1 Introduction
Phosphoenolpyruvate carboxylase (PEPc: EC 4.1.1.31) catalyses the irreversible β-carboxylation of phosphoenolpyruvate in the presence of to yield oxaloacetate and inorganic phosphate Pi [1,2]. PEPc catalyses the first step in the fixation of atmospheric CO2 during C4 photosynthesis [3]. This enzyme has been extensively studied in C4 and crassulaceae (CAM) plants. C3 plants contain less, yet appreciable, amounts of PEPc, which is found in virtually all organs and may play multiple physiological roles [4]. The properties and functions of PEPc in C3 and non-photosynthetic tissues of C4 and CAM plant are less well understood [1]. One role is the so-called anaplerotic function, which consists in the replacement of oxaloacetate in the tricarboxylic acid cycle, whenever the demand for carbon skeletons for amino acid biosynthesis is high. There is also increasing evidence for the presence of PEPc in seeds of different plant species [2]. Smith et al. [5] demonstrated that malate supports very high rates of fatty acid synthesis by isolated leucoplasts prepared from developing Castor oilseeds. Based on the measurement of localized NADP+ malic enzyme, they proposed that malate might be a key carbon source for fatty acid synthesis in vivo. PEPc may convert PEP into malate, which is then utilized by the leucoplast for fatty acid biosynthesis [1]. Developing Brassica napus seeds and microspore-derived embryos contain relatively high PEPc activity during their most active phase of oil synthesis [1]. In oleaginous plants, the PEPc is active, in particular during development of seeds [6]. Its activity and concentration increase have been observed during the most active phase of lipid accumulation during the course of castor seeds maturation [7]. In spite of the high importance of this enzyme in lipid and fatty acids synthesis, PEPc was less studied in the oleaginous plants [8].
Rapeseed is an important potential source of edible unsaturated oils. It is one of the most oleaginous plants cultivated in the world and it offers several advantages in the application of biotechnical approaches to improve oil composition [9,10]. Sebei et al. [11] reported that two Tunisian rapeseed varieties (Hybridol and Pactol) contained more than 60% of oleic acid, 18% of linoleic acid, and 8% of linolenic acid. Fatty acid composition varied from variety to variety and changed according to environmental conditions [12,13]. Only few information dealing with lipid accumulation in seeds, all along the course of maturation in relation with PEPc activity are available, and none concerns rapeseed.
The present investigation focuses on the time–course changes in PEPc activity during maturation of seeds from two varieties (Hybridol and Pactol) of rapeseed (Brassica napus L.), and (ii) the accumulation of total lipids, fatty acids and triacylglycerols. The two rapeseed cultivars used here are widely cultivated in Tunisia, owing to their high seed oil yield.
2 Material and methods
2.1 Culture conditions and plant sampling
The study was carried out on the two rapeseed varieties, Hybridol and Pactol (‘double zero’). The plants were grown in a parcel at the National Institute of Agronomic Research of Tunisia (INRAT), under natural conditions and were irrigated with tap water twice a week until flowering stage. During this study, seven samples (one sample per week for each variety) were achieved, starting with the apparition of siliques (30 days following flowering) until the end of seed maturation. It is noteworthy that sampling of Pactol seeds occurred earlier than that of Hybridol seeds because of its precocity.
2.2 Total lipid extraction
Total lipid extraction was performed according to Folch [14]. This extraction was used to determine fatty acid and triacylglycerol composition. The seed oil content was determined using the Soxhlet extraction according to the official method [15]. From each cultivar, 50 g were ground and than extracted with petroleum ether in a Soxhlet apparatus for 6 h. Petroleum ether was then evaporated under reduced pressure using a rotavapor. Oil content was expressed as % of seed fresh weight.
