1 Introduction
Leptin (Lep) is an adipose tissue-derived cytokine that reduces food intake by acting on the hypothalamus 〚1,2〛. The leptin receptor (Lepr) is a member of the class I cytokine receptor family and is encoded by a complex gene generating multiple splice variants with distinct intracellular domains 〚2,3〛. The Lepr-b isoform, which is predominantly expressed in the hypothalamus, is predicted to possess a long intracellular domain 〚4〛. This isoform has been implicated in the weight-reducing effect of leptin 〚5〛. Lepr-a, which has a short cytoplasmic domain, is the major form of leptin receptor and is expressed in several peripheral tissues 〚3,4,6〛. Originally identified as a satiety factor, leptin also exerts a wide spectrum of actions outside of the central nervous system. For example, it influences various aspects of reproduction, glucose homeostasis, hematopoiesis, the immune response and angiogenesis (for a review see 〚7〛). It has recently been shown that leptin also influences bone formation 〚8〛 and wound healing 〚9〛. Although some of the peripheral actions of leptin are probably mediated indirectly through a hypothalamic relay 〚8,10〛, a number of cells are responsive to leptin in vitro.
Lepr signalling capabilities have been investigated in reconstituted cell systems and in cell lines that naturally express Lepr isoforms. Only Lepr-b appears to activate the STAT (signal transducer activation of transcription) pathways, whereas both isoforms can signalling to IRS (insulin receptor substrates) and MAPK (mitogen-activated protein kinases) 〚11〛. Direct cross talk between leptin and insulin signalling occurs in hepatic 〚12–14〛 and muscle 〚15〛 cells. Moreover, leptin increases the production of reactive oxygen species (ROS) and activates the c-Jun kinase/stress-activated protein kinase pathway in umbilical vein endothelial cells (HUVEC) 〚16〛. These observations indicate that a variety of signalling pathways are directly activated by leptin in targets cells, accounting for the pleiotropic cellular effects of the cytokine.
In adults, adipose tissue is the main source of leptin and circulating levels of leptin serve as a measure of the degree of adiposity. The cytokine was recently shown to be synthesised in the gastric epithelium 〚17〛 and in skeletal muscle 〚18〛. Leptin expression is constitutive and regulated in the gastric epithelium. In muscles, leptin is expressed after specific metabolic stimulation by intermediates of the glucosamine pathway. In these non-adipose tissues, it has been suggested that leptin exerts a local effect rather than a systemic effect 〚17,18〛. Observations that leptin is also synthesised in the placenta 〚1〛 (for a review see 〚19〛) raised questions about the role that leptin plays during embryogenesis. Pioneering studies by Hoggard using in situ hybridisation and immunocytochemistry revealed that in the 14.5-day postcoitus mouse foetus leptin mRNA and protein are present in the placenta and in a restricted number of tissues, mainly developing bones and cartilage 〚20〛. Thus, the distribution of the leptin gene in foetal tissues appears to be strikingly different from that in adults. In addition, leptin receptors have been detected in the placenta and in the bones of the developing foetus. These correspond to the sites at which leptin is expressed, suggesting that the cytokine has autocrine or paracrine effects in these tissues 〚20〛.
This study looked at the expression pattern of Lepr at each stage of mouse embryogenesis. Moreover, we show, for the first time, that Lepr is expressed in periocular vascularised mesenchymal structures during late embryogeneis and adulthood, revealing new target tissues for leptin.
2 Materials and methods
2.1 Tissue preparation
Morphologically normal mice embryos and foetuses ranging from 9.5 to 18.5 day post-coitum (dpc) were collected from pregnant NMRI mice under anaesthetic. Two embryos were processed at each stage. The embryos were micro-dissected under the microscope, frozen in dry ice and stored at –80 °C. Cryostat serial sections (15 μm) were mounted on slides previously coated with 2% 3-aminopropyltriethoxysilane solution in acetone. Sections were fixed for 30 min in 2% paraformaldehyde in 0.1 M phosphate buffer (pH = 7.4), dehydrated with a graded ethanol series, air-dried and stored at –80 °C.
