Version française abrégée
La perception des substances microbiennes joue un rôle prépondérant dans les interactions des plantes avec les microorganismes. En particulier, les plantes possèdent des systèmes de perception d'éliciteurs dérivés des champignons pathogènes leur permettant de mettre en œuvre des réactions de défense. Des fragments oligosaccharidiques, des glycopeptides, des peptides et des composés lipidiques induisent des réactions cellulaires précoces, notamment des migrations ioniques : influx de Ca2+ et influx de H+, associé à un efflux de K+. Dans la plupart des cas, ces changements des propriétés de perméabilité du plasmalemme se traduisent par une dépolarisation du potentiel transmembranaire.
À partir de mesures de variations de pH du milieu d'incubation de tissus pulvinaires et d'enregistrements électrophysiologiques intracellulaires dans des cellules motrices de pulvini de Mimosa pudica L., des caractéristiques de la perception de l'ergostérol (composé spécifique de la membrane fongique) ont été comparées à celles du chitosane (composé trouvé dans la paroi fongique), connu pour induire des réactions d'élicitation.
L'addition d'ergostérol (10 μM en concentration finale) au milieu d'incubation de tissus pulvinaires induit une rapide augmentation du pH extracellulaire après un temps de latence de 5,7±1,5 min et d'amplitude maximale de 0,12±0,03 unité pH en 30 min. L'addition de chitosane (125 μg
L'ergostérol induit une modification de la différence de potentiel transmembranaire originale, puisqu'il induit une hyperpolarisation du potentiel membranaire, alors que les autres stérols testés n'ont pas d'effet significatif et que le chitosane, au contraire, provoque une dépolarisation membranaire. Un processus de désensibilisation spécifique a également été mis en évidence, puisque à la suite d'une première hyperpolarisation, les cellules ne réagissent pas à une seconde application du produit (même à une concentration double), alors qu'elles se dépolarisent sous l'effet du chitosane. D'une manière comparable, une seconde application de chitosane (à double dose) ne provoque pas de nouvelle dépolarisation, tandis que l'ergostérol induit l'hyperpolarisation habituellement provoquée.
Les modifications des propriétés membranaires des cellules motrices pulvinaires, induites par l'ergostérol et indiquées par les variations précoces des flux de protons et du potentiel transmembranaire, sont dépendantes de la dose dans la gamme de concentration utilisée, la concentration seuil se situant à 1 μM.
L'influx de H+ ainsi noté résulte le plus probablement d'une inhibition de l'H+-ATPase plasmalemmique, enzyme active dans le modèle utilisé, comme le montre l'action de la fusicoccine. Néanmoins, une activation du système redox membranaire (activation de la NADPH oxydase) n'est pas à écarter. Le résultat de ces modifications d'activité enzymatique est une réduction du gradient électrochimique de protons à travers le plasmalemme ; en particulier, la suppression du gradient de pH peut servir de signal de transduction. Cependant, les résultats électrophysiologiques obtenus suggèrent que les mécanismes ioniques impliqués après la perception de l'ergostérol et du chitosane doivent différer fondamentalement.
Après traitement aussi bien par l'ergostérol que par le chitosane, il apparaı̂t un phénomène similaire de désensibilisation spécifique, qui présente des caractéristiques observées dans les processus d'adaptation des systèmes animaux. Les données obtenues montrent que les systèmes de perception sont spécifiques de l'ergostérol comparativement aux autres stérols testés et argumentent par ailleurs l'existence d'un récepteur identifiable pour le chitosane. En conclusion, le système de perception décrit pour l'ergostérol peut s'avérer opérationnel dans les interactions plantes–pathogènes, puisque ce stérol a été trouvé dans des tissus végétaux à la suite d'une colonisation par des champignons pathogènes ou ectomycorrhiziens.
1 Introduction
Perception of microbial substances by host organisms plays a major role in the interaction of plants with microbes. In particular, plants possess sensitive perception systems for elicitors derived from pathogenic fungi. Oligosaccharide fragments, glycopeptides, peptides, fatty acids and ergosterol have been identified as fungal compounds showing elicitor activity in various plant systems [1].
The perception of these elicitors induces a variety of defence responses [1,2]. The earliest response of plant cells to elicitors is indicated by changes in plasma membrane properties, including influx of H+ (frequently shown by an alkalinization of the growth medium). This proton influx may be associated with K+ efflux and Ca2+ influx [3,4]. Several works have also reported rapid changes in plasma membrane potential in response to elicitors. In many cases, depolarization of the transmembrane potential was noted [5–12].
