1 Introduction
Fritillaria meleagris L. (Liliaceae) is a valuable perennial bulbous plant often used as an attractive ornamental species. Fritillaries are mainly distributed throughout the temperate climates of the northern hemisphere and spend a specific period of the year in the form of dormant bulbs underground. Almost all Fritillaria species contain different alkaloids with interesting pharmaceutical properties (used as antitussive and expectorant herbs); due to this feature, many of these species have been used for centuries in the traditional medicine of China [1], Turkey [2], and Japan [3]. The production of F. meleagris by conventional methods (seed germination or from bulbs) is very slow and unpredictable, and it takes several years to obtain a whole plant [4]. Due to these limitations, there is great interest in improving protocols for the micropropagation of species of this genus that have so far proved relatively successful [5–7]. In vitro plant propagation can lead to effective and rapid multiplication of fritillary species through various morphogenetic pathways when conventional methods are ineffective. In most of the described protocols, parts of bulbs or bulb scale segments were used as explants [4] from which new bulbs could be regenerated. Morphogenesis in F. meleagris can be established via organogenesis or somatic embryogenesis. By the process of organogenesis, unipolar organs are formed de novo, while somatic embryogenesis is the process of forming embryos from somatic cells. Both morphogenetic pathways lead to the formation of the whole plant, but it is still not known why some cells form somatic embryos, while others are subject to the process of organogenesis [8]. Bulb scales can be used as explants for the induction of morphogenesis in many Fritillaria species, but they are very susceptible to infection [9]. Using the parts of the bulb that formed in vitro overcomes problems with contamination of the plant material and can significantly increase the number of regenerated plants.
A large percentage of bulbs regenerated in vitro become dormant [10,11]. In order to overcome dormancy, a period of several weeks of exposure to low temperature is often needed. Low-temperature treatment, applied prior to bulb growth on nutrient media containing different growth regulators, can have a positive impact on the percentage of regeneration of new bulbs [12]. As an alternative to cold pre-treatment, an increased concentration of sucrose in the medium may also improve bulb regeneration and overcome dormancy and thus enhance morphogenesis in vitro [13]. During a period of dormancy, bulbs accumulate greater amounts of sugar. These accumulated carbohydrates are used during the next vegetative period [14].
Somatic embryogenesis of F. meleagris has been achieved using two different types of explants: mature zygotic embryo [15] or leaf base [7]. However, the molecular basis of somatic embryogenesis has not been sufficiently clarified. In most cases, analysis has been performed on total protein expression during the process of somatic embryogenesis, leading to the discovery of markers of somatic embryogenesis [16]. Potential markers of somatic embryogenesis include esterases, characterized as acidic esterases, detected in suspension cells of Dactylis glomerata. These enzymes are specific to embryogenic cells that have the potential to regenerate the whole plant and are absent from non-embryogenic cell suspensions [17]. Esterases are a group of hydrolytic enzymes that catalyze the decomposition reaction of esters from which acids and alcohols are obtained. Most data on the role of esterases in the physiological processes are related to animals and microorganisms, with little information available on plant esterases [18]. The most commonly studied esterases in plants are aryl and carboxyl esterases that can be detected according to the protocol established by Burlina and Galzigna [19], which are based on the affinity of esterases for 1- and 2-naphthyl acetate. Increased esterase activity has been found during somatic and zygotic embryogenesis [20]. The role of these enzymes in the process of somatic embryogenesis can be attributed to the degradation of pectin, which is an integral part of the cell wall, by a process of demethylation and deacetylation, thus directly supporting the process of cell proliferation [21]. Increased esterase activity has also been shown in Mammilaria gracillis during regeneration in the process of organogenesis [22]. The greater expression of esterase isoforms in the embryogenic callus of this species in relation to sprouting tissues supports the hypothesis that different isoforms of esterase participate in the morphogenetic process. Similar results were obtained in Plantago ovata where the isoforms of esterase differ in the callus and regenerated shoots [23]. In addition to the process of morphogenesis, esterases are involved in other processes, such as pollen germination [24]. These enzymes can be used for the analysis of genetic structure in populations [25], as markers for the detection of certain types of stress [26], in the determination of grafting compatibility [27], as growth markers of different plant cell cultures [28] or for the characterization of some cultivars using esterase isoenzymes as molecular markers [29].
Peroxidases also have an important role in morphogenesis in plants [22]. Peroxidases are involved in a large number of reactions in the cell, such as the oxidation of phenolic compounds, lignification and linking cell wall polysaccharides [30]. The role of these enzymes has been confirmed in the growth and development of plants [31], auxin catabolism [32] and in defending plants against pathogens and oxidative stress [33]. There are three groups of peroxidase, but the most common is group III, which is also called plant peroxidase [32]. Peroxidase activity and different isoforms of peroxidase have been reported as markers of somatic embryogenesis in some studies [34,35]. Moreover, peroxidase can be applied as a marker of morphogenesis and tumorization, as demonstrated in horseradish tissue culture [36].
The aim of the present study was to identify the isoforms of esterases and peroxidases during morphogenesis in vitro of F. meleagris as well as to investigate the effect of different sucrose concentrations and temperatures on bulb growing.
2 Materials and methods
2.1 Plant material
Bulbs of F. meleagris were obtained and cultured according to a previously published procedure [15], which includes bulb regeneration from embryogenic callus. Somatic embryos and their multiplication, formation of bulblets and shoots were observed on Murashige and Skoog medium (MS) [37] supplemented with 3% sucrose, 0.7% agar, 250 mg/L casein hydrolysate, 250 mg/L l-proline and 1.0 mg/L thidiazuron (TDZ). All cultures were maintained at a temperature of 24 ± 2 °C and a 16-h-light/8-h-dark photoperiod with an irradiance of 40 W/m2.
