Biological tests were designed to measure the antifungal activity of GOD on tomato plants. Both curative and protective activities were evaluated on tomato artificially inoculated with F. solani.
For GOD protective activity, tomato plants were treated with GOD 24 hours before pathogen inoculation. For its curative activity, tomato plants were inoculated with 20 mL of fungal spore suspension (1.0 × 108 spores/mL) of F. solani. These plants were incubated for 24 h and then treated with different concentrations of GOD. The control plants were uniformly sprayed with sterile water or pathogen suspension used as negative or positive control groups of the experiments without using any antimicrobial. As a commercial fungicide, Hymexazol was used against a grey mould of tomato and evaluated in both protective and curative assays at the recommended concentration for comparison.
The area of lesions on treated plants was measured in millimetre using a Vernier calliper. All tests were conducted in three replicates. The antifungal efficacy of the test samples on the disease was evaluated after 12 days as a percentage of inhibition calculated by the formula:
2.11 Optimization of enzyme production
2.11.1 Influence of carbon source, nitrogen source, CaCO3, and pH
For a high production of GOD, we chose the best carbon source, nitrogen source, CaCO3 level and pH using the traditional method ‘one variable at a time’. The experiences were carried out in a 250-mL Erlenmeyer flask containing 50 mL of the production liquid medium. After sterilization, the flasks were inoculated with inoculums (4%, v/v) and maintained under different compositions: 10 g/L of the carbon source (glucose, galactose, sucrose, starch, maltose, mannose, molasses and lactose), 5 g/L of the nitrogen source (yeast extract, peptone, tryptone, Edamin, Hy case amino, corn steep liquor, urea, NH4SO4, KNO3 and NaNO3) and different CaCO3 concentrations (0, 10, 20, 30, 40 and 50 g/L). The most suitable pH of the fermentation medium was evaluated by adjusting the pH of the culture medium at various levels in the range from 4.0 to 8.0.
2.11.2 Screening of factors
The Plackett–Burman design was used to screen medium components and culture conditions affecting GOD production using the new isolated K38 fungus. This design was also employed to select the most important variables among a large number of factors. Hence, the effects of eight components at two levels, low (−1) and high (+1), including temperature, agitation, inoculums size, sucrose concentration, NaNO3, peptone, corn steep liquor (CLS) and yeast extract (YE) on GOD production were studied. The calculation of the effect of each variable was carried out by the determination of the contrast coefficient (b) obtained from the difference between the average of the measurements made at high (+) and the low (−) levels of the variable. The statistical significance of the factor effect was checked using a t-test (Table 1).
Estimation of the effect of significant independent variables on the GOD production of K38 strain using the Plackett–Burman design.
Code of variables | Variables | Levels | b | t-value | P > |t| | |
Low (−1) | High (+1) | |||||
X1 | Temperature (°C) | 30 | 37 | −8.61 | −2.64 | 0.03 |
X2 | Agitation (rpm) | 150 | 200 | −1.96 | −0.60 | 0.57 |
X3 | Inoculum size (%) | 2 | 4 | −8.16 | −2.50 | 0.04 |
X4 | Sucrose (g/L) | 10 | 20 | 3.97 | 1.22 | 0.26 |
X5 | NaNO3 (g/L) | 0 | 6 | −0.98 | −0.30 | 0.77 |
X6 | Yeast extract (g/L) | 0 | 6 | 7.09 | 2.18 | 0.06 |
X7 | Peptone (g/L) | 0 | 2 | 3.97 | 1.22 | 0.26 |
X8 | Corn steep liquor (g/L) | 0 | 2 | 5.66 | 1.74 | 0.12 |
2.11.3 Response surface methodology
Based on the results of the Plackett–Burman design, four variables [temperature (X1), inoculum size (X2), yeast extract (X3) and corn steep liquor (X4)] were found to have a greater influence on GOD production by the K38 isolate. Thereafter, the Central Composite Design (CCD) Response Surface Methodology was performed to determine the level of each of these factors giving the highest GOD production. These variables were prescribed into five levels, coded −2, −1, 0, 1 and 2.
Modelling was based on the second-order polynomial equation:
(1) |
The predicted response was calculated using the model involving only significant parameters. The insignificant model terms were eliminated using a step-by-step procedure.
2.12 Analytical methods
2.12.1 Enzyme activity
Glucose oxidase activity was assayed in a reaction mixture (3.1 mL) containing 100 μL of the enzyme, 1 mL of benzoquinone solution (0.1%) and 2 mL of a 1 M glucose solution prepared in a sodium citrate buffer (0.1 M, pH 5.0). The reaction mixture was incubated for 10 min at 35 °C. The hydroquinone liberated in the reaction mixture was measured according to the fast spectrometric method at 290 nm [26].