2.2 DNA probes for in situ hybridisation
Oligonucleotide probes (60 mers) were synthesised and purified by Genset (Les Ullis, France). They were 3’ end labelled with 〚α35S〛 dATP at a specific activity of approximately 7 × 108 cpm/μg. The probes were purified on biospin P 30 columns (Bio Rad). Two sense and two antisense oligonucleotides were designed to anneal to the mouse Lepr cDNA sequence (Genebank accession number: U 46135), and one sense and one antisense oligonucleotide, were designed to anneal to the rat LEPR cDNA sequence (Genebank accession number: U 52966). The six oligonucleotide probes were chosen by use of the OLIGO software with the following parameters: 55% GC content and ΔG > –4 kcal/mol for hairpins and self-pairing. FASTA and BLAST were used to compare all of the sequences to the Genebank and EMBL nucleotide sequence databases, to ensure that they were specific to the Lepr gene.
The first probe is common to all mouse Lepr isoforms (position: 608-668). The sequences are: Antisense: TGG CTC TGG GTA CCG GTA CCG GCA CAT GAC ATT CAC ATC CCC GAA GAC TGC AGT TGC ATT GGA CAG . Sense: CTG TCC AAT GCA ACT GCA GTC TTC GGG GAT GTG AAT GTC ATG TGC CGG TAC CCA GAG CCA. A second probe was chosen to match a sequence that is only transcribed in the long Lepr isoform (position: 3345–3405). The sequences are the following. Antisense: TGG AGG ATC CTG ATG TCA CTG A AC AGA CAC TGG GCT GGG AAT GTG CAC AGG ATT CCT CCG. Sense: GGC AGG AAT CCT GTG CAC ATT CCC AGC CCA GTG TCT GTT CAG TGA CAT CAG GAT CCT CCA. The third probe is common to all rat Lepr isoforms (position: 354-414). The sequences are the following. Antisense: TGA AGC CAG CGT CTT CCC TTC AGT GTT GCC TGT GAG TGC GGA GCA GTT TTG ACC TTG CTC. Sense: GAG CAA GGT CAA AAC TGC TCC GCA CTC ACA GGC AAC ACT GAA GGG AAG ACG CTG GCT TGA. The three sense Lepr oligonucleotide probes did not detect any hybridisation signal.
2.3 In situ hybridisation procedure
The procedure was previously described 〚21〛. Briefly, the hybridisation mixture contained: 50% formamide, 4 X SSC (standard saline citrate), 1 X Denhardt’s solution, 0.25 mg/ml yeast tRNA, 0.25 mg/ml sheared herring sperm DNA, 0.25 mg/ml poly A, 10% dextran sulphate, 100 mM DTT and 6 × 105 cpm/100 μl 〚α35S〛 dATP-labelled probe. Hybridisation solution (100 μl) was put onto each embryonic section. The sections were covered with a parafilm coverslip and incubated in a humidified chamber at 43 °C for 20 h. After hybridisation, the slides were washed twice in 1X SSC supplemented with 10 mM DTT for 15 min each at 55 °C, twice in 0.5 X SSC supplemented with 10 mM DTT for 15 min each at 55 °C, and once in 0.5 X SSC supplemented with 10 mM DTT for 15 min at room temperature. The sections were then dipped in water, dehydrated through a series of graded ethanol concentrations and exposed to Amersham Betamax X-ray films for 4 days and then to Kodak NTB2 photographic emulsion for 2 months at +4 °C.
3 Results
The probe common to all mouse Lepr isoforms detected specific in situ hybridisation signals in 12.5 dpc embryos. At this early stage of development, the yolk sac was strongly labelled (Fig. 1A, B). The dense mesenchyme tissues, which subsequently contribute to the formation of the connective tissues and smooth muscles of the larynx and laryngeal cartilage, were labelled. The neural crest-derived mesenchyme, which later differentiates into the inner component of the tooth primordium, was also strongly labelled. The spinal cord of the dorsal part of the developing neural tube was specifically labelled and the ectoderm was more faintly labelled (Fig. 1C, D). Lepr gene expression was also detected in several other structures of the developing central nervous system including the leptomeninges, the choroid plexuses, the cerebellum, the roof of the mid-brain and the ependyma. The same probe also detected strong labelling of the choroid plexuses in sections of adult mouse brain tissue. Finally, a cluster of cells located in the lumen of a vessel was strongly labelled (Fig. 1E, F).