The influence of an increased sucrose concentration on the growth and on the development of bulbs was investigated on a hormone-free medium containing, rather than the standard sucrose concentration of 3%, an elevated concentration of 4.5 or 6% sucrose. Bulbs were grown at standard temperature (24 °C) or low temperature (4 °C) during the first four weeks, and then all bulbs were grown for four weeks at a standard temperature. Changes in fresh weight were observed after four and eight weeks of growth in vitro.
Some of the in vitro formed plants were stored at 4 °C, and some were cultured on a medium with an increased sucrose concentration (4.5% (m/v)). Both pre-treatments were carried out for 30 days and plants were cultured on a medium without growth regulators. The bulbs regenerated in the pre-treatment were used for further experiments.
2.2 Induction of in vitro morphogenesis
Parts of previously regenerated bulbs, i.e. individual scales, were separated from the basal plate of bulbs (referred to as bulb scales). Bulb scales, grown on different pre-treatments, were cultured on media with different concentrations of growth regulators with the aim of inducing morphogenesis.
Control plants were cultured under standard conditions on hormone-free medium.
Media supplemented with different concentrations of 2,4-dichlorophenoxyacetic acid and kinetin (2,4-D and KIN, in mg/L: 0, 0.1, 0.5, 1, 2, 5, 10) or TDZ (in mg/L: 0, 0.05, 0.1, 0.2, 0.5, 1, 2) were used. The numbers of somatic embryos and newly formed bulbs were counted after 30 days in culture. The experiments were performed as three replicates containing 75 explants (bulb scales) per concentration. Bulb scale segments were collected every seven days from the beginning of morphogenesis, i.e. after 7, 14, 21, and 28 days.
The same concentration of plant growth regulators was used in the experiment because the different combinations of growth regulators were too complicated for interpretation of the results.
Also, previous experiments in our laboratory were done at different concentrations of these growth regulators and it would be unnecessary to repeat them [7].
2.3 Enzyme extraction
To determine peroxidase and esterase isoforms, bulb scales on a medium enriched with 2,4-D and KIN (1 mg/L, respectively) or TDZ (1 mg/L) were used.
Frozen (–70 °C) bulb scales (500 mg) were homogenized on ice in 4 mL of 0.1 M potassium phosphate extraction buffer (K–P buffer) [pH 6.8, containing 200 mg of insoluble polyvinyl pyrrolidone (PVP) and phenylmethylsulfonyl fluoride (PMSF)]. The homogenate was centrifuged for 5 min at 12,000 g at 4 °C. The samples for esterase detection were homogenized in 0.2 M Tris–HCl buffer, pH 8. The protein content of the supernatants was determined according to Bradford's method [38].
2.3.1 Electrophoretic separation of peroxidase and esterases isoenzymes
For the separation of peroxidase and esterase isoforms and isoelectric focusing, a polyacrylamide gel was used, containing 3.75 mL of acrylamide, 4 mL of glycerol, 6.5 mL of deionized water, 12 μL of tetramethylethylenediamide (TEMED), and 75 μL of ammonium persulfate (APS). Isoelectric focusing was performed using a Multiphor II electrophoresis system (Pharmacia-LKB Biotechnology) according to the manufacturer's instructions. Focusing was carried out on a gel with ampholytes in a pH range from 3.0 to 10.0, at 7 W of constant power for 1.5 h at 10 °C. A broad pI kit (GE Healthcare) was used for the isoelectric point (pI) markers. After the run, one part of the gel was stained with Commassie Brilliant Blue (CBB) and the other was processed for enzyme activity staining [39].
Peroxidase isoforms were determined by gel incubation for 30 min in a 50 mM monopotassium phosphate buffer (pH 5.8) that contained guayacol (10 μL of guayacol was dissolved in 10 mL of 20 mM Tris, pH 7.0) and 10 μL of hydrogen peroxide according to the modified method of Siegel and Galston [40]. Gel analysis was performed using the TotalLab TL 120 graphics package. Isoelectric focusing of esterases was performed as described above.
Twenty milligrams of 1-naphtylacetate and 20 mg of 2-naphtylacetate (substrates for esterases) were dissolved in 2 mL of 50% (v/v) acetone and mixed with 100 mL of 50 mM K-P buffer (pH 7.2). For isoelectric focusing, 15 μL of sample was used. The gel was incubated for 30 min in this solution at room temperature, rinsed in tap water, and stained during 25 min at 37 °C in 0.2% Fast Blue RR Salt (Sigma Chemical Co, New York). The staining solution was prepared by dissolving the 50 mg of Fast Blue RR Salt in 10 mL of distilled water and added to the gel. By staining the gel with only one substrate, it was shown that 1-naphtylacetate gave a dark brown color, while the 2-naphtylacetate gave a pink color.
2.4 Statistical analysis of data
The results of all experiments are presented as means ± standard errors. The statistical analyses were performed using StatGrafics software version 4.2 (STSC Inc., Rockville, Maryland, USA). Data were subjected to analysis of variance (ANOVA) and comparisons between the mean values of treatments were made using the least-significant-difference (LSD) test calculated at a confidence level of P ≤ 0.05. A population of 30 bulbs was used in all treatments. All measurements were repeated three times.