2.12.2 Dry cell weight determination
The samples were centrifuged at 8000 g for 20 min and the mycelia pellets recovered were washed with distilled water and dried at 105 °C to a constant mass in a drying oven. The biomass concentrations were expressed in dry cell weight per litre of culture medium.
2.13 Statistical analysis
The data were analysed using SPSS (Version 11.0.1 2001, LEAD Technologies, Inc., and USA) statistical software. The response surface was plotted using Microsoft Excel software (Version 2007, Microsoft Inc., USA). The two analytical steps, study of variance (ANOVA) and regression analysis were employed to establish the optimum levels of the GOD production parameters. Mean values among treatments were compared by Duncan's multiple range test at the 5% (P < 0.05) level of significance.
3 Results
3.1 Isolation and screening of fungi for the glucose oxidase enzyme producing strain
In order to find GOD producing fungi, a simple screening method in solid medium was followed. Seventy-five new fungi were isolated from various expired cereal product samples and screened for GOD production. Among these, seven efficient fungi strains, based on zone formation due to glucose converting, were selected for further studies.
GOD production in liquid medium by the seven selected strains showed that the K38 strain was the most potent isolate for enzyme production (31.25 U/mL). Consequently, this fungal strain was selected for subsequent studies.
3.2 Morphological and molecular identification of the fungus strain K38
Fungus strain K38 showed a mycelial growth with black sporulation on solid medium plate. The optic microscopy examination of this strain illustrated the presence of upright conidiophores that terminated in a swollen vesicle, with conidiospores on it. Therefore, this strain was identified as primarily Aspergillus genus.
The ITS sequence amplification of the K38 strain by PCR resulted in a product of 574 bp in size. The PCR-amplified ITS sequence showed 100% nucleotide identity with Aspergillus tubingensis HQ315841, a high nucleotide identity of 99% with A. tubingensis strain G2-22 GU723439 and A. tubingensis isolate GU134883. Therefore, the strain was identified as A. tubingensis. The sequence was deposited in GenBank in NCBI and the accession number of A. tubingensis CTM 507 was KM594388.
3.3 In vitro antifungal glucose oxidase activity
The antagonistic activity of a partial purified GOD against plant pathogenic fungi was evaluated by the disc diffusion method after 6 days of culture. The findings revealed that GOD exhibited a potential antifungal activity against the phytopathogenic fungus F. solani. The antifungal activity of the GOD was dose-dependent. Indeed, a gradual decrease of the fungi's diameter was observed by increasing the GOD concentration from 0 AU to 125 AU (Fig. 1). At a concentration of 5 AU, growth mycelia diameter was about 40.4 mm and was approximately 27.5 mm and 18 mm at a concentration of 50 AU and 70 AU, respectively.

(Colour online.) Antifungal activity of partial purified GOD towards F. solani: effect of increasing concentration on the antifungal potency after six days of incubation. A. Negative control (non-treated). B. Treated with 5 AU GOD. C. Treated with 40 AU GOD. D. Treated with 85 AU GOD. E. Treated with 125 AU GOD. F. Positive control (treated with Hym).
Fig. 2A presents the F. solani growth inhibition percentage at different GOD doses. The IC 50%, which represented the concentration that would inhibit growth at 50%, was about 27 AU of GOD. The minimum inhibitory concentration (MIC), defined as the lowest concentration of partial purified GOD that resulted in a complete growth inhibition of F. solani, was found to be 125 AU. Indeed, the GOD exhibited a pronounced antifungal activity on fungal spore production. As compared to the control, spore production of F. solani was strongly hampered in the presence of GOD. It was significantly decreased with increasing enzyme concentration within the range of 25% to 100% at a concentration ranging from 5 AU to 125 AU (Fig. 2B). At concentrations of 5 AU and 20 AU, spore production was 7.5 × 105 spores/mL and 5 × 105 spores/mL, respectively. It was inhibited at a concentration of 100 AU.