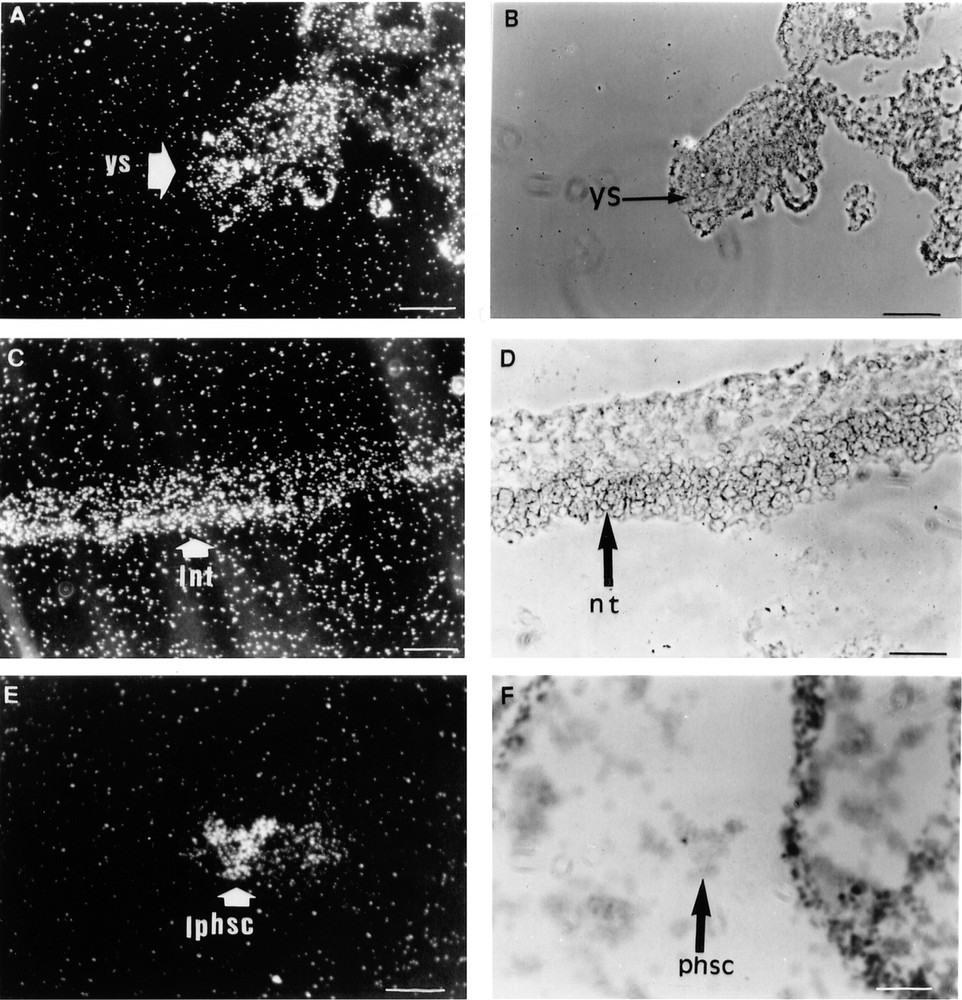
Three sections of a 12.5 dpc mouse embryo are shown. In situ hybridisation was performed as described in the Materials and methods section with the probe common to all mouse Lepr isoforms. A–B: 10 mm bar = 10 μm. A. Dark field aspect of the Lepr cellular hybridisation signal arising from the yolk sac (ys). B. Bright field histology of the same section. C–F: 10 mm bar = 20 μm. C. Dark field aspect of the Lepr cellular hybridisation signal arising from the neurectoderm (nt). D. Bright field histology of the same section. E. Dark field aspect of the Lepr hybridisation signal arising from putative hematopoietic stem cells (phsc) located in the lumen of a differentiating vessel. F. The same signal in bright field 1. lnt: labelling of neurectoderm; lphsc: labelling of putative hematopoietic stem cells.
