1 Introduction
The Sfax region belongs to the central eastern part of Tunisia, 270 km from the capital. Nowadays, it accommodates one of the most important industrial complexes, among which the (SIAPE) factory constitutes the main pollution source. It is a phosphate fertilizer-producing factory, located in the southern suburb of Sfax, that converts crude phosphate with a high fluoro-apatite [Ca5(PO4)3F] content into a granule phosphate fertilizer easily assimilated by plants. During the phosphate attack by sulphuric and phosphoric acids, fluoride compounds such as HF, H2SiF6, and CaF2 are given off by the factory chimney [1–3]. In addition, analysis of the air surrounding the factory showed that fluoride air contents oscillate between 3 and 12 μg dm−3 day−1 [4,5]. Since the installation of the SIAPE, the agrosystems in its surroundings have undergone increasing degradation. In the vicinity of the factory, some local fruit species, such as pomegranate and almond trees, grow in a naked landscape and are daily subject to fluorine air pollution. For the almond tree, which has often been considered as sensitive to fluoride pollution [6,7], we did not observe any flowers or fruit within a radius of 1.5 km from the factory. However, for the pomegranate tree, classified among resistant fruit species to fluoride pollution [7], some ecotypes thrive and fructify in the most polluted area.
In an attempt to explore some adaptation strategies adopted by resistant as well as sensitive fruit species to survive in such restrictive conditions, and to explain different plant responses to air pollutants, we studied, throughout the growing season, the variation of fluorine concentrations jointly with those of some mineral elements, such as calcium, magnesium and phosphorus.
2 Materials and methods
2.1 Description of the study site
The study region is a low plain along the Mediterranean sea-side lined up with series of 100-m-high hills and situated about 20 km along the coast. It is submitted both to continental dry winds and to highly humid sea coastal winds. The prevailing winds from the southeastern sector have a frequency of (25.5%), and those of the southwestern sector appear with a frequency of about 16.25%. However, northwest and northeast winds occur with intermediate frequencies.
2.2 Plant material and sampling techniques
The studied species, 15 years old, were marked in land plots close to the SIAPE factory. They consist of 2 fruit species: pomegranate (punica granatum), and almond trees (amygdalis communis) planted in a loamy sand soil with a fine polyhedral structure. The control area belongs to the region of Jebeniana, 30 km from the factory. Similarities between polluted and non-polluted sites were verified by particle size analysis and the Tunisian pedological map revised by Lehouerou and le Floch (1987) [8]. Leaf samples from each species were taken from several branches in different parts of the tree side exposed to the factory fume. Control samples, of the same age as polluted ones, were gathered by the same sampling techniques. Only leaves occupying the middle of the shoots were taken. Three samplings of 100 leaves per month were gathered regularly from 10 plants/species (10 leaves/plant). In both sites, sampling was carried out from April to October. Parallel to the sampling, weekly field observations conducted regularly in the area allowed an accurate record of the necroses appearance time on both leaf species.
To determine the distribution of fluoride and nutrient elements in the leaf area, we selected, before fruit ripening, almond and pomegranate leaves exhibiting leaf tips and/or marginal necroses. 100 leaves (10 leaves/plant) were cut into marginal and central round slices including main veins. Marginal and central slices were separated from each other and then analyzed 24 hours after collecting. The same cutting model was applied to determine the concentration of different elements in the control site.
The follow-up of marginal and apical leaf necroses evolution was performed, along the growing season, on both leaf species. After estimating the percentage of leaf necroses, as described by Mabrouk and Carbonneau (1996) [9], damaged leaf areas were cut off carefully in such a way that healthy leaf surfaces remained attached on the mother plant shoots for photosynthesis measurements. Necrotic leaf slices were distributed into classes according to their necroses percentage. The same cutting model was applied for controls.