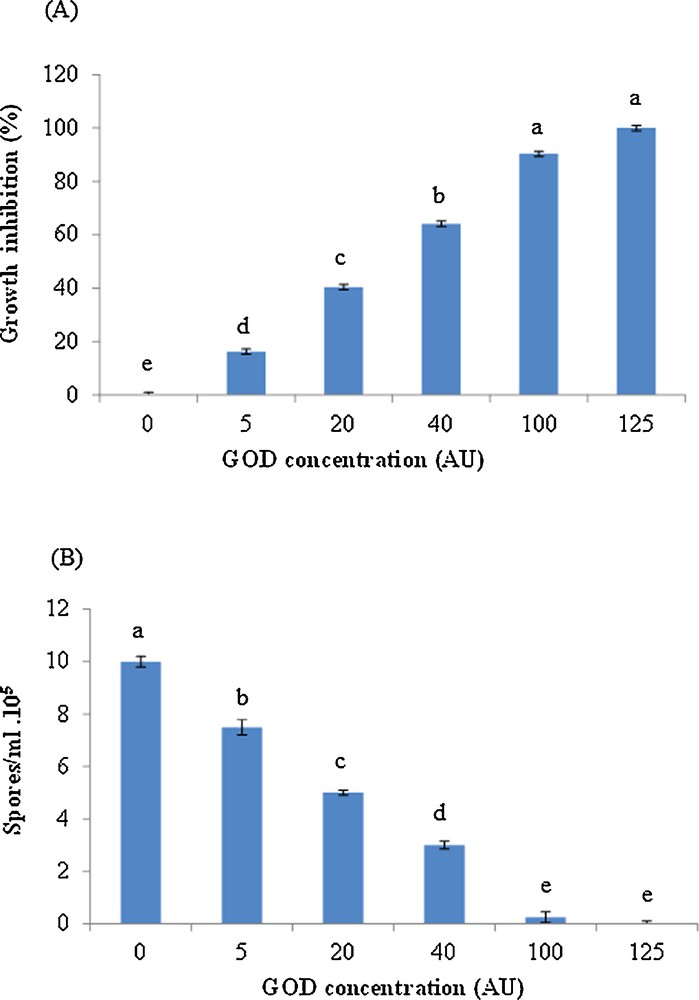
(Colour online.) Inhibitory potency of different concentration of GOD towards F. solani mycelium growth (A) and spore production (B). The different letters (a, b, c, d and e) indicate significant differences among treatments at the 5% level according to Duncan's test.
A microscopic study was conducted in order to determine the effects of GOD on the mycelial growth of F. solani. Compared to controls (Fig. 3A), a treated Fusarium mycelium showed strong lysis (Fig. 3B), cytoplasm vacuolization (Fig. 3C), early chlamydospores formation (Fig. 3D) and induction of mycelial cords via anastomosis between hyphal filaments (Fig. 3E). Microconidia are grouped in false heads (Fig. 3F).

(Colour online.) Microscopic examination of the mycelium grown on F. solani after four days of culture in a medium containing 100 AU of GOD. A. Negative control (without GOD). B. Lysis of fungal mycelia. C. Cytoplasm vacuolization. D. Chlamydospores formation. E. Mycelia cords formation. F. Microconidia grouped in false heads.
In order to determine the fungicidal or fungistatic nature of GOD against F. solani, the sample collected from fungal suspension in the presence of GOD (125 AU) was transferred into the PDA medium without GOD. The absence of growth of the fungus after 48 h indicated that the inhibition of fungal growth by GOD was fungicidal in nature.
3.4 In vivo antifungal glucose oxidase activity
In this study, the value of the antifungal activity of the partial purified GOD against F. solani was assayed, in vivo, on tomato root infestation. GOD was sprayed on the tomato leaves before (protective activity) and after (curative activity) fungal inoculation. The protective and curative effects of A. tubingensis CTM 507 GOD on the invasion and development of the phytopathogenic fungus F. solani has been performed by the biocontrol of the tomato root infestation (Fig. 4). The protective effect of GOD was evaluated after one week of inoculation. The tomato seedlings inoculated and untreated (control) manifested symptoms of leaf burning one week after inoculation. These symptoms progressed to necrosis in snares two weeks later. However, the plants inoculated and treated with GOD were similar to the healthy control plants. Indeed, no symptoms or necroses were observed at the time of assessment. However, after two week of inoculation, the inoculated and treated tomato plants with both positive control hymexazol and GOD showed both yellowing in the leaves and burning. These results indicated that treating tomato plants with GOD is effective in preventing the onset of symptoms on seedlings.

(Colour online.) Effect of GOD and of the hymexazol treatment on soft rot tomato root development occasioned by F. solani after 20 days of incubation at 30 °C; healthy control (Ts); inoculated control (Tm); preventive treatment by GOD (G + i); preventive treatment by hymexazol (H + i); curative treatment by GOD (i + G); curative treatment by Hymexazol (i + H).