At 13.5 dpc, the ectoderm, leptomeninges and choroid plexuses displayed a strong cellular in situ hybridisation signal. A landmark of the 13.5 dpc embryonic stage was the labelling of the mesenchymal condensations surrounding the developing ribs, vertebrae and bones of the hind limbs. The Lepr expression pattern observed at 13.5 dpc was maintained throughout the subsequent developmental stages until birth.
At 17.5 dpc, the Lepr gene was predominantly expressed in the mesenchymal tissues adjacent to the tracheal epithelium and in the outer smooth muscles surrounding the trachea (Fig. 2A, B). The cartilage surrounding the differentiating bones of the digits and hair bulbs was also strongly labelled (Fig. 2C, D). When the probe specific for mouse long isoform Lepr-b was used for in situ hybridisation, the periosteum of the occipital bones (Fig. 3A, B) and the cartilage undergoing ossification (Fig. 3C–F) were strongly labelled. Lepr-b was also strongly transcribed in intervertebral disks and ligaments (Fig. 4A, B) and in musculoaponeurotic tissues (Fig. 4C–F). This pattern of expression was maintained until birth.
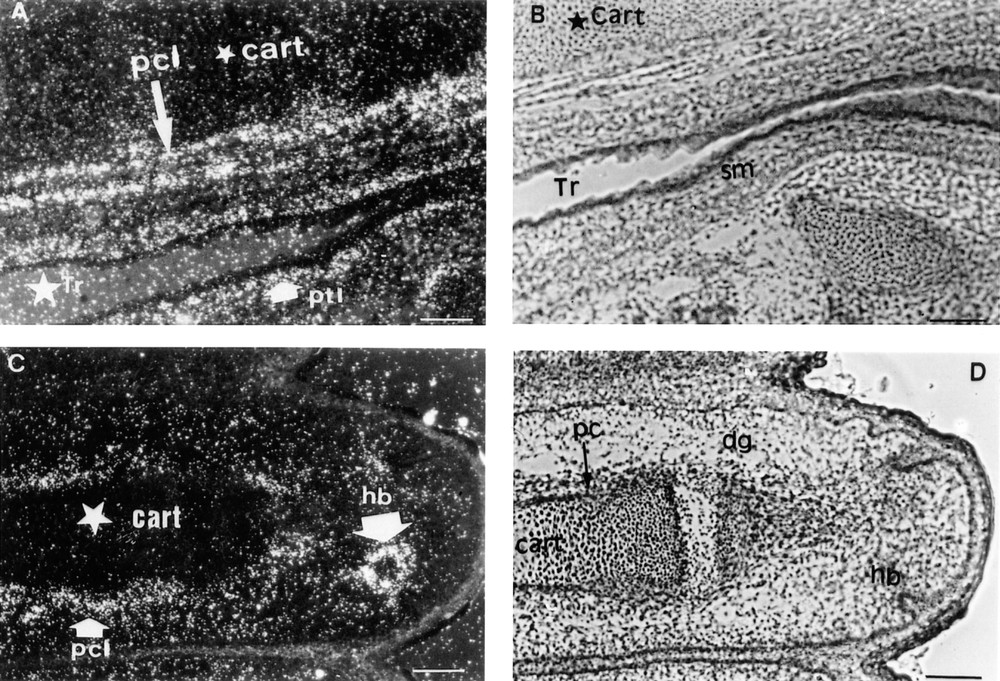
Two sections of a 17.5 dpc mouse embryo are shown. In situ hybridisation was performed as described in the Materials and Methods section with the probe common to all mouse Lepr isoforms. A–D: 10 mm bar = 10 μm. A. Dark field aspect of the Lepr cellular hybridisation signal arising from the cartilage (cart) and smooth muscles (sm) surrounding the trachea (Tr). pcl: perichondral labelling. ptl: paratracheal labelling. B. Bright field histology of the same section. C. Dark field aspect of the Lepr cellular hybridisation signal arising from the perichondrum around the cartilage of a phalange and from cells around a hair bulb (hb). D. Bright field histology of the same section. dg: digit. pc: perichondrum.