3 Results
3.1 Influence of increased concentrations of sucrose on the growth of bulbs formed in vitro
The greatest mass increase of bulbs was observed when bulbs were grown on a medium with 4.5% sucrose for a first four weeks. At this concentration of sucrose, bulbs that had previously been grown at a temperature of 4 °C showed a mass increase of 21.04% compared to bulbs grown on a medium with 3% sucrose. Bulbs cultured at a standard temperature (24 °C) had a mass increase of 40.96% compared to bulbs that were grown at standard sucrose concentrations (Fig. 1A).
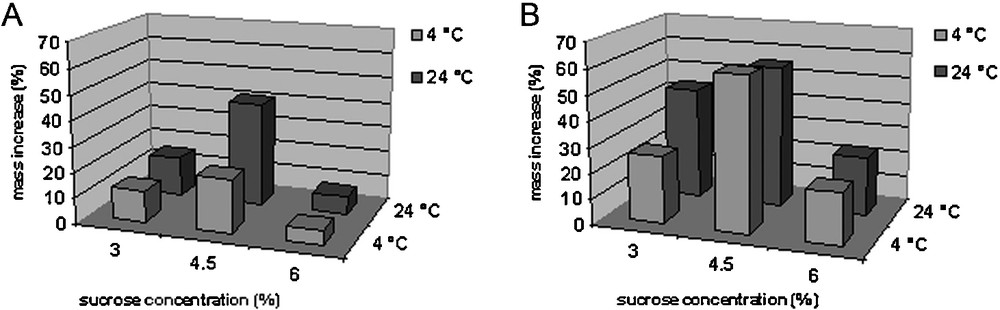
Effect of different sucrose concentrations and temperatures on fresh mass increase of newly formed bulbs of F. meleagris. A. Mass increase of bulbs cultured at 4 °C and 24 °C for 4 weeks. B. Mass increase of bulbs cultured for 4 more weeks at 24 °C (total of 8 weeks in culture).
The mass increase of bulbs after four weeks of growth at standard temperature (eight weeks in culture) is shown in Fig. 1B. The greatest increase in mass was observed on bulbs grown in the medium containing 4.5% sucrose and previously grown for four weeks at low (4 °C) and standard (24 °C) temperatures.
It was found that a higher concentration of sucrose in the nutrient medium (4.5%) combined with growing bulbs at a low temperature had a positive effect on the increase in the mass of bulbs. A sucrose concentration of 6% in the medium led to a decrease in growth at both investigated temperatures.
3.2 Induction of morphogenesis in bulb-scale culture
3.2.1 Induction of morphogenesis in bulb-scale culture on a medium with 2,4-D and KIN
In almost all tested nutrient media enriched with 2,4-D and KIN, morphogenesis in vitro was induced via processes of somatic embryogenesis and organogenesis (Tables 1 and 2), respectively. Somatic embryogenesis occurred in all of the investigated nutrient media. Organogenesis was lacking on bulb segments grown on the medium with the highest concentration of 2,4-D and KIN (10 mg/L) under standard conditions and after having grown the bulbs in a medium with an increased concentration of sucrose.
Effect of two pre-treatments and different concentration of growth regulators applied after, on the number of somatic embryos (SE) in a bulb-scale culture of F. meleagris. Values represent means ± SE, bulblets of three replicates of 50 explants each. Means followed by the same letters within columns are not significantly different according to LSD test at the P ≤ 0.05 probability level.
Medium 2.4-D and KIN (mg/L) |
Pre-treatment | ||
24 °C | 4 °C | 4.5% sucrose | |
No of SE | No of SE | No of SE | |
0 | 2.00 ± 0.25cde | 2.60 ± 0.27ef | 1.80 ± 0.20cd |
0.1 | 2.93 ± 0.30fg | 2.80 ± 0.34efg | 1.93 ± 0.28cde |
0.5 | 4.06 ± 0.34h | 3.13 ± 0.32fgh | 2.26 ± 0.33def |
1.0 | 6.20 ± 0.42i | 6.20 ± 0.35i | 3.66 ± 0.39gh |
2.0 | 2.33 ± 0.37def | 2.53 ± 0.37def | 3.00 ± 0.52efgh |
5.0 | 1.00 ± 0.25ab | 1.33 ± 0.18bc | 2.00 ± 0.37cde |
10.0 | 0.60 ± 0.19a | 1.40 ± 0.27bc | 1.73 ± 0.35bcd |
Effect of two pre-treatments and of different concentration of growth regulators applied after, on the number of bulblets in a bulb-scale culture of F. meleagris. Values represent means ± SE, bulblets of three replicates of 50 explants each. Means followed by the same letters within columns are not significantly different according to LSD test at the P ≤ 0.05 probability level.
Medium 2.4-D and KIN (mg/L) |
Pre-treatment | ||
24 °C | 4 °C | 4.5% sucrose | |
No of SE | No of SE | No of SE | |
0 | 1.40 ± 0.23de | 1.86 ± 0.19ef | 1.26 ± 0.15d |
0.1 | 1.46 ± 0.23de | 2.46 ± 0.32fg | 1.46 ± 0.23de |
0.5 | 1.33 ± 0.21de | 2.20 ± 0.27fg | 2.06 ± 0.35efg |
1.0 | 1.86 ± 0.25ef | 2.80 ± 0.22g | 1.86 ± 0.27ef |
2.0 | 0.80 ± 0.17c | 1.06 ± 0.22cd | 0.73 ± 0.18c |
5.0 | 0.20 ± 0.14ab | 0.46 ± 0.25bc | 0.13 ± 0.09ab |
10.0 | 0a | 0.13 ± 0.13ab | 0a |
The greatest numbers of somatic embryos and bulblets were formed on medium enriched with 2,4-D and KIN and at a concentration of 1 mg/L each, after all the applied pre-treatments. At the aforementioned concentrations, the highest number of somatic embryos was formed after cold pre-treatment (6.20 ± 0.35) (Table 1) and after standard growth conditions (6.20 ± 0.42). The greatest number of bulbs (2.80 ± 0.22) was regenerated on bulb segments that were previously subjected to cold pre-treatment (Table 2).