Moreover, the curative F. solani invasion was significantly more effective than the protective method. Thus, a total inhibition (100%) of disease development was observed in the curative GOD treatment, which significantly affected plant development and increased biomass and root shoots length, without any evidence of symptoms of the disease.
Accordingly, pathogenicity results revealed different percentages of disease incidence on the tomato cultivars. The GOD treatment considerably reduced the disease incidence on the leaves when compared to the inoculated plants control (Fig. 5). The incidence was null in the curative treatment with GOD and hymexazol. In contrast, it was around 45% and 20%, respectively, for the preventive treatment with GOD and hymexazol. Therefore, the biological GOD caused a significant reduction in the disease incidence by up to 100%.
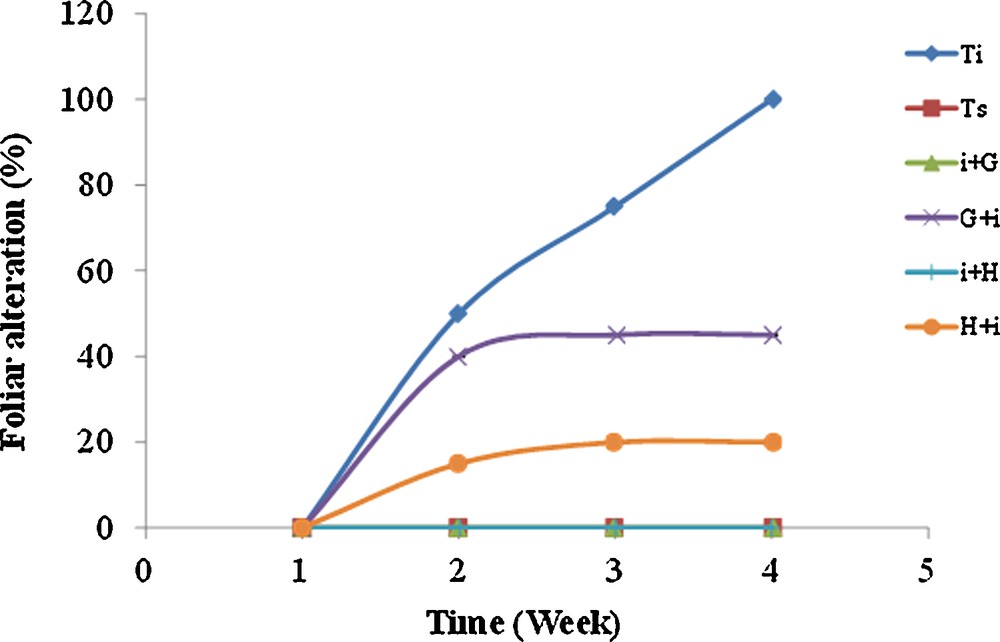
(Colour online.) Temporal evolution of foliar alteration occasioned by F. solani infestation; healthy control (–■–); inoculated control (–♦–); preventive treatment by GOD (×); preventive treatment by hymexazol (–●–); curative treatment by GOD (); curative treatment by hymexazol (+).
3.5 Optimization of GOD enzyme production
3.5.1 Effect of carbon sources
The influence of various carbon sources, including glucose, galactose, sucrose, starch, maltose, mannose, molasses and lactose, at a concentration of 10 g/L on the production of GOD by the new isolated strain A. tubingensis CTM 507 was checked. Indeed, significant GOD activity was observed (40.86 U/mL) using sucrose as a carbon source, followed by glucose (31.23 U/mL). Maltose and molasses, respectively, supported the production of GOD up to 20.33 U/mL and 15.27 U/mL, while the other carbon sources were found to be poor inducers for GOD production.
Otherwise, the production of GOD, in a medium containing sucrose, is significant after 24 h of incubation and it is repressed after 48 h of fermentation. The decline of the enzyme activity leads us to follow production every two hours (Fig. 6). The obtained results indicated that enzyme production increased with fermentation time from 4 h to 32 h, leading to a maximum of 43.73 U/mL. The decrease in activity after 32 h of incubation is probably related to the strain morphology and biomass growth. In addition, the biomass profile (Fig. 6) shows the presence of two phases of exponential growth. Thus, in the first exponential phase of growth, up to 30 h of fermentation, the mycelial organisms become small pellets with a diameter of approximately 2.2 mm. After 30 h (the second exponential phase of growth), the pellet diameter increases dramatically and reaches 4 mm, before the pellet is destroyed after 48 h (Fig. 7A, B and C).