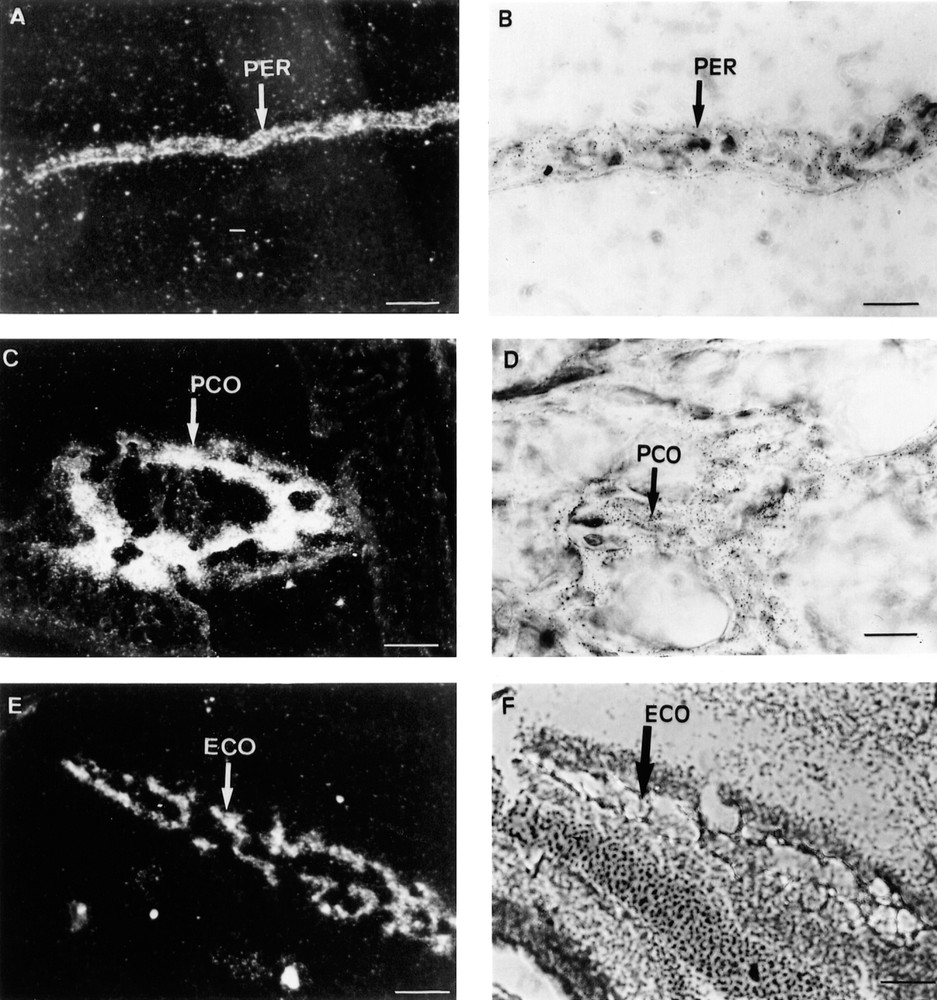
Three sections of a 17.5 dpc mouse embryo are shown. In situ hybridisation was performed as described in the Materials and Methods section with the probe recognising the long mouse Lepr-b isoform. A, C, E, F: 10 mm bars =10 μm. B, D: 10 mm bars = 40 μm. A. Dark field aspect of the Lepr-b cellular hybridisation signal arising from the occipital periosteum (PER). B. Bright field histology of the same section at a higher magnification. C & E. Dark field aspects of the Lepr-b cellular hybridisation signals arising from perichondral (PCO) and endochondral (ECO) ossification areas. D & F. Bright field aspects of the same sections.

Three sections of a 17.5 dpc mouse embryo are shown. In situ hybridisation was performed as described in the Materials and Methods section with the probe recognizing the long mouse Lepr-b isoform. A–E: 10 mm bars = 10 μm, F: 10 mm bar = 40 μm. A. Dark field aspect of the Lepr-b cellular hybridisation signal arising from the intervertebral discs (IVD). B. Bright field histology of the same section. C & E. Dark field aspects of the Lepr-b cellular hybridisation signals arising from longitudinal and transversal sections of aponeurotic laminae (Ap). D & F. Bright field aspects of the same sections. Ve = vertebra.
