1 Introduction
The impact of introduced species on native species, indigenous communities, and ecosystems is diverse, threatening biodiversity on both the genetic and species levels [1]. Even though introduced species are often unable to establish persisting populations [2], around 15% find themselves unrestrained by predators, parasitoids, parasites, or interspecific competition [1]. Such populations might grow exponentially, and they are considered as substantial contributors to global biodiversity change [3]. Invasive species have been the focus of a large body of studies, but the complexity of the invasion process restricts the potential of predicting the future ecological success of introduced species with any degree of accuracy. In particular, variability of local adaptation is responsible for difficulties in predicting the duration of the time lag between initial introduction and the beginning of increased population growth and subsequent range expansion [1]. Basic ecological research, therefore, has a large potential for contributing significantly to the management of invasive, or potentially invasive species. Management actions need profound knowledge of the biological process that accompanies introductions of species. For example, Newsome and Noble [4] were able to link the invasiveness of a species to the higher longevity and growth rate of individuals, as that kind of species would survive, resist, and recover from temporarily suboptimal environmental conditions better than short-lived and slow-growing species.
The introduction of Rana ridibunda to France and Switzerland has led to a significant change of the indigenous water frog fauna [5]. Most of the stable R. ridibunda populations in France and Switzerland are the result of introductions due to human consumption [6–8]. The use of genetic methods to determine the range of water frogs in France has shown that R. ridibunda inhabits a much larger area in France than anticipated earlier [9,10]. Here, we contribute to the small number of studies illustrating the possible threats that R. ridibunda poses to the indigenous water frog assemblages of France, with a focus on R. perezi and its hybridogenetic associate R. grafi. These two frog taxa are always sympatric, due to dependence of the hybrid R. grafi on backcrosses with R. perezi, similar to their more northerly distributed relatives, R. lessonae and R. esculenta [11,12]. We test for habitat preferences of the different species and differences in the ratio of R. grafi to its parental species R. perezi in dependence on the presence or absence of R. ridibunda. In addition, we compare the longevity and growth rates of R. ridibunda, R. perezi, and R. grafi, which are important factors in interspecies competition to evaluate the competitive strength of the different frog taxa [13,14]. Additionally, we also contribute data to the distribution range of R. ridibunda, R. perezi and R. grafi in Mediterranean France. Such data are difficult to produce, as the classification of water frogs based on morphological data alone is extremely difficult, and needs confirmation by genetic methods [15].
2 Material and methods
We collected water frogs (
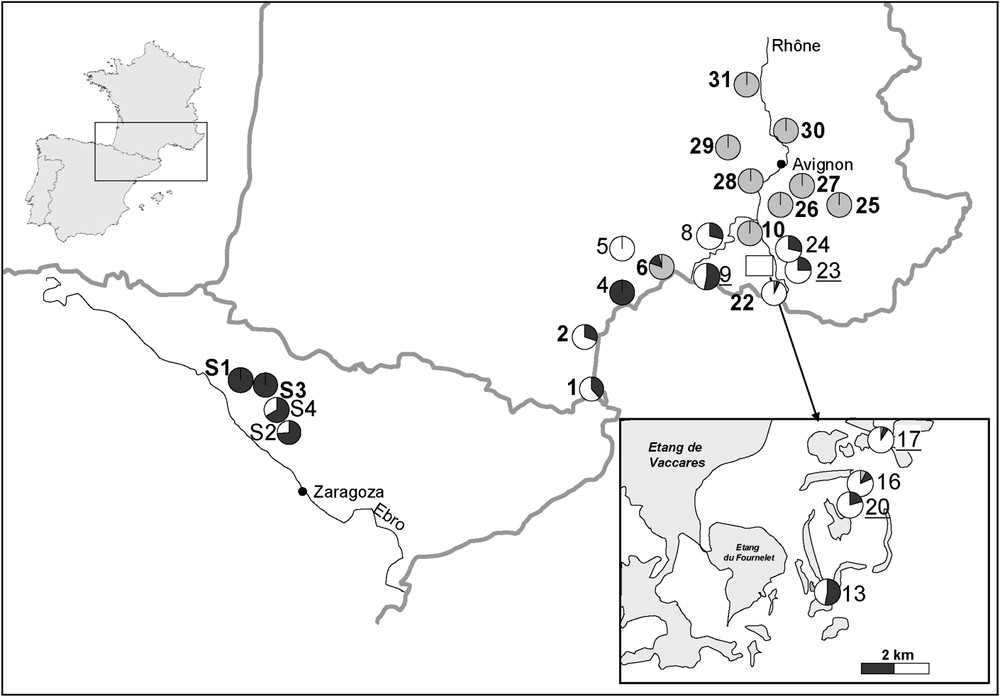
Population composition. The figure illustrates the relative amount of the three taxa R. ridibunda (grey), R. perezi (black), and the hybridogen R. grafi (white). The numbers correspond to Table 1. Bold population numbers indicate habitats of the A-type, plain ones represent B-habitats, and italic ones stand for C-habitats.