Kinetic growth of K38 fungus (–●–) and GOD production (–■–).

(Colour online.) Fungal morphology for various fermentation durations: A. Pellet diameter up to 30 h. B. After 30 h. C. After 48 h of fermentation.
3.5.2 Effect of nitrogen sources
To investigate the effect of nitrogen sources on fungus growth and GOD production, different organic and inorganic nitrogen sources were tested (yeast extract, casein peptone, tryptone, Edamin, Hy case amino, corn steep liquor, urea, NH4SO4, KNO3 and NaNO3) with a C/N substrate ratio of 1.
The obtained results showed that corn steep liquor and sodium nitrate were the most suitable nitrogen sources for GOD production, with GOD activities of 15.55 U/mL and 19.73 U/mL, respectively. The other sources were beneficial to enzyme production and good inducers of fungus growth. Yeast extract (4.76 g/L) and casein peptone (4.96 g/L) gave the maximum biomass production. Consequently, corn steep liquor, NaNO3, yeast extract and casein peptone were selected to be screened in the subsequent study using the Plackett–Burman design.
3.5.3 Effect of pH
The initial medium pH is one of the most important environmental factors affecting mycelial growth and enzyme production. The K38 strain GOD production was studied at various pH ranging from 4.0 to 8.0 in a pre-optimized culture medium.
The highest GOD production of 44 U/mL was recorded using a medium with an initial pH of 6.5. The decrease in the initial pH of the medium from 6.5 to 4.0 showed a significant decline in GOD production. A loss of 66% in enzyme production was observed at the initial medium pH of 4.0. Also, the increase in the initial pH of the medium from 6.5 to 8.0 caused a slight decrease in GOD production.
3.5.4 Effect of CaCO3 supplementation
The effect of calcium carbonate, at various concentrations (0–50 g/L) on GOD production was studied in a pre-optimized culture medium and at pH 6.5.
The obtained results showed that GOD production was remarkably influenced by CaCO3. The maximum GOD level (57.07 U/mL) was obtained at 30 g/L CaCO3 and was about 1.3-fold higher than that in the absence of CaCO3. Over 30 g/L CaCO3, the enzyme production decreased. It was about 57.28% of the optimal level, at 50 g/L CaCO3.
3.5.5 Screening of GOD production main factors
In the first approach, the Plackett–Burman design was applied to determine the effect of various factors on GOD production. Eight different variables including some operational parameters and medium components were screened using 16 runs matrix. The data showed a wide variation of GOD activity production ranging from 3.31 U/mL to 62.93 U/mL. This variation showed the importance of this step to select the most influent factors and the level of the others. The main effect of each variable was estimated by evaluating the contrast coefficient (b). Indeed, temperature, agitation, inoculum size and NaNO3 displayed a negative effect on GOD production, whereas yeast extract, peptone, sucrose and corn steep liquor had a positive effect on it. The critical effect of these factors on GOD production was confirmed by statistical analysis, particularly by P-value and t-test (Table 1). The factors having the most important contrast coefficient including temperature, inoculum size, yeast extract and corn steep liquor were the most significant variables affecting GOD production by statistical analysis. These factors showed, respectively, the highest level of significance with a t-value of −2.64, −2.5, 2.17 and 1.74 and a very low P-value, demonstrating their significant effect on GOD production. They presented 86% of the cumulative effects. For further optimization, the level of the most significant factors was optimized by CCD, while insignificant ones were used in all trials at their optimum level.
3.5.6 Optimization of the levels of the main factors
Based on the results given above, four factors (temperature, inoculums size, yeast extract, and corn steep liquor) were chosen as critical variables affecting GOD production. Central Composite Design was used to optimize the level of the selected factors and to obtain the highest GOD activity.
The system performance was explained by the following quadratic model equation:
(2) |
When Y is the GOD activity (U/mL), X1, X2, X3 and X4 are respectively the temperature (°C), the inoculum size (%), the yeast extract and corn steep liquor contents (g/L).
The regression analysis results showed that the F-value was 18.968, with a very low probability value (P < 0.0001) indicating the significance of the model. The closeness of experimental and predicted GOD activity can be expressed by the coefficient of determination R2. In this case, it is equal to 0.941, demonstrating that only 5.9% of the total variation experiment was not explained by the model. The adjusted R square (predicted R2) of 0.84 explains the good agreement between the experimental and the predicted results. The model equation related to GOD production shows an important negative linear effect for yeast extract (X3) and corn steep liquor (X4), and a significant positive linear effect for temperature (X1). In this model, the interactions between yeast extract with, respectively, inoculum size (X2X3) and corn steep liquor (X3X4) show a significant positive effect on GOD production, whereas the temperature–inoculums interaction size (X1X2) shows a negative effect. However, the interaction between temperature and, respectively, yeast extract (X1X3) and corn steep liquor (X1X4) is the less significant. These results were confirmed by Student's t-test (α = 0.05) (Table 2).