At 17.5 dpc, a faint labelling was detected in the choroid and the sclera of the developing eye. This unexpected observation led us to assess Lepr expression in adult eye. Therefore, sections of adult rat eye were submitted to in situ hybridisation with a probe common to all rat Lepr isoforms. Surprisingly, strong signals arose from the sclera and fainter labelling was detected in the corneal stroma (Figs. 5A, B, E and F). The Lepr gene was also highly expressed in the connective tissue of the limbus area and in the outer layer of the choroid, which contains large vessels and was more intensely labelled than the inner layer, which mostly contains small vessels. In contrast, the conjunctival and the corneal epithelia were not labelled and no signal was detected in the neural retina, the retinal pigment epithelium (Fig. 5B, F) or in the Bruch’s membrane. No major in situ hybridisation signals were detected with the sense probe (Fig. 5C, D).

Three sections of an adult rat eye are shown. In situ hybridisation was performed as described in the Materials and Methods section with the probe common to all rat Lepr isoforms (A, B, E, F) and with the sense probe (C, D). A. 10 mm bar = 40 μm. B, E, F: 10 mm bar = 10 μm. C, D: 10 mm bar = 20 μm. A. Bright field aspect of the Lepr cellular hybridisation signal arising from the choroid (CHR), around choroidal vessels (CHRV). B. Bright field histology of the same section at a lower magnification. nr: neuroretina; scl: sclera; cjmb: cunjuntiva. C. shows the lack of cellular hybridisation signal in the choroid with the sense probe. D. Dark field aspect of the same section. F. Dark field aspect of the Lepr hybridisation signal arising from the choroid and the sclera. E. Bright field aspect of the same section.
4 Discussion
This study showed that the Lepr gene is expressed during mouse embryogenesis, starting at 12.5 dpc, and is still expressed at birth. Although no strong in situ hybridisation signal was observed in 9.5 dpc embryos, Lepr may be expressed at low levels at this, or earlier embryonic stages.
Lepr transcripts were detected in several regions of the developing nervous system in 12.5 dpc embryos, consistent with previous observations 〚20〛. The presence of Lepr in neural tissues at early stages of embryonic development suggests that leptin is involved in the growth and differentiation of the central nervous system. It is noteworthy that the brains of ob/ob mice, which are genetically leptin-deficient, and fa/fa rats or db/db mice, which bear mutations in the Lepr gene, are markedly reduced in size 〚22–24〛. Alteration of a potential leptin effect on brain development in utero might be responsible for this defect, which can be corrected by leptin injections in adult ob/ob mice 〚25,26〛.
At 12.5 dpc, Lepr gene expression was detected in cells of the yolk sac and in clearly identified cells in the lumen of a differentiated blood vessel that was probably derived from hematopoietic stem cells. The simultaneous and early detection of Lepr mRNA in these cells suggests that leptin and its cognate receptors have a precocious role in hematopoiesis. Our observations are consistent with several recent studies demonstrating such a role for leptin. Leptin regulates the proliferation and differentiation of yolk sac cells, foetal liver cells and hematopoietic precursors of the erythrocytic and myelopoietic lineages in vitro 〚27〛. Synergistic effects of leptin with erythropoietin (EPO), stem cell factor (SCF), c-Kit ligand (KL) and steel factor (SLF) have been reported 〚27–29〛. In addition, it was recently shown that db/db mice are deficient in lymphopoiesis, because their bone marrow and peripheral blood contain reduced levels of lymphocytes and because their blood lymphocytes fail to recover fully following irradiation 〚30〛.