2.3 PEPc activity
The extraction was achieved by grinding frozen seeds (250 mg) in a chilled mortar. Powder was resuspended in 1 ml of an extraction medium containing in 100 mM Tris-HCl pH 8: glycerol (5%), 3.5 mM MgCl2, mercaptoethanol (1%). The protease inhibitors were: 1 mM PMSF and Polyclar 10%, 100 μl of mercaptoethanol and polyvenylphosphate (PVP). The samples were centrifuged (13 000 rpm at 4 °C for 15 min). PEPc activity was assayed at 340 nm by coupling the reaction to NADH-oxidation mediated by malate dehydrogenase (MDH). The standard assay medium contained in 100 mM Tris-HCl (pH 8) : 5 mM MgCl2; 1.6 mM NaF; 0.2 mM NADH; 1.9 mM DTT; 10 mM NaCO3 and 4 mM phosphoenolpyruvate (PEP). The reaction was initiated by addition of 700 μl standard assay medium, 10 μl DTT and a buffer volume. After 30 min at 30 °C, 20 μl NADH, 100 μl PEP and the sample were added.
2.4 Analysis of total fatty acids
Fatty acids were methylated using the technique of Metcalfe et al. [16] modified by Lechevallier [17]. Methyl esters were analysed by gas chromatography (GC) using a HP 4890 chromatograph equipped with a flame ionisation detector (FID) on a capillary column coated with supelcowaxTM 10 (30 m length, 0.25 id, 0.2 mm film thickness). Temperatures (°C) of column, detector and injector were: 200°, 250° and 230°, respectively.
2.5 Analysis of triacylglycerols (TAG)
TAGs were analysed by High-Performance Liquid Chromatography (HPLC) on a SCHIMADZU apparatus equipped with a RP18 stainless steel column (250-mm length × 4.6-mm internal diameter), a guard column and a refractive index detector (differential refractometer). The mobile phase was acetonitrile/acetone 1:1 v/v. The flow rate was isocratically controlled at 1.5 ml min−1. Ten microlitres of sample were injected at each run.
2.6 Statistical analysis
The data (three replicates) were statistically evaluated using the SAS version 12.6 software (Statistical Analysis System). (SAS, Institute INC, Box 8000, Cary, North Carolina 27511, USA).
3 Results
3.1 Developmental profiles of PEPc activity (Fig. 1)
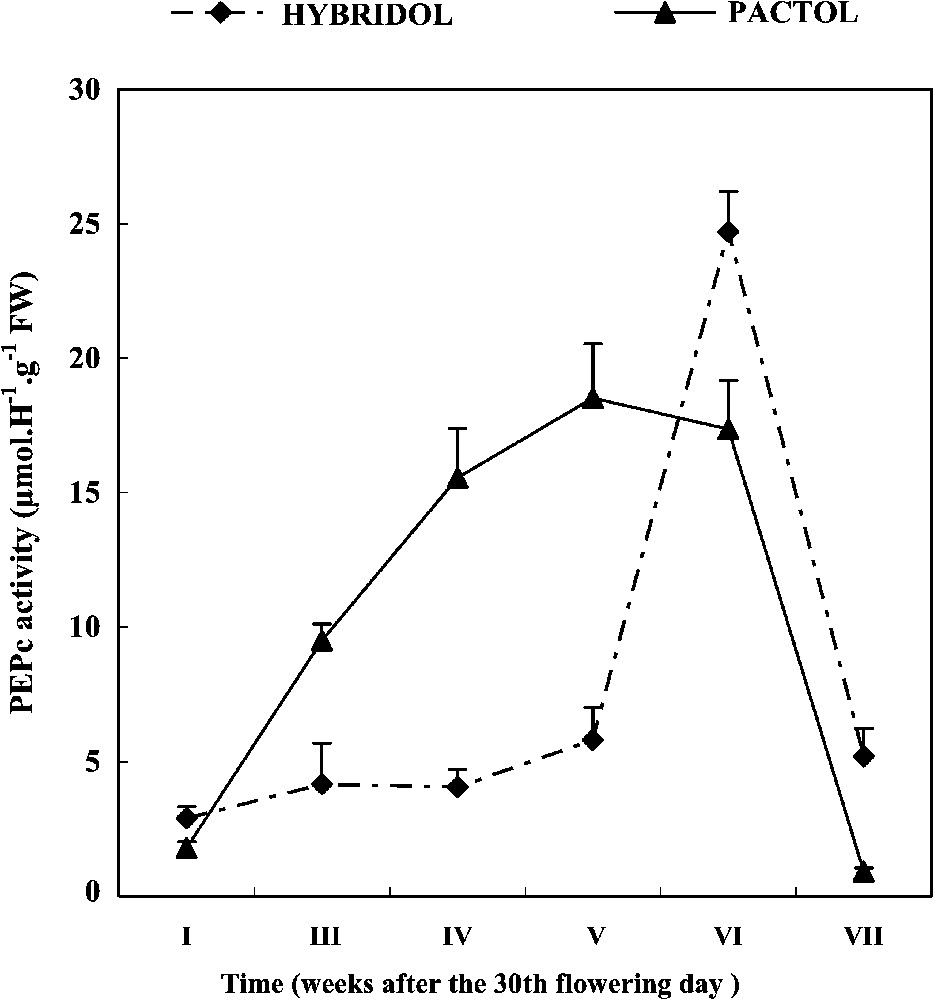
Developmental profiles of PEPc activity during seed maturation of two rapeseed varieties (mean values, n=3).