Little attention has been paid to the biological events triggered by ergosterol applied to plant cells. However, it has been shown that a factor released from Cladosporium fulvum spores and identified as ergosterol was perceived by a suspension of tomato cells in culture [13]. It thus induced alkalinization of the growth medium. This reaction showed a refractory period identical to that noted after application of chitin oligo-saccharides derived from yeast cells [14].
Based on measurements of pH variations and transmembrane electro-physiological recordings, we describe here some characteristics of the perception system of pulvinar motor cells for ergosterol and compare them with that noted after application of chitosan, a derivative from chitin, a major component of fungal cells walls. Chitosan has been shown to produce the characteristic reactions of elicitation and thus is used here as reference compound [15,16].
2 Materials and methods
2.1 Plant-growth conditions
The sensitive plants (Mimosa pudica L.) were grown in an organic compost watered daily. They were kept in climate-controlled chambers at 27.5±0.5 °C and 65±5% relative humidity. Illumination was regulated to give 16 h of light (photophase: 06.00–22.00 h) provided by fluorescent tubes (mixing Osram fluora and Osram daylight types) providing a photon flux density (400–700 nm) of 36 μmol
2.2 Measurement of pH variations
Primary pulvini of Mimosa pudica (650 mg) were divided in transverse sections (about 0.2 mm) and pre-incubated for 1 h in 6 ml medium M: 0.5 mM CaCl2, 0.25 mM MgCl2. The sections were then transferred to 6 ml fresh medium M. Variations of the pH in the incubation medium were read on a pH-meter (Expandomatic SS2; Beckman, Roissy, France) provided with a combined electrode (Futura micro combination, Beckman) and linked to a potentiometric recorder. The incubation medium was aerated by a rod stirrer (Metrohm E 622; Vélizy-Villacoublay, France). In order to quantify the amount of mobilized protons, titration was made on 2 ml of the incubation medium with NaOH at 5×10−3 N.
2.3 Electrophysiological measurement
The transmembrane electrical potential was measured by the classical method on the Mimosa pudica pulvinar motor cell, which gives very distinct bioelectrical variations under various types of stimuli. Briefly, capillaries provided with an internal microfibre (GC 150F15; Clark Electromedical Instruments, Panghourne, UK) were drawn to microelectrodes (tip diameter <1 μm, tip resistance from 5 to 30 M
2.4 Chemicals
All chemicals were purchased from Sigma-Aldrich Chimie SARL, Saint-Quentin-Fallavier, France. Sterol solutions were prepared in stock solutions at 2 mM in absolute ethanol and used generally at a final concentration of 10 μM (0.5% ethanol final concentration). Chitosan from crab shells was prepared according to a method previously described [19] and generally used at a final concentration of 125 μg
3 Results
3.1 pH variations in the incubation medium of pulvinar tissues
A previous work has presented in detail the pH variations recorded in the bathing medium of pulvinar tissues [20]. Thus, it has been noted that after a transient alkalinization lasting about 1 h, the medium became constantly acidic until an equilibrium around pH 4.5 was attained after 8 h of experimentation. Addition of effectors in the course of the acidification phase at an appropriate time (i.e., 3 h after the start of experiment) allows us to evidence the promoting or inhibitory effect of the tested effectors on H+ efflux in a discriminating way.
In such conditions, addition of ergosterol to the incubation medium of pulvinar tissues induced a rapid increase in extracellular pH (Fig. 1A). When treated with ergosterol at 10 μM, the process started after a lag of 5.7±1.5 min and reached a maximum, 0.12±0.03 pH unit above the initial value, after about 30 min. Addition of chitosan induced a similar pH variation. When treated with this latter compound at 125 μg

Representative curves of the time course of pH variations in the bathing medium of pulvinar slices of Mimosa pudica primary pulvini. A. Following addition of chitosan at 250 μg
Representative curves of the time course of pH variations in the bathing medium of pulvinar slices of Mimosa pudica primary pulvini. A. Following addition of chitosan at 250 μg
Data in Table 1 show that extent of proton influx and duration of pH changes were dose-dependent in the concentration range assayed, the threshold value being 1 μM in our experimental model.
Amount of protons absorbed and duration of pH variation following application of ergosterol at various concentrations. Calculations on H+ absorption were made 30 min after addition of the compound (mean ± SD; n=5)
Ergosterol (μM) | H+ absorbed (neq/g FW) | Duration (min) |
1 | 53 ± 45 | – |
5 | 259 ± 35 | 52 ± 8 |
10 | 493 ± 98 | 78 ± 5 |
20 | 697 ± 96 | 96 ± 6 |
Fig. 1B shows that pulvinar cells stimulated once with 10 μM ergosterol entered a refractory state, since the reaction triggered by a second application of this sterol (even at 20 μM) was extremely weakened. By contrast, the cells remained fully responsive to a chitosan application.