2.3 Analyses and measure methods
Leaf photosynthesis was measured using a portable infrared gas analyzer (CID 301 PS, USA) on attached almond leaves in the field, occupying the middle of shoots, between 9: 30 and 10 am. Only the healthy leaf area was introduced in the leaf chamber. Leaf surface as well as leaf percentage necroses were estimated according to Mabrouk and Carbonneau (1996) [9]. In order to avoid the effect of light intensity variation, all measurements were taken on sunny days, with Photosynthetic Active Radiation being higher than 1600 μmol m−2 s−1, by orienting the leaf chamber to obtain maximum light absorption. The average leaf temperature was . After photosynthesis measurements, attached healthy leaf areas were picked to determine their chlorophyll content. This latter was estimated by absorbance at 645 and 663 nm of an 80% acetone extract [10] of ten leaf discs obtained from each sample used for photosynthesis measurements.
Different leaf tissues for fluoride and nutrient elements analysis were dried at 80 °C for 48 h and ground to pass a 40-mesh sieve in a Willey hammer mill. Leaf powder was re-dried prior to weighing sub-samples for analysis at either 80 °C (fluoride) or 105 °C (nutrient elements) for 1–2 h.
Fluoride concentrations were determined using the potentiometric technique described by the Association of Official Analytical Chemists [11]. After digesting plant powder with nitric and perchloric acids (2v/1v), calcium and magnesium were analyzed by using the atomic absorption spectrophotometry technique. Phosphorus was determined by the vanado–molybdate phosphoric yellow colour method.
2.4 Statistical analyses
Statistical analyses were performed with the SAS package (Statistical Analysis System, version 6.12, Cary, NC, USA) using Student and Duncan multiple range Tests at the 5% significance level.
3 Results
3.1 Morphological responses of fruit species
Regular field follow-up in the polluted area allowed us to recognize various expressions of damage caused to almond and pomegranate trees. In almond, damage appears on leaf tips and margins from the beginning of the growing season. They consist of apical brick-yellow necroses that extend to leaf margins (Fig. 1). On pomegranate leaves, despite their high fluoride content, necroses were not frequent and appeared later at the end of August. They did not exceed 40% of the leaf surface.
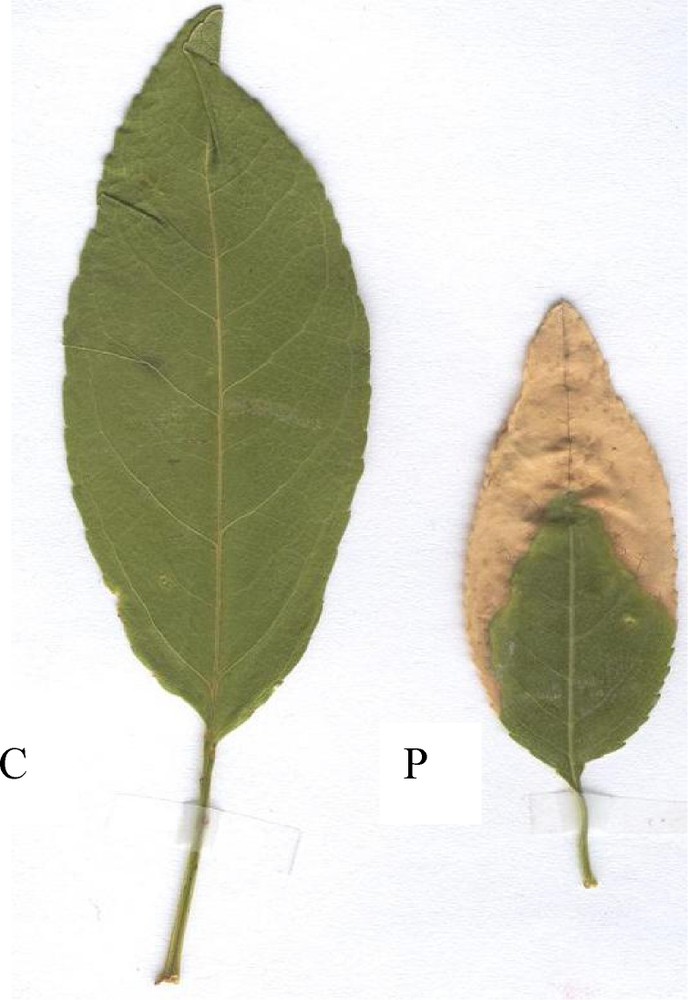
Control [C] and damaged [P] almond leaves.