In both varieties, PEPc activity dropped sharply immediately after seed maturation. During the later seed maturation weeks, a marked difference was observed between the two varieties. For Hybridol, PEPc activity was very low during the first weeks of maturation, followed by a spectacular increase, so that the maximum of PEPc activity (25 μmol H−1 g−1 FW) corresponded to the sixth week of maturation (one week before the end of Hybridol maturation). On the contrary, for Pactol, time-course changes in PEPc activity were more pronounced, varying according to a hyperbole like curve. The maximum of PEPc activity (18 μmol H−1 g−1 FW) was recorded at the fifth week of seed maturation.
3.2 Evolution of oil content during maturation (Fig. 2)

Evolution of oil content during maturation of two rapeseed varieties (mean values, n=3).
In both varieties, seed oil content was low during the first period of lipid accumulation (2 weeks) (18 and 25 g 100 g−1 DM, respectively for Pactol and Hybridol). The second period (three weeks for Pactol and four weeks for Hybridol) was characterised by a drastic increase in seed-oil content, reaching a maximum value at five (47%) and six weeks (45%), respectively for Pactol and Hybridol. Later maturation stages showed a significant decrease in lipid accumulation, especially in Pactol.
3.3 Evolution of seed fatty acid (FA) content during seed maturation (Fig. 3)

Evolution of fatty acid (FA) content during seed maturation of two rapeseed varieties (mean values, n=3).
Pactol and Hybridol varieties displayed different time-related changes in this parameter. In both varieties, the main fatty acid was oleic acid (C18:1) (respectively). The content of the latter increased during seed maturation, but appeared to be genotype-dependent: in Pactol, C18:1 percentage rapidly increased approximately in a linear way, up to the fifth week (ca. 650 mg g−1 oil), before slowly decreasing. On the contrary, the oleic acid (C18:1) content in Hybridol increased slowly during the first four weeks of maturation. It then sharply increased during the following two weeks, and attained a maximum value at the sixth week (ca. 700 mg g−1 oil), being higher than the maximum observed in Pactol.
Concerning the evolution of the polyunsaturated fatty acid (PUFA) content, it increased in Pactol during the first three weeks of seed maturation and then regularly decreased. In contrast, in Hybridol, it increased only slightly from the second week to the fourth week, with a maximum at the third week, as found in Pactol, but at a lower amount (ca. 230 mg g−1 oil and 250 mg g−1, respectively). The saturated fatty acid (SFA) remained low during the whole seed maturation period.