Analysis of the model terms by Student's test.
Coefficients | Unstandardized coefficients | Standardized coefficients | t | Significance | |
B | Standard error | Beta | |||
X 1 | 23.16 | 4.43 | 5.26 | 5.22 | 0.30E-04 |
X 3 | −94.29 | 14.22 | −4.28 | −6.63 | 1.15E-06 |
X 4 | −95.16 | 16.54 | −4.33 | −5.75 | 8.68E-06 |
X 1 X 1 | −0.38 | 0.05 | −5.26 | −6.78 | 8.23E-07 |
X 1 X 2 | −4.64 | 0.55 | −3.82 | −8.41 | 2.52E-08 |
X 1 X 3 | 0.73 | 0.39 | 1.42 | 1.89 | 0.07 |
X 1 X 4 | 1.61 | 0.39 | 2.48 | 4.16 | 4.08E-04 |
X 2 X 3 | 23.19 | 2.75 | 3.82 | 8.41 | 2.53E-08 |
X 3 X 4 | 8.71 | 1.94 | 2.67 | 4.48 | 1.83E-04 |
Response surface plots supply a method to predict the response for the various values of the test factors. The contour plots help in determining the interactions types between test factors. A saddle or elliptical contour plot of response surfaces indicates that the interaction between the related factors is significant, whereas a circular contour demonstrates that the interaction between the corresponding variables is negligible [27]. Fig. 8 shows the mutual interaction between the inoculums size and yeast extract. An increase in GOD production was recorded in the maximum and minimum levels of the two factors, respectively. Thus, GOD activity evolution was observed with the simultaneous decrease or increase of these two factors.

(Colour online.) Response surface plot of GOD activity, showing the interactions among the size of the inoculums and the concentration of the yeast extract (temperature and corn steep liquor content were set at the central point).
3.5.7 Validation
The model validity was examined by additional experiments using the optimal culture conditions: temperature 30 °C, agitation 150 rpm, inoculum size 1.5% and optimum culture medium composition: sucrose 20 g/L, yeast extract 4 g/L, corn steep liquor 2 g/L, peptone 2 g/L, KCl 0.5 g/L, MgSO4·7H2O 0.5 g/L, KH2PO4 1.5 g/L and with traces of CuSO4, ZnSO4, MnCl2 and FeSO4.
Glucose oxidase production yield (81.48 U/mL) was absolutely more important than that obtained during the preliminary study (31.25 U/mL). Thus, GOD activity was multiplied by a factor of 2.6. The expected result (82.17 U/mL) was very close to the experimental one (81.48 U/mL).
4 Discussion
For many decades, plant diseases caused by phytopathogenic fungi have created challenging problems in agriculture and caused real economic and environmental threats [28]. Crop rotation breeding for resistant plant varieties and using chemical fungicides are inefficient to control the diseases of vegetative crops [29,30]. Therefore, the development of novel antifungal agents can be useful for controlling plant diseases. Recently, screening has been oriented more and more towards biomolecules that possess a selective action against these fungi without being toxic to the ecosystem [31].
Glucose oxidase has been extensively researched as an antimicrobial agent to be used in human medicine and food preservation [32]. Despite this remarkable success, the share of GOD as biofungicides has not been reported yet. To the best of our knowledge, there were no earlier reports regarding the antifungal properties of GOD against phytopathogenic fungi. Thus, this study may be the first report suggesting its potent antifungal activity, which would allow it serve as a new cost-effective biofungicide to control pathogenic fungi in agricultural fields. In this article, we strive to evaluate the potential of GOD produced from a novel strain K38 isolated from contaminated cereal samples to control plant pathogenic fungi. By virtue of its morphological and ITS region sequence homology, strain K38 was identified as A. tubingensis. The significance of GOD in the biological control process was improved through several tests to ensure their potentials to inhibit mycelium growth and spores production of the phytopathogenic fungus F. solani as well as to reduce the incidence and severity of the disease in in vivo assays.