3.2 Leaf calcium and fluoride distribution, during the growing season, in polluted and non-polluted area
In the non-polluted area, during the growing season, pomegranate and almond trees showed a slight increase in Ca leaf contents. However, in the polluted area, these species showed respectively an important increase in their calcium leaf content increasing jointly with fluoride, until the end of June and August, after which fluoride leaf contents dropped with a slight fall in calcium contents (Figs. 2 and 3). On the other hand, after weekly field observations of necrotic leaf tips and/or margins zones, we noticed that theses abnormalities occurred with the first appearance of necroses. Occurrence times are indicated on the curves (Figs. 2 and 3). Since fluorine is an extraneous element to plant metabolism, its fluctuations (from 14 to 16 μg/g of dry weight), in the non-polluted area were not significant and, hence, not represented on the figures.
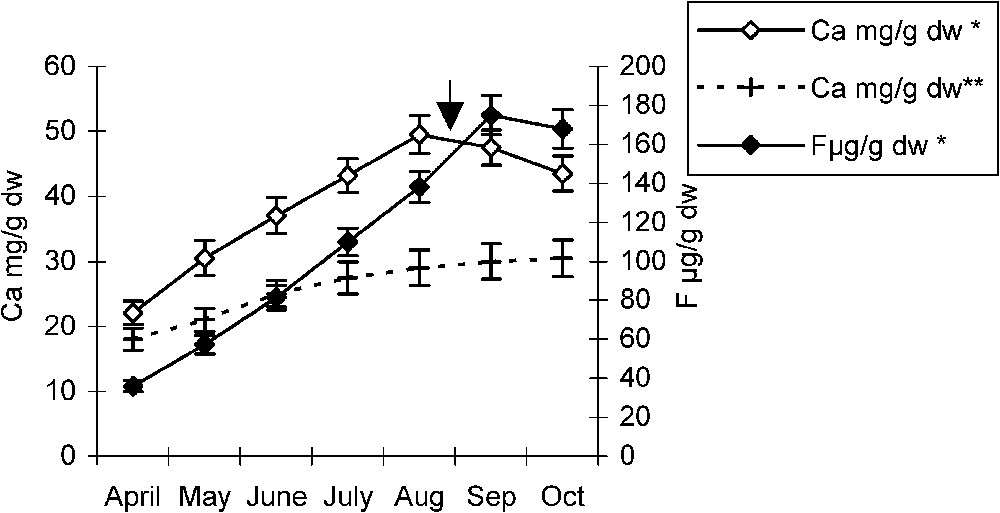
Temporal variations of calcium and fluoride in pomegranate leaf in polluted* and non-polluted** areas. The arrow indicates necroses appearance time. Error bars represent ± one standard deviation from the mean, n=3.
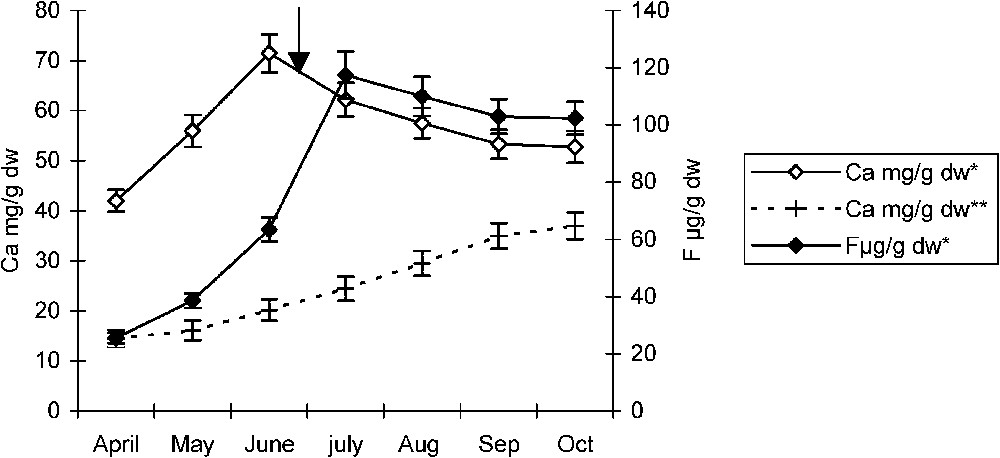
Temporal variations of calcium and fluoride in almond leaf in polluted* and non-polluted** areas. The arrow indicates necroses appearance time. Error bars represent ± one standard deviation from the mean, n=3.