A landmark during embryogenesis is the expression of Lepr in mesoderm-derived tissues, including cartilage and smooth muscles surrounding the larynx and the trachea, mesenchymal condensations within structures undergoing ossification and the aponeurotic laminae of several muscles. Foetal mesenchymal tissues were labelled with both the common probe and with the probe specific for the long Lepr-b isoform, in agreement with previous observations 〚20〛. This pattern of tissue distribution suggests that leptin is involved in the formation of bones, cartilage and muscles. Consistent with this hypothesis, leptin has been shown to stimulate the proliferation of C3H10T1/2 mouse embryonic fibroblasts, which can differentiate into muscle cells, adipocytes or chondrocytes 〚31〛. Moreover, a recent study demonstrated that leptin acts on human marrow stromal cells to enhance their differentiation into osteoblasts and to inhibit their differentiation into adipocytes 〚32〛. In addition, when adult ob/ob mice are given leptin, the reduced femur length characteristic of these mice is corrected, suggesting that the cytokine plays a role in bone formation in adult mice 〚33〛. Further studies are needed to identify the molecular mechanisms involved in the potential osteogenic action of leptin, possibly by altering the expression of bone morphogenetic proteins (BMP) 〚34〛. Moreover, BMPs have been implicated in the formation of ventral mesoderm and hematopoietic precursors 〚35〛. Therefore, these factors could be a common molecular link mediating leptin action on cartilage/bone formation and hematopoiesis during embryogenesis.
Unexpectedly, Lepr was also detected in teeth primordium and in hair follicles in 12.5 and 13.5 dpc embryos, as already reported at 14.5 dpc 〚20〛. The physiological relevance of these observations is currently unclear because leptin signalling deficient rodents do not display any marked alteration in teeth and hair formation.
Similarly, the unexpected expression of Lepr in the vascularised connective tissues surrounding the retina is puzzling. There are at least two hypotheses to explain this phenomenon. Firstly, leptin is a potent angiogenic factor 〚36,37〛 that may participate to the development of choroidal vessels, which is of major importance for eye growth. Alternatively, the role of leptin might be to stimulate immune response, particularly in the limbus. This region is rich in immune cells, which may be directly stimulated by leptin resulting in an increase in their production of proinflammatory cytokines, as demonstrated in blood macrophages and T-lymphocytes 〚38,39〛. Elevated cytokine production may be the primary cause of the marked corneal neovascularisation induced by corneal leptin pellet implantation in rat eye 〚36〛. It remains to be determined whether the in situ hybridisation signal detected by the common probe represents the long or a short Lepr isoform and which signalling pathway mediates the potential action of leptin in the development or function of the eye. It is tempting to predict that functional and/or anatomical ocular defects might be detected in individuals with disrupted leptin signalling.
In summary, our data demonstrate that the leptin receptor gene is mainly expressed in mesoderm-derived tissues during embryogenesis. They suggest that this cytokine is specifically involved in the differentiation and growth of mesenchymal tissues. Leptin, which was discovered on the basis of a particular biological dysfunction in ob/ob mice 〚1〛, now appears to exert a variety of actions during foetal development and adulthood. Our study widens the physiological fields in which leptin might fulfil different roles through specific signalling pathways, some of which have yet to be identified.
Acknowledgements
We thank Prof. G. Couly for helping us to ascertain the nature of several embryonic structures. We thank Retina France–AFRP for continuous financial support. This research was supported by a PROGRES grant from INSERM to M.G-M.
Version abrégée
La leptine est une cytokine produite par le tissu adipeux, qui agit au niveau hypothalamique pour réduire la prise alimentaire. Ses récepteurs (Lepr) appartiennent à la famille des récepteurs de cytokine de classe I, qui possèdent un domaine trans-membranaire unique. Plusieurs isoformes Lepr, provenant de l’épissage alternatif d’un même gène, se distinguent par la nature et la longueur de leur domaine intracellulaire. L’isoforme longue (Lepr-b) est impliquée dans l’effet anti-obésité de la leptine. L’isoforme Lepr-a, ayant un domaine intracellulaire plus court, est la plus abondante et la plus ubiquiste. Outre son effet satiétogène, la leptine exerce une grande variété de fonctions, certaines étant relayées par un effet central, et d’autres par un effet direct sur les tissus périphériques. En accord avec l’existence de plusieurs isoformes de Lepr, la leptine active différentes voies de signalisation, outre la voie Jak-STAT propre aux cytokines.