Concentrations of growth regulators greater than 1 mg/L led to a decrease in the number of bulbs and somatic embryos. These values were the lowest at the highest concentration (10 mg/L) of applied growth regulators.
Morphogenesis also occurred in the medium without growth regulators. Bulb scales rarely formed a callus, and somatic embryos differentiated on explants after only seven days of cultivation on all combinations of auxins and cytokinins (Fig. 2A and B). Somatic embryos usually formed at the basal part of the explant; they were mostly white to light green. Fig. 2A shows globular somatic embryo developmental stages starting after seven days in culture. The somatic embryo was heart-shaped after 14 days (Fig. 2C) and reached the cotyledonary stage after 21 days (Fig. 2D and E). Fully developed somatic embryos were observed after 28 days (Fig. 2F). At the end of the subculture, somatic embryos were separated from the initial explants. Somatic embryogenesis was asynchronous, which means that the initial explants formed somatic embryos at different developmental stages.
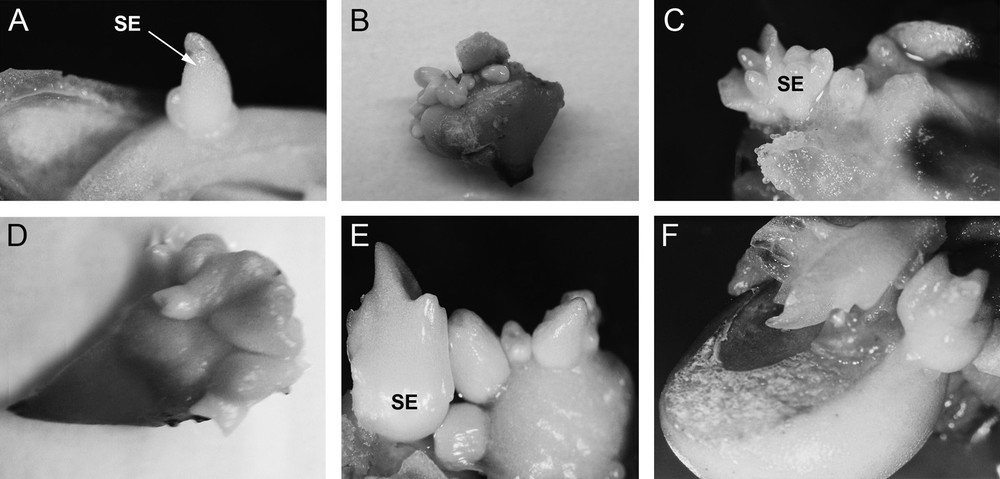
Induction of morphogenesis in F. meleagris in bulb-scale culture on a nutrition medium with 2,4-D and KIN (1 mg/L each) after: (A, B) 7 days, (C) 14 days, (D, E) 21 days and (F) 28 days in culture. SE: somatic embryo.
Along with somatic embryogenesis, bulb scale segments grown on medium enriched with 2,4-D and KIN underwent the process of organogenesis and regeneration of new bulbs (Fig. 3D). Organogenesis was direct, meaning that new bulbs were regenerated directly on the explant without the callus interphase.
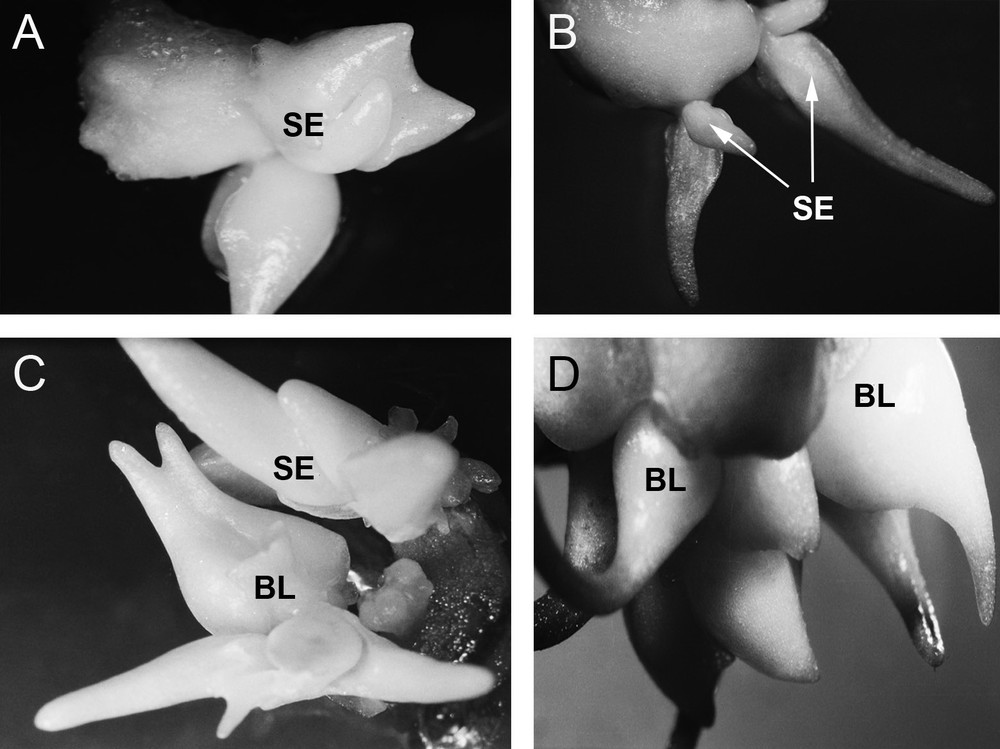
Induction of morphogenesis in F. meleagris in bulb-scale culture on a nutrition medium with TDZ (1 mg/L) after: (A) 7 days, (B) 14 days, (C) 21 days and (D) 28 days in culture. SE: somatic embryo; BL: bulb.