3.3 Leaf magnesium and phosphorus distribution, during the growing season, in polluted and non-polluted area
In the non-polluted area, both pomegranate and almond trees showed a slight increase in their Mg leaf contents. However, a slight decrease in their phosphorus leaf contents was observed. A high-level accumulation of these two elements was recorded in the polluted area. Following their leaf calcium fall, pomegranates as well as almond trees show, respectively, in July and September a sudden increase in their leaf Mg contents (Figs. 4 and 5). After that, Mg content in almond leaves increases jointly with that of fluoride until the end of August, after which it drops, while necroses have invaded large leaf plates. However, that of pomegranate leaves tends to be maintained at a constant level. For both fruit species, and from the moment of necroses appearance, leaf phosphorus content did not cease increasing with time (Figs. 4 and 5).
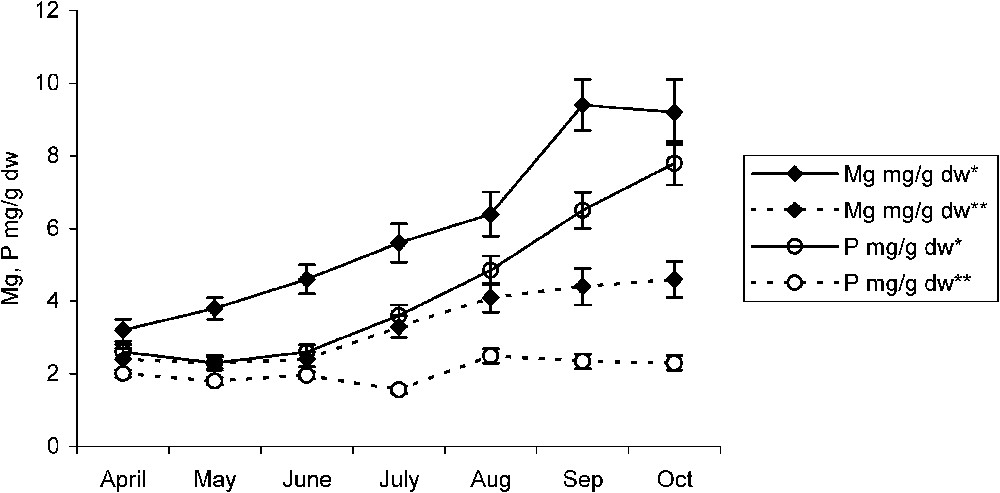
Temporal variations of magnesium and phosphorus in pomegranate leaf in the polluted* and non-polluted** areas. Error bars represent ± one standard deviation from the mean, n=3.
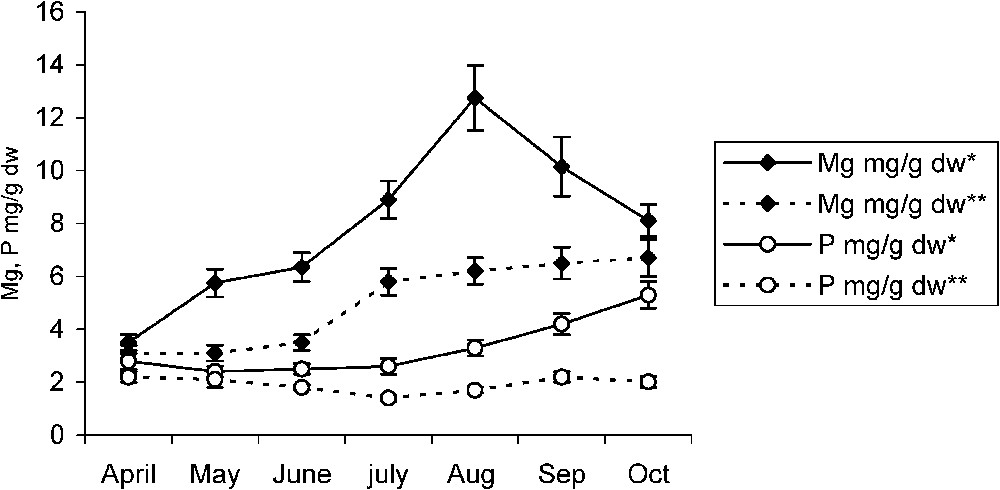
Temporal variations of magnesium and phosphorus in almond leaf in the polluted* and non-polluted** areas. Error bars represent ± one standard deviation from the mean, n=3.