The results revealed that the partially purified GOD has a potential antifungal activity against F. solani. GOD inhibited mycelia growth and spore germination of F. solani in a dose-dependent manner in vitro, indicating that the activity of GOD may involve a direct fungitoxic property. Additional assays showed that the growth of F. solani treated with GOD at a concentration of 125 AU could not be restored after its transfer to a neutral culture medium. This led to the conclusion that GOD was a fungicide agent. Microscopic observation showed a morphological alteration in the phytopathogen F. solani, which confirms the fungicide effect of the GOD. Thus, GOD activity is not limited to the inhibition of F. solani mycelial growth, but extends also to the vital functions of the pathogen. The mechanism for the antifungal activity of the GOD has not been fully elucidated. However, it could presumably be attributed to the fact that GOD treatment may damage cell membrane and leakage of intracellular proteins and sugars, thus inhibiting the fungal phytopathogen [33]. GOD has a versatile ability to inhibit the growth of microbes by naturally produced hydrogen peroxide [32]. It was largely reported in the literature that H2O2, at very low concentrations (at the μg level), inhibits the growth of microorganisms. Recently, Oliveira et al. [34], Heller and Tudzynski [35] have reported that H2O2 has direct antimicrobial effects and that it is involved in other defence responses, such as cell wall modification, lipid peroxidation, phytoalexin production and defence-related gene activation. It also serves as an intra- or intercellular signalling molecule in the activation of plant defence mechanisms against pathogen attack. In fact, the H2O2 effect may involve the elevation of defence enzymes [36].
In vivo, the fungicidal activity of the partially purified GOD against F. solani was also investigated on tomato root infestation. The finding revealed that both preventive and curative GOD treatments were effective in reducing fungal infection. However, the greatest control was consistently achieved in the curative treatment. The greatest control was consistently achieved in the curative treatment because of the immediate interaction between GOD and the phytopathogen F. solani. In the case of the preventive assay, however, it depended on the time taken by GOD to reach infected parts and therefore to affect GOD activity. Our results are in concurrence with those of Wang et al. [37], who also demonstrated that, compared with the preventive effect, lactoferrin exhibited significant curative effect against grey mould on tomato plants caused by Botrytis cinerea. Similarly, Soylu et al. [1] reported that the treatment with origanum essential oil provided good protection against B. cinerea causing grey mould on tomato plants, especially as a curative treatment. These reports suggested that lactoferrin as well as origanum essential oil exerted their greatest effect on early fungal development on the leaf surface, such as spore germination, germ tube growth and/or appressorium formation.
Considering the inhibition of mycelial growth and spores production of F. solani in vitro and the incidence of disease symptoms on GOD treated tomato plants, A. tubingensis CTM 507 GOD may represent an important biocontrol agent versus F. solani. The promising properties of A. tubingensis CTM 507 GOD, field trials, have helped to improve GOD production levels through the optimization of media components and culture conditions and thus to decrease production cost. The optimization of the fermentation process using statistical experimental design clearly demonstrated the impact of the process parameters on the enzyme yield as well as their independent nature in influencing the ability of the fungi to synthesize the enzyme. Thus, the effect of environmental conditions on the GOD production could play an important role in the induction or repression of the enzyme by using specific compounds.
Carbon and nitrogen sources, pH and CaCO3 concentration were selected as the main effective factors by the one-factor-at-a-time method. Indeed, sucrose and glucose were identified as the best carbon sources. These results suggest that glucose is necessary for the effective induction of GOD in A. tubingensis CTM 507. This implies that the enzyme is specific to glucose-rich substrates and can be produced constitutively up to a certain level. Similarly, Bankar et al. [18], who investigated the effect of different carbon sources on the growth and GOD activity of A. niger, reported that the maximum GOD yield (0.0067 U/mL) was achieved with sucrose as a carbon source. A kinetic study conducted for 48 h indicated that GOD production started after 4 h and reached its maximum after 32 h. Further incubation did not allow an increase in GOD yield and instead resulted in a decline. The reduction in GOD yield after the optimum period was probably related to strain morphology and biomass growth. In addition, the biomass profile showed the presence of two phases of exponential growth. A possible explanation of this behaviour could be a successive use of two different substrates (sucrose and gluconic acid) as carbon sources. As a result, the gluconic acid, produced in the first phase of approximately 22 h from sucrose by means of GOD action, can be considered as a useful source of carbon for growth in the second phase of 30 h [38]. From this interpretation, the observed changes of the fungal morphology during fermentation can be justified. Thus, in the first culture phase of fermentation, A. tubingensis CTM 507 cultures are grown in quasi-spherical aggregates called pellets. After being (48 h of fermentation), the pellets were destroyed and the filamentous fungi grew as free mycelia. This particular morphological form (pellets) can promote a lower viscosity medium resulting in easier nutriments diffusion, a sufficient oxygen uptake by cell mycelium and therefore an increase in enzyme production [39]. The autolysis of pellets can be explained by gluconic acid catabolite repression. This phenomenon can also be explained by the fact that, in the final phase of the fermentation, the pellets become bulky, with pores increasingly closed (due to the growth of the biomass), which cause the drop in oxygen.