3.2.2 Induction of morphogenesis in bulb-scale culture on a medium containing TDZ
In all tested nutrient media enriched with TDZ, morphogenesis was induced in vitro via the processes of somatic embryogenesis and organogenesis (Tables 3 and 4), respectively. The greatest number of somatic embryos was formed on a medium supplemented with TDZ at the concentrations of 0.5 and 1 mg/L, while the greatest number of bulblets was obtained on a medium supplemented with 1 mg/L TDZ compared to the medium enriched with 2,4-D and KIN. More bulbs were regenerated on a medium supplemented with TDZ compared to a medium enriched with 2,4-D and KIN.
Effect of two pre-treatments and different concentration of TDZ applied after, on the number of somatic embryos (SE) in a bulb-scale culture of F. meleagris. Values represent means ± SE, bulblets of three replicates of 50 explants each. Means followed by the same letters within columns are not significantly different according to LSD test at the P ≤ 0.05 probability level.
Medium TDZ (mg/L) |
Pre-treatment | ||
24 °C | 4 °C | 4.5% sucrose | |
No of SE | No of SE | No of SE | |
0 | 2.00 ± 0.25bc | 2.60 ± 0.27cd | 1.80 ± 0.20b |
0.05 | 2.46 ± 0.33cd | 1.93 ± 0.33bc | 2.00 ± 0.21bc |
0.1 | 3.53 ± 0.30ef | 2.46 ± 0.29cd | 3.06 ± 0.33de |
0.2 | 4.66 ± 0.48fgh | 3.80 ± 0.51ef | 4.26 ± 0.37fg |
0.5 | 5.46 ± 0.36h | 5.66 ± 0.31h | 5.53 ± 0.40h |
1 | 5.06 ± 0.40gh | 5.33 ± 0.47gh | 5.66 ± 0.33h |
2 | 1.46 ± 0.25ab | 1.66 ± 0.33ab | 1.13 ± 0.23a |
Effect of two pre-treatments and of different concentration of TDZ applied after, on the number of bulblets in a bulb-scale culture of F. meleagris. Values represent means ± SE, bulblets of three replicates of 50 explants each. Means followed by the same letters within columns are not significantly different according to LSD test at the P ≤ 0.05 probability level.
Medium TDZ (mg/L) |
Pre-treatment | ||
24 °C | 4 °C | 4.5% sucrose | |
No of SE | No of SE | No of SE | |
0 | 1.40 ± 0.23bc | 1.86 ± 0.19cd | 1.26 ± 0.15b |
0.05 | 2.00 ± 0.19cd | 2.00 ± 0.23cd | 1.40 ± 0.16bc |
0.1 | 2.13 ± 0.16de | 1.86 ± 0.19cd | 2.06 ± 0.24cde |
0.2 | 2.26 ± 0.18de | 1.93 ± 0.24cd | 2.60 ± 0.25ef |
0.5 | 2.60 ± 0.19ef | 2.33 ± 0.21def | 2.46 ± 0.30def |
1 | 2.93 ± 0.22f | 2.93 ± 0.24f | 2.73 ± 0.24ef |
2 | 0.73 ± 0.18a | 0.93 ± 0.24ab | 1.40 ± 0.28bc |
The greatest number of somatic embryos (5.66 ± 0.31) were formed at a TDZ concentration of 0.5 mg/L after all pre-treatments (Table 3). The greatest number of bulbs was regenerated on bulb segments growing on a medium with 1 mg/L TDZ under standard conditions (2.93 ± 0.22) as well as on the medium of the same composition, but after cold pre-treatment (2.93 ± 0.24) (Table 4). TDZ at a concentration of 2 mg/L led to a decline in the number of somatic embryos and bulbs, but not to a complete absence of morphogenesis.
In bulb scale segments grown on media enriched with TDZ, direct somatic embryogenesis and organogenesis also occurred (Fig. 3). Somatic embryos were light green in colour. Somatic embryos first differentiated directly on the explant, at early stages of development (Fig. 3A). Somatic embryos were elongated after 14 days in culture. Subsequently, the regeneration of new bulbs occurred (Fig. 3B). Over the next week, there was further growth of somatic embryos and bulbs (Fig. 3C) leading to full development after four weeks in culture (Fig. 3D).
3.3 Detection of enzyme isoforms
To determine the peroxidase and esterase isoforms, bulb scales were cultured on a medium enriched with 2,4-D and KIN (1 mg/L each) or TDZ (1 mg/L) because these media were the most successful for the induction of F. meleagris morphogenesis in previous experiments.