3.4 Calcium, magnesium, phosphorus and fluoride distribution in leaf area
As shown in Figs. 6 and 7, fluoride concentrations recorded in leaf central parts including main veins are in the range of control ones. However, marginal leaf slices show a high-level accumulation of fluoride suggesting, in other words, that necroses appearing in these areas probably represent fluoride-induced symptoms. On the other hand, the mineral analysis of the central and marginal leaf (tips and/or margins) slices shows, for both pomegranate and almond, high calcium concentrations in leaf margins (Figs. 6 and 7). The pomegranate tree, supposed to be a high accumulator of fluoride in its leaf tips and/or margins, accumulates jointly more calcium contents than almond (Figs. 6 and 7). For both species, Mg concentration is reduced in leaf margins with respect to that of the centre. However, with respect to almond species, pomegranate seems to keep more magnesium in its leaf central parts (Figs. 6 and 7). High phosphorus concentrations in the leaf centre as well as in the leaf tips and/or margins of the pomegranate tree are recorded in polluted areas (Fig. 6). Phosphorus content in almond leaf margins is in the range of the control ones (Fig. 7).
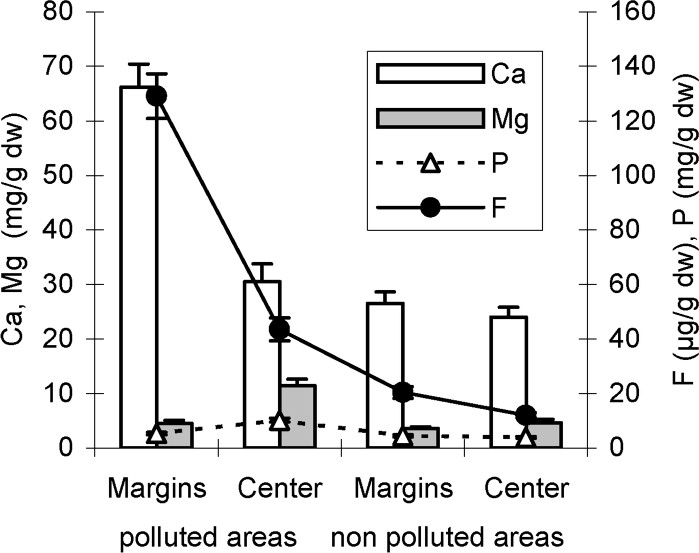
Fluoride, calcium, magnesium and phosphorus concentrations of central and (tips + marginal) leaf slices of pomegranate tree in polluted and non-polluted areas. Values are the means ± SD of 10 replicates.
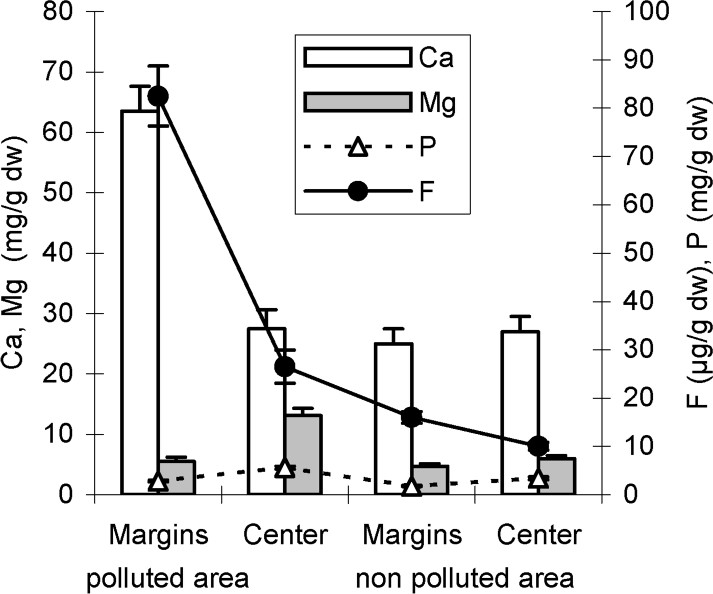
Fluoride, calcium, magnesium and phosphorus concentrations of central and (tips + marginal) leaf slices of almond tree in polluted and non-polluted areas. Values are the means ± SD of 10 replicates.