When the cells were first exposed to cholesterol, they still reacted to a further application of ergosterol, indicating that none of them interfered with ergosterol perception (Fig. 1C). The same result (not shown) was obtained after treatments with stigmasterol and sitosterol.
The same mode of reaction was obtained with chitosan: a second application of the compound (×2) did not trigger a noticeable pH rise, whereas ergosterol was perceived as noted above (Fig. 1D).
3.2 Modifications of transmembrane potential
Fig. 2A shows that 10 μM ergosterol induced a hyperpolarization of the transmembrane potential in motor cells, whereas chitosan induced a depolarization of the PD. The modification of membrane potential started after a lag of 4.8±1.8 min for ergosterol and 2.5±0.5 min for chitosan. Data given in Table 2 show that hyperpolarization induced by ergosterol was dose-dependent in the range 1–20 μM, the threshold value being considered as 1 μM.
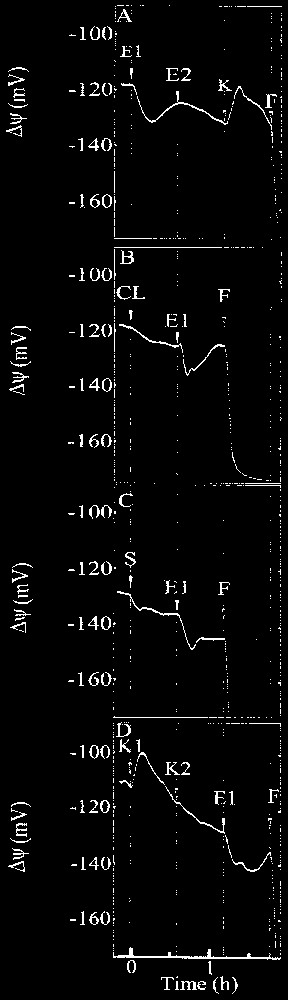
Typical recordings of transmembrane potential variation in extensor motor cells of Mimosa pudica primary pulvini induced by application of (A) 10 μM ergosterol (E1) followed by a subsequent treatment with 20 μM ergosterol (E2) and chitosan at 125 μg
Typical recordings of transmembrane potential variation in extensor motor cells of Mimosa pudica primary pulvini induced by application of (A) 10 μM ergosterol (E1) followed by a subsequent treatment with 20 μM ergosterol (E2) and chitosan at 125 μg
Modifications of transmembrane potential induced by ergosterol at increasing concentrations as indicated, various other sterols at 10 μM, 0.5% ethanol and chitosan at 125 μg
Compound | n | ΔΨ (mV) | SD (mV) |
Ergosterol (1 μM) | 5 | −2.0 | 1.4 |
Ergosterol (5 μM) | 6 | −6.2 | 2.3 |
Ergosterol (10 μM) | 11 | −15.5 | 5.3 |
Ergosterol (20 μM) | 6 | −24.8 | 3.8 |
Cholesterol | 8 | −3.1 | 3.0 |
Stigmasterol | 10 | −1.7 | 3.0 |
Sitosterol | 5 | −1.4 | 3.6 |
Ethanol | 5 | −3.2 | 1.6 |
Chitosan | 7 | +15.9 | 3.6 |
A refractory state was also found in this bioelectrical process since, following a first hyperpolarization induced by ergosterol, cells did not react to a second application of this compound (×2), whereas the cells remained responsive to a chitosan application. A response specificity was also found, since cholesterol, sitosterol and stigmasterol did not induce great potential modifications of the PD (Table 2) and did not interfere with ergosterol perception (Fig. 2B and C). As shown in Fig. 2D, a second application of chitosan (×2) did not induce subsequent depolarization, whereas ergosterol triggered usual hyperpolarization. Fusicoccin (10 μM) added at the end of each experiment triggered a strong hyperpolarization of the cell membrane: ΔΨ reached an equilibrium around −180 mV in 30 min (Fig. 2B). This effect ascertains the bioelectrical viability of the impaled motor cell.
4 Discussion
Many fungal molecules secreted or liberated from wall and membrane surfaces may potentially play a role in the recognition process and in the induction of plant defence responses. These molecules of different structure (oligosaccharides, peptides, lipids) have in common the triggering of early events at the membrane site, indicating that their receptors may be located at this level. Changes in plasma membrane properties, shown by modifications of ion fluxes (including H+ flux), are among the earliest responses of plant cells to microbial elicitors [3].