3.3.1 Peroxidase isoforms
During the induction of in vitro morphogenesis in F. meleagris in bulb-scale culture, 11 peroxidase isoforms were observed, except in the case of bulbs grown in the medium containing 4.5% sucrose (Fig. 4A–C). Isoforms 1, 6 and 8 were not detected in the control bulb segments immediately after isolation of the explant. Isoform 2 was more intense than other isoforms in the control explants. The mentioned isoform, in explants cultivated on inductive nutrient media, had equal or even higher staining intensity when explants were grown during 21 and 28 days on media supplemented whit 2,4-D and KIN or TDZ. Isoforms 6 and 7 were less pronounced in bulb segments that were grown at 24 °C compared to the explants that were cultured with pre-treatment at 4 °C or on the medium containing an increased concentration of sucrose (Fig. 4A).
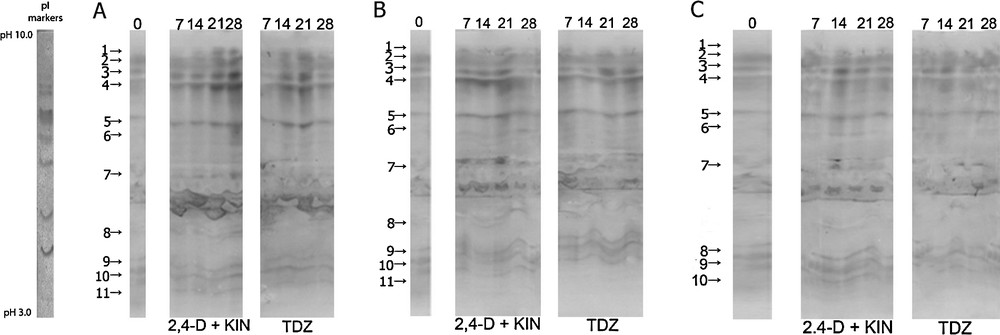
Isoforms of peroxidase in a bulb-scale culture of F. meleagris cultured on a nutrition medium with 2,4-D + KIN or TDZ after 30 days of pre-treatment culture at: (A) 24 °C, (B) 4 °C and (C) 4.5% sucrose. The number of days after which different isoforms of peroxidase were detected is indicated at the top of the image.
Isoforms 6 and 7 were less pronounced in the segments and bulbs that were previously grown at 4 °C and then transferred to the inductive nutrient medium with TDZ. Isoform 7 was highly expressed during the first three weeks of explant growth on the medium containing 2,4-D and KIN (Fig. 4B).
Previous cultivation of bulbs with an increased concentration of sucrose resulted in a reduction in the number of peroxidase isoforms, such that 10 isoforms were detected (Fig. 4C). Isoform 8 was not detected in these explants. Peroxidase isoforms were less intense than in bulb segments grown constantly at 3% sucrose. Isoform 7 was expressed only 14 days after the beginning of bulb segment growth in a medium containing 2,4-D and KIN.
3.3.2 Esterase isoforms
Six isoforms in control bulbs and more than six isoforms in segments of bulbs that were grown on a medium with growth regulators were detected for both esterase substrates used (Fig. 5A–C). Esterases were detected in the acidic and basic pH regions. The pI values were within the range of 3.5 to 8.65. A larger number of esterases (isoforms 3, 4, 5 and 6) were detected in the acidic part in the case of both substrates. Greater staining intensity in the acidic part indicated esterase when 2-naphthyl acetate was used as the substrate (Fig. 5A–C, right), and higher staining intensity in the basic part indicated esterase when 1-naphthyl acetate was used as the substrate (Fig. 5A–C).
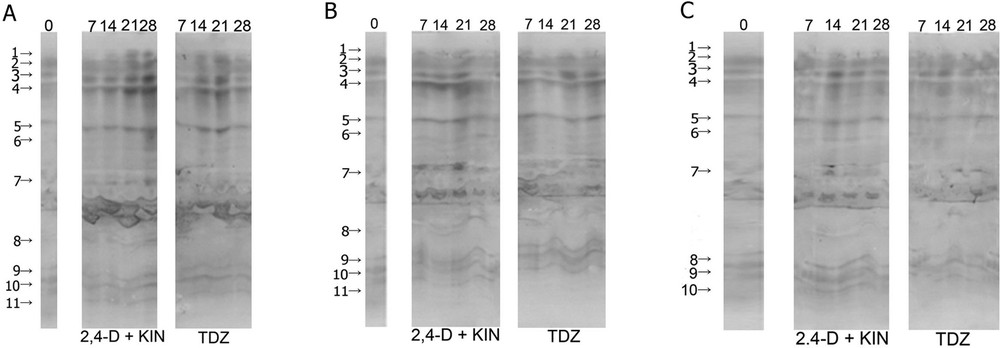
Isoforms of esterase shown with 1-naphtyl acetate (left) and 2-naphtyl acetate (right) in a bulb-scale culture of F. meleagris on a nutrition medium with 2,4-D + KIN or TDZ after 30 days of pre-treatment at: (A) 24 °C, (B) 4 °C, and (C) nutrition media with 4.5% sucrose. The number of days after which different isoforms of esterase were detected is indicated at the top of the image.
Isoforms of esterase in control explants (1 and 6) had stronger staining intensity detected with 1-naphthyl acetate compared to other isoforms, while esterase isoforms detected with 2-naphthyl acetate had a similar staining intensity. Esterase isoforms from control explants had weaker staining intensity in relation to the esterases isolated from the segments of bulbs that were grown on media with growth regulators.