3.5 Photosynthesis and chlorophyll contents
We can see, for both species, that while the fluoride contents of damaged leaf areas increase with the percentage of leaf necroses, the photosynthesis of healthy leaf areas decreases (Tables 1 and 2). With 30 to 40% of leaf necroses, the photosynthesis and chlorophyll contents of almond leaves decreased more significantly than those of pomegranate, which proceeds in the range of control (Tables 1 and 2). However, up to 60% of almond leaf necroses, chlorophyll content decreases by 48% with respect to that found in leaves of non-polluted almond trees (Table 1).
Net photosynthesis (μmolCO2 m−2 s−1) and chlorophyll content (mg dm−2) of healthy leaf almond areas in function of leaf necroses percentage
Percentage of leaf necroses % | |||||
Control | 10–20 | 30–40 | 50–60 | >60 | |
Classes | C0 | C1 | C2 | C3 | C4 |
Pn | 18.1 ± 1.1 | 16.9 ± 1.2NS | 15.16 ± 1.34+++ | 12.24 ± 1.17+++ | 8.0 ± 1.0+++ |
Chl content | 2.81 ± 0.15 | 2.74 ± 0.12NS | 2.17 ± 0.08+++ | 1.89 ± 0.07+++ | 1.46 ± 0.04+++ |
Net photosynthesis (μmolCO2 m−2 s−1) and chlorophyll content (mg dm−2) of healthy leaf pomegranate areas in function of leaf necroses percentage
Percentage of leaf necroses | |||
Control | 10–20 | 30–40 | |
Classes | C0 | C1 | C2 |
Pn (n=19) | 14.5 ± 1.1 | 13.8 ± 1.2NS | 13.2 ± 1.4NS |
Chl content | 3.01 ± 0.15 | 2.94 ± 0.12NS | 2.83 ± 0.08+ |
4 Discussion and conclusion
4.1 Morphological responses of fruit species
As shown in Fig. 1, necrotic tissues are separated from healthy ones by a dark violet borderline. It consists of anthocyanin pigments secreted by plant leaf cells when subjected to any stress [12]. The secretion of anthocyanins along a waved line seems to show a primary defence reaction developed by the plant in order to limit damage at leaf tips and/or margins. The little fluoride retained by the almond leaf central parts, including the main veins, on the one hand, and the fluoride preferential accumulation in almond leaf tips and/or margins, where necroses appear, on the other hand, suggest that apical and marginal necroses probably represent typical symptoms of fluoride pollution as previously described [7,13]. These findings are confirmed by the non-significant fluoride quantities recorded in the internodes and leaf stalks adjacent to pollutant leaves (unpublished results of our laboratory). Miller (1993) and Fornasiero (2001) [14,15] reported that gaseous fluorides enter the leaf by diffusion through the stomata, and dissolve in the humid spaces of sub stomatal cavity; the ions are carried with the transpiration stream to sites of greatest evaporation, which are usually leaf margins and tips where concentrated F− amounts cause the first signs of damage.
As for the pomegranate tree, our results are in agreement with our previous works considering this species as resistant to air fluoride pollution, since resistant fruit species are those accumulating fluoride without showing symptoms either of F toxicity or growth restrictions [7].