3.4 Evolution of the percentage of linoleic acid (C18:2) and linolenic acid (C18:3) and the three main triacylglycerols (TAG) (Figs. 4 and 5)
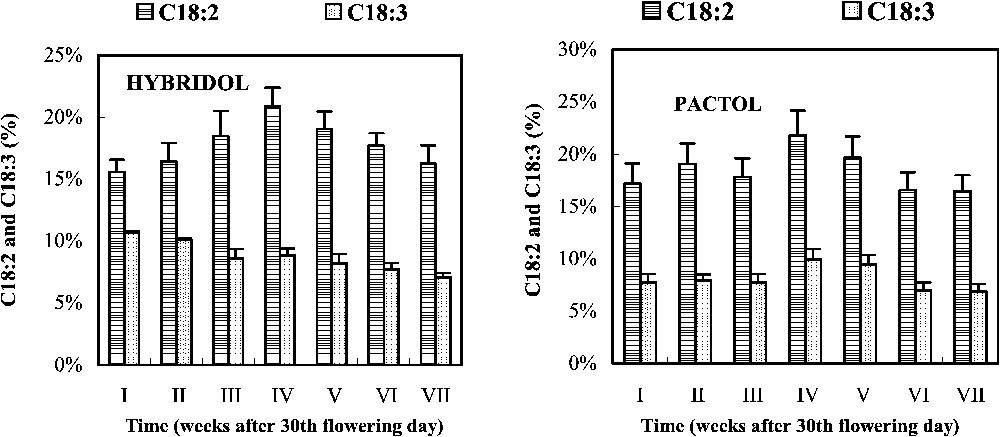
Evolution of the percentage of linoleic acid (18:2) and linolenic acid (18:3) during seed maturation of two rapeseed varieties (mean values, n=3).
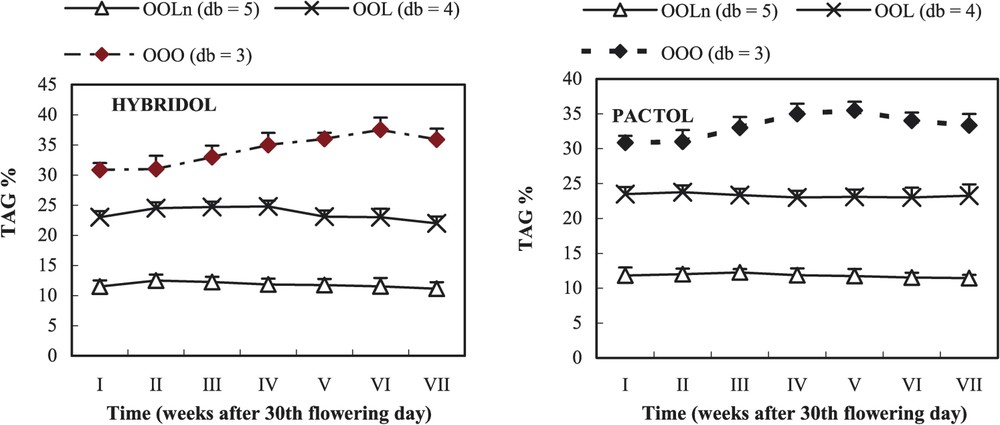
Evolution of the percentage of the three main triacylglycerols (TAG) during maturation of two rapeseed varieties (mean values, n=3). db = double bond.
In Hybridol, the C18:2 percentage slightly increased from ca. 15% of the total FA to ca. 20% at the fourth week, before decreasing to reach the initial value (16%) at the end of maturation (Fig. 4). The C18:3 percentage, however, decreased regularly from the beginning to the end of seed maturation (12 and 8%, respectively). In Pactol, no significant changes in C18:2 and C18:3 percentages were observed during seed maturation, despite a slight maximum was shown at the fourth week. C18:3 percentage which was low at the beginning, remained rather constant (Fig. 4).