On bulbs that were previously grown at 24 °C, an increase was observed in the number of esterase on a medium with 2,4-D and KIN after 14 days, detected with 1-naphthyl acetate. The bulb segments grown on the medium with TDZ contained all isoforms after 7 days of cultivation (Fig. 5A). In these bulb segments, a significant staining intensity of isoform 1 was observed, with a pI value of 8.45, after three weeks of explant growth on medium containing TDZ. After three weeks on the same medium, the maximum staining intensity of all six esterase isoforms was observed when 1-naphthyl acetate was used.
In the case of bulb segments pre-treated at 4 °C, higher staining intensity was observed in explants grown on a medium containing 2,4-D and KIN (Fig. 5B). In these explants, significant esterase staining intensity, detected with 1-naphthyl acetate, was also detected in the acidic spectrum of explants grown on medium with 2,4-D and KIN throughout the entire period, especially isoform 6 with a pI value between 5.20 and 5.85.
In the case of the bulbs pre-treated with a higher concentration of sucrose, the staining intensity of esterase was lower compared to the bulbs kept under standard conditions or at 4 °C (Fig. 5C). The maximal activity of isoforms 1 and 6 was observed after 28 and 14 days, respectively, on the medium containing 2,4-D and KIN after this pre-treatment.
4 Discussion
In this study, the morphogenetic process was investigated using bulb scales as explants on media that contained a combination of auxins and cytokinins (2,4-D and KIN) and on medium that contained only TDZ.
We investigated the effects of increased concentration of sucrose on the mass increase of bulbs of F. meleagris. The greatest mass increase of bulbs was found with a sucrose concentration of 4.5%. Increasing the concentration of sucrose in the nutrient medium had a positive effect on the number and mass of newly formed bulbs of F. thunbergii, where it was noted that the mass of bulbs increased by five- to seven-fold when sucrose was present in the nutrient medium at a concentration of 5 or 7% [41]. A similar effect was noted in lilies where an increase was observed in the dry weight of bulbs, which was correlated with increasing concentrations of sucrose in the nutrient medium [42]. It is assumed that adoption of sucrose from the culture medium was intense at higher temperatures, which is, in this case, 24 °C. Therefore, the increase in bulb mass is probably more intense at standard temperature [43] than at 4 °C. In addition, enzymes that break down sucrose are more active at higher temperatures. When bulbs of F. meleagris were grown for one month at a standard temperature after growing at 4 °C, the greatest mass increase was found in bulbs grown in the medium with 4.5% sucrose. Dormancy in bulblets was interrupted during cooling at 4 °C and led to increased metabolic processes and more effective adoption of sucrose from the culture medium, and thus an increase in the mass of bulbs.
Morphogenesis in F. meleagris in bulb-scale culture was achieved on media that contained a combination of 2,4-D and KIN or TDZ. These growth regulators are commonly used for the induction of somatic embryogenesis; 2,4-D is an auxin and TDZ is a cytokinin [44]. The induction of somatic embryogenesis in bulb-scale culture, using only 2,4-D or TDZ as growth regulators, has been previously observed in F. meleagris [15]. The differences in the optimum concentration of TDZ for the induction of somatic embryogenesis can be attributed to the possible long-term in vitro cultivation of plants in nutrient medium with TDZ, so that after a period of time in culture, higher concentrations of this plant growth regulator are required in order to obtain satisfactory results. These results show that a range of 0.5 to 1 mg/L TDZ was an optimal concentration for bulb regeneration of F. meleagris. Previous results from our laboratory have shown that the best bulb regeneration occurred on media with a TDZ concentration from 0.05 to 0.5 mg/L [15]. These results may be explained by the habituation of the explants to an increased concentration of TDZ in the medium in which the bulbs were grown previously.
In the leaf base culture of F. meleagris, direct somatic embryogenesis can be successfully induced using a medium enriched with 2,4-D or KIN [7]. In this study, the addition of 2,4-D in the nutrient medium with KIN stimulated the formation of somatic embryos, which reached a maximum at a concentration of 1 mg/L. Previous studies [44,45] have shown that somatic embryos do not form in different tissue cultures of Narcissus pseudonarcissus without 2,4-D in the nutrient medium. This auxin and cytokinin combination proved to be adequate for the induction of somatic embryogenesis in many plants with rhizomes as well as in bulb-like Astromeria [46], Iris [47], and tulip [48]. Bulbs were maximally regenerated on a medium with an auxin and cytokinin concentration of 1 mg/L each, but their numbers were lower than in a medium enriched with TDZ. Bulblet production in the bulb-scale culture of F. thunbergii showed a high frequency of bulblet regeneration using various concentrations of cytokinins and naphtyl acetic acid (NAA) [4]. In this paper, we used equal concentrations of an auxin and a cytokinin in the same medium. In order to improve the protocol, it will be necessary to test different combinations of these growth regulators to obtain better results.
The expression of esterase and peroxidase was examined in the bulb scale of F. meleagris, in which morphogenesis was induced on media that contained 2,4-D and KIN or TDZ at a concentration of 1 mg/L each, because these concentrations of growth regulators in nutrient media proved to be the best for the induction of morphogenesis in this species. Before the induction of morphogenesis, plants were grown for a month with two different pre-treatments. The isoforms of the enzyme were assessed every seven days from the beginning of morphogenesis induction. During the induction of morphogenesis in F. meleagris, six isoforms of esterase were detected in the acidic and basic parts of the spectrum. In sweet potato, a larger number of esterases can be detected in embryogenic tissues compared to non-embryogenic ones [49]. The same authors also pointed out that there is no difference in the number of esterase and peroxidase isoforms in embryogenic callus tissue and globular embryos. According to Dodeman and Ducreux [50], there are no specific isoforms during embryogenesis; this was based on the analysis of seven enzymes performed at ten different stages of the development of carrot somatic embryos. However, the number of studies that indicate the specificity of isoforms that appear in the early stages of somatic embryogenesis and morphogenesis is far greater.