4.2 Fluoride–calcium interaction
In comparison with the same species growing in the control areas, the temporal evolution of their Ca and Mg leaf contents seems to be influenced by fluoride accumulation. In both species, during fluoride intoxication, there is a tendency to balance the slowness of fluoride accumulation by a parallel slowness of calcium accumulation (Figs. 2 and 3). In addition, the coincidence of calcium content fall with the appearance time of the first leaf necroses suggests a strong affinity between these two elements as well as a relation between necroses appearance and the quantity of calcium available in leaf tips and/or margins. Therefore, damage seems to appear in the form of necroses when calcium ions are no more available, probably corresponding to its fall (Figs. 2 and 3).
These findings are corroborated by the high calcium and fluoride contents recorded in pomegranate and almond leaf tips and margins slices (Figs. 6 and 7). The strong affinity between fluoride and calcium has been reported with other cations such as silicon [16]. Likewise, Konishi and Miyamoto (1983) [17] have mentioned the possibility of attenuating F− toxicity on tea plants by an excess of aluminium. In our study, pomegranate accumulating high leaf fluoride and calcium in its tips and/or margins (Fig. 6) did not exhibit any typical symptoms of fluoride intoxication until the end of August. This is not the case for the almond tree, where necroses appear from the end of June (Fig. 3). According to Machoy-Mokrzynska (1995) [18], it is a strategy that consists in a trapping mechanism of fluoride in the form of CaF2 and hence cannot disturb the plant metabolism.
Therefore, the pomegranate tree, which could assimilate calcium ions, would have the ability not only to fight F− ions toxicity through trapping them in the form of a CaF2 insoluble complex, but also to delay the appearance of necroses as late as possible, thus explaining the relative thriving and fructifying of pomegranate despite its existence in the most polluted area. Considering these data, it would be more rational to grow plants with high calcium content in polluted areas. However, by tending to considerably increase their Ca and Mg content against F− toxicity, polluted species may pay a physiological cost that consists in accelerating their leaf aging and reducing their yield production. As a case in point, species growing in polluted areas are generally characterised by reduced leaf surfaces (Fig. 1) and number.
On the other hand, the maximum fluoride contents reached respectively in July and September by pomegranate and almond trees (Figs. 2 and 3), at the moment when necroses have already invaded leaf tips and margins, seem to ascertain the existence of a threshold concentration above which fluoride becomes toxic.
4.3 Fluoride–magnesium interaction
In comparison with non-polluted species in pomegranate and almond, the sudden increase of Mg leaf content (Figs. 4 and 5) occurring just after a fall in its calcium leaf content suggests the existing possibility of a second MgF affinity. It appears that this phenomenon, as it is for CaF, consists of a fluoride trapping mechanism in the form of MgF2, which helps sensitive species such as the almond tree [5,7] to avoid damage as long as possible. The decrease in almond magnesium leaf concentration since September (Fig. 5) suggests, however, that this type of Mg provided protection is temporary. In fact, taking into account the multiple roles of Mg as a regulator of osmotic relations, and an activating agent of various important physiological processes as phosphorylation, citric acid cycle, respiration chain, absorption of other nutrient elements, enzyme reactions, proteins synthesis as well as photosynthesis where Mg is a central component of chlorophyll molecule [19,20], this interaction may constitute a limiting factor for the metabolic functions. This is true for sensitive plants as well as for the calcium and/or magnesium deficient plants. In accordance with our results, Machoy-Mokrzynska (1995) [18] reported that MgF interaction lowers all enzyme activities where Mg is involved. In contrast to almond, pomegranate Mg leaf content increased jointly with that of fluoride until the end of September after which Mg content tends not to drop (Fig. 4). Hence, species resistant to fluoride pollution, as pomegranate, have the ability to preserve their Mg leaf concentration at an adequate level compatible with its survival in arid and polluted areas. This hypothesis is confirmed by present results, which reveal a high Mg concentration in leaf central parts with respect to leaf tips or margins (Figs. 6 and 7). In addition, with 30 to 40% of leaf necroses, almond photosynthesis decreased more significantly than that of pomegranate, which proceeds in the range of control (Tables 1 and 2), thus suggesting its relative tolerance to air pollution. We also suggest that tolerant varieties accumulating fluoride in leaves are able to sequester it in the form of CaF2 or MgF2, in order to protect metabolic activities from its harmful effects. Necroses and burns appearing in leaf blade extremities are induced by a double interaction of calcium and magnesium with fluoride [16].