Plusieurs observations indiquent que la leptine est produite par des tissus autres que le tissu adipeux, dont l’épithélium gastrique et les muscles squelettiques dans certaines conditions métaboliques. Dans ces tissus, la leptine pourrait exercer un rôle local, paracrine ou autocrine. La leptine est également synthétisée par le placenta, ce qui soulève la question de son rôle au cours de l’embryogenèse. Chez l’embryon de souris, une étude antérieure montre que la leptine est exprimée particulièrement dans les os et les cartilages, ce qui est totalement différent de sa répartition tissulaire chez l’adulte.
Dans ce travail, nous avons réalisé la cartographie d’expression du gène Lepr au cours de l’embryogenèse de souris par hybridation in situ. Nous avons utilisé deux sondes oligonucléotidiques, l’une reconnaissant toutes les isoformes de Lepr et la seconde reconnaissant spécifiquement Lepr-b. Nos résultats montrent la présence de transcrits Lepr dès le stade 12,5 post-coïtum. Le sac vitellin et diverses structures du système nerveux central, dont les leptoméninges et le plexus choroide, sont marqués à ce stade. Aux stades ultérieurs, un fort marquage apparaît dans les cartilages, les zones d’ossification et les aponévroses musculaires. Ce profil d’expression se maintient jusqu’à la naissance. De plus, le même profil d’expression est obtenu avec la sonde spécifique de Lepr-b, qui reconnaît des transcrits dans le périoste des os occipitaux, les disques et ligaments intervertébraux et diverses aponévroses musculaires.
Au cours de cette étude, nous avons détecté un marquage spécifique des transcrits Lepr au niveau de l’œil, chez un embryon au stade 17,5 post-coïtum. Cette observation inattendue nous a conduit à rechercher la présence de transcrits au niveau de l’œil chez le rat adulte. Ainsi, nous montrons pour la première fois que Lepr s’exprime fortement dans les tissus vascularisés, choroïdes et sclère, entourant l’œil.
Cette étude révèle que Lepr est exprimé au cours de l’embryogenèse dès le stade 12,5 post-coïtum et jusqu’à la naissance. Le fait que Lepr soit exprimé précocement dans les tissus neuronaux suggère que la leptine puisse jouer un rôle dans la croissance et la différenciation du système nerveux central. Chez l’adulte, le cerveau des souris mutantes ob/ob, dépourvues de leptine, et db/db, dépourvues de Lepr, est notablement réduit en taille. Une altération de l’effet potentiel de la leptine in utero pourrait rendre compte de cette anomalie. Par ailleurs, on sait que la leptine régule la différenciation et la prolifération des précurseurs hématopoïétiques chez l’adulte. Nos observations d’une expression de Lepr dans le sac vitellin suggèrent que cette cytokine puisse exercer une fonction de cette nature au cours de l’embryogenèse. Plus inattendue est la présence forte et constante au cours de l’embryogenèse de Lepr dans les cartilages et les os en développement. Une étude récente montre que la leptine est capable d’augmenter la différenciation des cellules stromales de la moelle osseuse en ostéoblates. D’autre part, il est à noter que les souris ob/ob présentent des anomalies morphologiques au niveau du fémur, qui peuvent être corrigées par administration de la cytokine. Ces observations suggèrent que la leptine puisse avoir un rôle ostéogénique au cours de l’embryogenèse et du développement post-natal. Les mécanismes moléculaires impliqués dans cette action potentielle restent à décrypter.
L’un des résultats les plus inattendus de ce travail révèle la forte présence de Lepr dans les tissus vascularisés entourant l’œil, ce qui n’avait jamais été montré auparavant. Au vu de plusieurs études récentes démontrant une forte capacité angiogénique de la leptine, on peut formuler l’hypothèse que cette cytokine participe au développement des vaisseaux choroidiens, d’importance majeure pour le développement de l’œil. D’autre part, cette région étant riche en cellules immunitaires, il est possible également que la leptine module leur fonction comme cela a été démontré chez l’adulte. L’action potentielle de la leptine dans le développement et les fonctions oculaires méritent d’être étudiées plus avant.
Découverte sur la base d’une fonction spécifique de régulation de la prise alimentaire, la leptine est maintenant impliquée dans une large variété de fonctions. Nos observations révèlent de nouveaux tissus cibles de la leptine chez le fœtus et chez l’adulte et élargissent le champ des domaines physiologiques dans lesquels la leptine est impliquée.