A greater number of esterase isoforms were observed during morphogenesis in F. meleagris in the acidic pH region in the range of pI values from 3.5 to 6.5. In a cell suspension of seven-day-old D. glomerata, a number of esterases were also detected in the acidic pH region (acidic esterase) with a smaller number of isoforms that were visible in the base pH region [17]. The pI values of these acidic esterases ranged from 3.8 to 5.8; the pI of the most acidic isoform (3.8) was similar to that of the most acidic isoform (3.5) that was found during morphogenesis induction of F. meleagris in vitro. Esterase isoforms may be specific to the genotype, as is the case with a variety of cell lines of D. glomerata. Esterases that are specific to certain stages of morphogenesis can be used as early markers and are much more interesting for research than non-specific isoforms. Some of these isoforms include the acidic esterase isoform with a pI value of 3.8 that occurs in D. glomerata [17], two acidic isoforms in cotton [51] and several acidic isoforms in sweet potato [49]. Isoforms 1 and 2 were not detected in cultures of F. meleagris seven days after the induction of morphogenesis in vitro, in a medium with 2,4-D and KIN, or on day 28 on the medium with TDZ under standard growing conditions. These isoforms specifically occurred in the later stages of morphogenesis induction: on day 28 in cultures grown on medium supplemented with 2,4-D and KIN, or on day 21 on the medium with TDZ. In addition to these two isoforms with decreased or absent activity in the initial stages of morphogenesis, there was not much difference in the esterase isoforms at different stages of this process. Esterase activity was lower at the beginning (day 7) and increased as the process of morphogenesis continued, which may indicate an active role for esterases during the initial phase of morphogenesis induction in vitro. In the case of F. meleagris morphogenesis, isoforms 2, 3, 4, 5 were present only during the process of morphogenesis. The acidic isoform with a pI value of 3.8, which also occurs in the embryogenic tissue D. glomerata, has a molecular weight of 36 kD and is linked to the cell wall through a very weak connection that allows free enzyme activity in the extracellular space and in the medium [17]. According to Bordenave and Goldberg [52], research on mung bean indicates that this is acidic pectin methyl esterase, which is also weakly bound to the cell wall and is active in the surrounding nutrient medium. Three isoforms were also found in the mentioned work, with pI values between 5.1 and 5.6, but the analysis of isoforms during embryogenesis was not done, so it cannot be said if there were differences in esterase isoform expression. Middle lamella pectin serves as a point of contact between cells, and the eventual decomposition of pectin could have an impact on the initial stages of embryonic development and morphogenesis [21]. Cell wall polysaccharide decomposition, which can serve as a trigger for the process of somatic embryogenesis, can be attributed to esterases. This hypothesis was confirmed by Mihaljević et al. [53], who noted increased activity of esterases during somatic embryogenesis in pumpkin. The increased esterase activity during F. meleagris morphogenesis after pre-treating growing bulbs at 4 °C may be explained by the increased metabolic activity of the bulb scale that spent four weeks at a low temperature, as this is how bulbs break dormancy. An accurate definition of the role of esterases in the process of morphogenesis could not be specified, and it cannot be said that they have a special role in relation to other factors. Esterase, with a smaller or larger number of isoforms, participates in the process of morphogenesis and somatic embryogenesis, and as such, it can be called a marker, but these isoforms may work together with other factors, and thereby support or facilitate the process.
During the induction of morphogenesis in F. meleagris in vitro, 11 isoforms of peroxidase were observed, which were detected in the basic pH range. Isoforms 1, 6 and 8 were not detected in the control explants. Isoform number 2 was more highly expressed in the control bulb segments than in those that had begun the process of morphogenesis in both types of inductive culture media, regardless of pre-treatment. Compared to the control, the number of isoforms increased during the first three weeks of morphogenesis induction, suggesting the possible involvement of peroxidase in the initial stages of this process. Many stress factors can affect the number of isoforms of class-III peroxidases that are present in plants [54]. Peroxidase can also affect the level of endogenous auxins and thus indirectly influence the process of somatic embryogenesis and morphogenesis [55]. Peroxidase can inhibit the activity of the enzyme IAA oxidase, which catalyzes the oxidation and thus the inactivation of IAA [56]. Some peroxidase isoforms are present only in embryogenic tissues of alfalfa in relation to non-embryogenic tissues [57]. Increased activity of peroxidase as well as of other antioxidant enzymes may result from the injury to bulbs in order to obtain explants, as shown in tulips [58]. Both esterase and peroxidase may serve as indicators of embryogenic potential, as shown in barley, before any morphological differentiation is visible [59]. It was shown in this study that enzyme analysis could predict what kinds of barley callus possess an increased or decreased capacity for embryogenesis and organogenesis.
Isoforms of peroxidase and esterases that are involved in in vitro morphogenesis in F. meleagris showed changes depending on the applied growth regulators and pre-treatment (4 °C and 24 °C).
Author contribution
MP produced and maintained in vitro cultures, designed and supervised the whole experiment. AS help with the interpretation of data and supervised writing the manuscript. SJ provided plant material. MT performed statistical analysis of date and help for experimental design. VT, MG and ZV were involved in detection of antioxidant enzymes activity.
Disclosure of interest
The authors declare that they have no competing interest.
Acknowledgments
This work was supported by the Serbian Ministry of Education, Science and Technological Development (Projects No. ON173015 and OI 172048).