Following application of ergosterol, the pulvinar motor cells reacted by increasing proton influx indicated by a transient alkalinization of the incubation medium. In most cases, such proton influx followed inhibition of H+-ATPase [19,21] playing the role of a switch between the elicitor recognition and the pathogen defence-signalling pathways. This inhibition reduces the proton electrochemical gradient across the plasma membrane and, in particular, the collapse of ΔpH may be sufficient by itself to serve as signal transduction [22]. This assumption was based on the effect of orthovanadate, an inhibitor of plasma membrane ATPase, which can act as elicitor [23,24]. In a previous work [20], we have shown that addition of FC, a fungal toxin activating specifically the plant H+-ATPase, induced an acidification of the bathing medium of pulvinar tissues, bearing the pH to an equilibrium around 4.5 in 2 h. This observation demonstrates that acidification in this system is related to H+-ATPase activity. By comparing H+ secreting activity between central cylinder tissues and parenchyma motor tissues of the pulvini, it was shown previously that the observed acidification of the medium mainly comes from the cells constituting this latter tissue.
However, it should also be remembered that this acidification could also be achieved by activation of the plasma membrane redox system through activation of NADPH oxidase. Such a hypothesis has been put forward in the case of action of cryptogein triggering cytoplasmic acidification and extracellular alkalinisation of tobacco cells [12]. This aspect needs further investigations, since recent data showed that inhibition of NADPH oxidase resulted in inhibition of oxidative burst, but without modification of the induced alkalinization [25].
Perception of ergosterol, visualized by the rise of pH in the incubation medium, showed similar characteristics in pulvinar motor cells to that noted in tomato cells, except that it was less sensitive [13]. This may be due to the presence in our experimental model of cell walls able to trap exogenous compounds. This trapping may imply therefore, as a consequence, that higher external compound concentration was needed to induce the observed biological effects. Another explanation is that only cells at the surface of pulvinus slices, in direct contact with the incubation medium were able to perceive the ergosterol signal. A third hypothesis is that pulvinar cells possess a lower density of receptors on their membrane surface compared with cultured tomato cells.
It is stressed that the modification of H+ flux in pulvinar motor cells following treatment by ergosterol was similar to the chitosan response. A small difference was however found in the lag phase of the reaction, shorter in the case of chitosan treatment.
By contrast, the induced effect by the compounds was completely different on the membrane potential, since ergosterol hyperpolarized the cell membrane, whereas chitosan depolarized it. This result indicates that the underlying processes differ basically in both cases and, in particular, the H+ influx cannot be explained in the same way. This also suggests strongly that, according to the perception of the compounds, the underlying ionic mechanisms are different. Indeed, in the case of ergosterol, the induced transmembrane potential variation and H+-flux modification showed a similar latency period, lasting only a few minutes, and presented similar characteristics in the respective time course of the phenomena during the first phase (30 min). However, the causal link between the two events is not direct, since a H+ entry in cells might correspond with a depolarization of the membrane potential. Therefore, we can postulate that ergosterol triggers another ion disturbance, either a release of a cation or an uptake of an anion. In this respect, the induced ionic currents have to be further investigated. This observed hyperpolarization is not related to the very specialized feature of the parenchyma motor cells, since a similar general response was also recorded in common parenchyma cells of Mimosa pudica cotyledons (data not shown).
After ergosterol treatment, it can be noted that a desensitization phenomenon takes place at the two levels of observation used in this work. This phenomenon appears similar to receptor adaptation in animal systems. The same remark can be made concerning the action of chitosan and thus argues for the existence of a receptor for this compound. In a previous work [26], the possibility of such an interaction of chitosan with a receptor was discarded by considering the activity of the compound as a function of its degree of polymerization and extent of N-acetylation, suggesting merely an interaction of the compound with regularly spaced negative charges on the plasma membrane.
The perception system described here may be relevant in plant–pathogen interaction by considering the fact that ergosterol has been quantified in plant tissues following fungal colonization both by pathogens and ectomycorrhizal fungi [27].
5 Conclusion
The data presented here extend previous experiments suggesting that plant cells possess detection system for characteristic compounds from fungi, particularly ergosterol. The sensitivity of the recognition differs depending on the plant considered. The detection systems are specific for a given compound, either a membrane component (ergosterol) or cell wall component (chitosan). The sterol detection system is specific in plants for ergosterol, since no particular reaction was observed following application of cholesterol and other ‘endogenous’ plant sterols (sitosterol, stigmasterol).
The different behaviour induced by chitosan and ergosterol on membrane potential, respectively depolarization and hyperpolarization, shows that the two molecules modify different ionic gradients following their perception at the cell surface.
Acknowledgements
This work was supported by the ‘Conseil régional Poitou-Charentes’ (France).