According to Charlot and Kisman (1983) [16], the exclusion of fluoride from protoplasm and cellular juice can only be as insoluble CaF2. Accordingly, when no more calcium is available, Mg will be taken from the chlorophyll molecule by reaction with fluoride, thus explaining the decrease in chlorophyll concentration and in net photosynthesis as almond leaf necroses percentage increases (Table 1). Hence, at more than 60% of almond leaf necroses, chlorophyll content decreases by 48% compared to that found in leaves of almond trees growing in non-polluted agro-systems. This reduction in chlorophyll becomes significant when the percentage of leaf necrosis varies between 30 and 40% (Table 1). This may be related to the breakdown of the membrane structure within the chloroplasts or to direct fluoride effects on chlorophyll biosynthesis, which in both cases leads to a loss of photosynthetic capacity and appearance of visible symptoms of necroses or chloroses. Our results are consistent with those of Stevens et al. [21], who considered the sequestration of Ca with F as a selective mechanism that modifies the permeability of cell membrane to fluoride ions. These modifications will attenuate, in the long run, the barrier preventing the entry of fluoride ions in cell compartments. Working on cell tissue culture, Diesendorf and Sutton [22] reported that, in the presence of fluoride, DNA molecule may be damaged and genetic malformations can be induced. After a long-term exposure to HF fumigations, dissolved fluoride in the mesophyll cell wall might diffuse across the membrane lining the cell and enter the cytoplasm, where it may become associated with cell organelles such as chloroplasts and mitochondria [23,24]. In consistence with our results, Giannini et al. [25] mentioned that the harmful effects of F− might cause physiological alterations and even cell death depending on its concentration in the cell sap.
4.4 Phosphorus–fluoride interaction
Throughout the growing season, from April to July, the phosphorus leaf content of the studied fruit species seems to be independent of pollutants effects. However, as pollutant toxicity increases and when necroses occupy more than 30% of almond leaf surfaces, phosphorus content keeps on increasing (Fig. 5). This occurs while almond magnesium concentration tends to be reduced. This is not the case of pomegranate where phosphorus concentration tends to increase from the beginning of the growing season (Fig. 4). According to Weinstein and Alscher-Herma [26], the toxic action of F consists in inactivating Mg at its sites of physiological activities, thus leading to the inhibition of the enzyme systems in which Mg is involved. However, this blocking occurs only when fluoride concentration is sufficient in the cell [23]. Therefore, the subsequent increase in phosphorus concentration seems to be a tendency of the plant to provide a supply of this element in order to protect enzyme systems in which Mg and/or P are involved. Doris (1985) [27] reported that fluorides inhibit a great number of enzymes such as phosphatases, phosphorylases, phospho-glucomutases. Therefore, a type of fluoride–Mg–phosphate complex is thought to be the cause of Mg inactivation. Since phosphorus is the main component of nucleic acids and phospholipids, and given its important part in the metabolism as PO2−4 group in phosphorylated compounds [19,20], the progressive accumulation of phosphorus and magnesium recorded for pomegranate throughout the growing season (Fig. 4) seems to have a beneficial effect allowing this resistant species to flower and to fructify in the most polluted area. These findings are consistent with those of Edwin and George (1993) [28], who reported that Mg is highly required for the activity of enzymes involved in the transfer of phosphate. In addition, the joint increase of phosphorus and magnesium in leaf central parts (Figs. 6 and 7) and the late appearance of necroses on pomegranate leaves suggest that a calcium–Mg–phosphate complex probably lies behind fluoride inactivation. Similarly, in grapevine, Champagnol [29] reported a correlation between the injury symptoms of saline toxicity and low phosphorus supply. In these conditions, dust and particles in the form of crushed crude phosphate drifted daily by wind in the area [3] may act as an adaptation factor to fluoride pollution. Besides, the increasing phosphorus content of the almond tree occurring after its magnesium content decrease seems to be a strategy allowing sensitive species to fight F− toxicity as much as possible and to survive in such restrictive conditions.
Acknowledgements
Support and technical assistance of Olive Institute and Regional Laboratory of Environmental Sciences (LARSEN) are greatly appreciated by authors.