In Hybridol and Pactol varieties, the three main TAG were in decreasing order: trioleoglycerol (OOO), dioleolinoleoglycerol (OOL) and trioleoyllinolenoylglycerol (OOLn) (Fig. 5). In both varieties, the percentage of OOO increased from the beginning of maturation to reach a slight maximum at the fifth and sixth week, respectively in Pactol (35%) and Hybridol (36%), before slightly decreasing (32 and 33%, respectively).
4 Discussion
Our findings revealed a close relationship between PEPc activity and accumulation of oleic acid during maturation of seeds in both varieties. The higher content in oleic acid corresponded to the higher activity of PEPc, and the high activity of PEPc resulted in high synthesis of saturated acids, especially stearic acid, in Pactol. Oleic acid (18:1) is synthesized from stearic acid (18:0) by Δ9 desaturation, a reaction that occurs in plastids. Since the SFA content remained low in this variety, it can be thought that the activity of the Δ9 desaturase was very high. In Hybridol, the synthesis of stearic acid increased only slightly as did PEPc activity. The latter was very high up to the sixth week of maturation, like the oleic acid content. In addition, the fact that SFA also remained low during seed maturation may indicate that the activity of the Δ9 desaturase was high in this variety.
The conversion of oleic acid (C18:1) into linoleic acid (C18:2) in Pactol by Δ12 desaturation may occur at the same rate as Δ5 desaturation in Pactol. In addition, the Δ5 desaturation may convert C18:2 into C18:3, since the ratio of these fatty acids did not vary during maturation. In Hybridol, the activity of Δ5 desaturase seemed to decrease with seed maturation, resulting in a lower proportion of linolenic acid (C18:3) as compared to linoleic acid (C18:2). The desaturation of C18:1 occurs while esterified to phosphatidylcholine (PC). Two specialised membrane-associated desaturases, FAD2 and FAD3 are involved in the synthesis of C18:2 and C18:3 respectively [18]. The acyl chains of phosphatidylcholine are esterified to coenzyme A, and transferred to cytoplasm where it constitutes a substrate pool for TAG formation. In our experiments, in agreement with the Kennedy scheme [19], the synthesis of the three main TAG fractions (OOO, OOL and OOLn) occurred from dioleoglycerol. The respective amount of these three TAGs decreased according to the order of formation of the three different FA (O, L, and Ln).
Results reported above suggest a close relationship between PEPc activity and FA biosynthesis activity in the rapeseeds studied. This suggestion is supported by a close parallelism between the enzyme activities at the different stages of maturation of the two rapeseed varieties. This agrees with previous data, reporting for instance that developing Brassica napus seeds and microspore-derived embryos show relatively high PEPc activity during their most active phase of oil synthesis [1]. Other authors also showed that, in oleaginous plants, PEPc activity was particularly high during development of seeds [7] and that PEPc concentration and activity were increased during seed maturation [8]. A schema is proposed (Fig. 6), in which it appears that PEPc, in concert with cytosolic malate dehydrogenase, may convert phosphoenolpyruvate (PEP) derived from imported sucrose via glycolysis into malate. The latter is then used by the leucoplast for fatty-acid biosynthesis. The glycolytic pathway of developing oil seed leucoplasts probably plays an important role in generating carbon skeletons and the energy required for long-chain fatty synthesis [1]. The synthesised stearic acid as 18:0-ACP under-goes desaturation at C9, catalysed by the Δ9-desaturase, to form oleoyl–ACP–Acyl–ACP thioesterases, which hydrolyse the acyl-ACPs to produce free FAs. These leave the plastid and upon arrival in the cytoplasm, become esterified in acyl-Coa, which serves as a substrate for endoplasmic reticulum-based TAG formation according to Kennedy pathway.

Carbon circulation and fatty acids biosynthesis via PEPc in oilseeds.
Acknowledgements
Authors are grateful to Emeritus Prof. Jean Bezard, ‘UPRES Lipides et Nutrition’, ‘Université de Bourgogne’, Dijon, France, for his valuable advices.