The investigation of the effect of various organic and inorganic nitrogen sources on the enzyme production showed that corn steep liquor and sodium nitrate remained the most suitable sources. Hence, yeast extract and casein peptone were the best biomass producers since they were balanced sources of protein in terms of composition and accessibility [40]. It could also be possible that organic nitrogen provided by yeast extract and peptone contained most of amino acids required by A. tubingensis CTM 507 and that these amino acids could be directly absorbed by the fungal cells. These results are in good agreement with the studies done by Kona et al. [41], who reported that corn steep liquor and NaNO3 were the principal inducers of GOD production and that none of the tested nitrogen sources (nitrate of calcium, sodium, ammonium and potassium, yeast extract, malt extract and peptone) stimulated GOD production. Likewise, Bankar et al. [18] tested different nitrogen sources on the growth and GOD activity of A. niger. They found that sodium nitrate and proteose peptone had a remarkable effect on GOD production, while mycological peptone was the best biomass producer. GOD activity, which used all studied nitrogen sources, was weaker than the initial one. The decrease in GOD activity can be explained by the presence of an interaction and a competitive action between nitrogen sources for fungus growth and enzyme production. The stimulating effect of CaCO3 on GOD production was well emphasized in the literature. In the frame of the present study, enzyme production was greatly affected by the presence of CaCO3 in the medium. Indeed, GOD production was increased when 30 g/L of CaCO3 was added to the medium. This result corroborates the study of Sabir et al. [27] and Bankar et al. [18], who found that 30 g/L of CaCO3 was the optimum level for the highest GOD production by Penicillium notatum and A. niger NCIM 545, respectively. In fact, the inclusion of calcium carbonate in the growth media might cause a metabolic shift from the glycolytic pathway to the direct oxidation of glucose by GOD. Thus, the induction of this enzyme by CaCO3 may compile with this mechanism [26]. The pH value has also been reported to play an important role in enzyme production. The highest GOD activity was recorded using a medium with an initial pH of 6.5. A large range of pH from 4.0 to 7.0 affecting GOD production by a variety of microorganisms was reported in the literature. The optimum pH for the production of GOD by Penicillium chrysogenum SRT19 Strain [42] and Penicillium fellutamum [43] was 6.5, while it was 4.0 and 5.5 for A. niger [44,47].
The significant variables necessary to enhance GOD production were selected using the Plackett–Burman design. Among the variables screened, temperature, inoculums size, yeast extract and corn steep liquor were identified as the most significant components influencing GOD production by A. tubingensis CTM 507. The Central Composite Design exploited in the present study enabled us to investigate the culture conditions that approximately supported a three-fold increase in GOD production. A high degree of similarity was observed between the predicted and experimental values, which demonstrates that the generated model gave an adequate prediction of GOD activity.
The present investigation dealt with A. tubingensis CTM 507 GOD as a promising mechanism that could be used as a biological control agent against phytopathogenic fungi. Hence, A. tubingensis CTM 507 GOD preparations could be ideally exploited for field trials to achieve the goal of practical applications of biocontrol. However, further studies need to be conducted to evaluate the efficiency of this enzyme on a wide range of plant microbial diseases in commercial greenhouses and against other phytopathogenic fungi.
5 Conclusion
In this work, A. tubingensis CTM 507 GOD has been shown to inhibit mycelium growth and spores production in vitro and to control the tomato diseases caused by F. solani in vivo. In the light of these studies, we can conclude that GOD can be used as alternative biofungicides to synthetic fungicides against phytopathogenic fungi, since it is multifunctional, biodegradable, non-persistent in the environment and cheap to produce. The possibility of developing glucose oxidase to be used in crop protection may be an attractive venture.
Disclosure of interest
The authors declare that they have no conflicts of interest concerning this article.
Acknowledgements
This work was funded by the Tunisian Ministry of Higher Education and Scientific Research and Technology (contract program LMB-CBS, grant No. RL02CBS01). The authors would like to express their sincere gratitude to Mrs. Salma Karray for her constructive proofreading and valuable language polishing services.