Environmental characteristics of the different populations
No. | N | R. ridibunda | R. perezi | R. grafi | Sex ratio | Ht | S/cm | pH |
|
Coordinates | Commune (French ‘département’) |
01* | 26 | 10 (38) | 16 (62) | 1 : 7 | A | 926 | 6.8 | 130 | 42°34.39 N, 3°00.48 E | Argelès-sur-Mer (66) | |
02* | 30 | 9 (30) | 21 (70) | A | 642 | 6.0 | 120 | 42°39.14 N, 2°54.39 E | Saint-Cyprien-Plage (66) | ||
04* | 37 | 19 (51) | 18 (49) | B | 1856 | 5.6 | 110 | 43°15.30 N, 3°10.90 E | Valras-Plage (11) | ||
05 | 17 | 17 (100) | B | 1200 | 6.8 | 120 | 43°48.34 N, 3°45.60 E | Saint-Martin-de-Londres (34) | |||
06* | 29 | 24 (83) | 4 (14) | 1 (3) | A | 1474 | 6.4 | 110 | 43°33.23 N, 3°54.21 E | Lattes (34) | |
08* | 28 | 8 (29) | 20 (71) | B | 1150 | 5.3 | 159 | 43°36.43 N, 4°20.34 E | Saint-Gilles (30) | ||
09 | 25 | 13 (52) | 12 (48) | 1 : 5 | C | 4690 | 6.0 | 70 | 43°32.80 N, 4°26.11 E | Les Saintes-Maries-de-la-Mer (30) | |
10* | 22 | 22 (100) | A | 870 | 5.8 | 65 | 43°38.86 N, 4°35.92 E | Arles (30) | |||
13* | 34 | 18 (53) | 16 (47) | 1 : 2 | B | 1050 | 5 | 200 | 43°28.71 N, 4°38.99 E | Le Sambuc (13) | |
16* | 32 | 1 (3) | 2 (6) | 29 (91) | 1 : 4 | B | 394 | 5.9 | 140 | 43°30.50 N, 4°40.18 E | Le Sambuc (13) |
17* | 27 | 2 (7) | 3 (11) | 22 (82) | C | 5200 | 5.4 | 90 | 43°31.30 N, 4°40.44 E | Le Sambuc (13) | |
20* | 24 | 5 (21) | 19 (79) | 1 : 5 | C | 450 | 5.3 | 30 | 43°30.32 N, 4°40.02 E | Le Sambuc (13) | |
22* | 27 | 1 (4) | 1 (4) | 25 (93) | A | 20000 | 5.7 | 130 | 43°22.23 N, 4°48.33 E | Salin-de-Giraud (13) | |
23 | 35 | 10 (29) | 25 (71) | C | 1500 | 5.3 | 130 | 43°32.20 N, 4°45.31 E | Mas Thibert (13) | ||
24* | 24 | 6 (25) | 18 (75) | 1 : 13 | B | 1020 | 6.3 | 100 | 43°27.99 N, 4°52.86 E | Mas Thibert (13) | |
25 | 28 | 28 (100) | A | 843 | 6.7 | 120 | 43°45.16 N, 5°13.45 E | Merindol (84) | |||
26 | 27 | 27 (100) | A | 630 | 5.3 | 180 | 43°47.15 N, 4°57.10 E | Mollégés (13) | |||
27 | 21 | 20 (95) | A | 689 | 6.2 | 65 | 43°50.01 N, 5°00.28 E | Cavaillon (84) | |||
28 | 28 | 28 (100) | A | 584 | 6.5 | 111 | 43°53.10 N, 4°41.32 E | Aramon (30) | |||
29 | 28 | 28 (100) | A | 860 | 6.4 | 100 | 44°00.22 N, 4°25.97 E | Uzés (30) | |||
30 | 29 | 29 (100) | A | 439 | 6.1 | 140 | 44°05.53 N, 4°45.73 E | Caderousse (30) | |||
31 | 29 | 29 (100) | A | 356 | 6.2 | 82 | 44°16.34 N, 4°38.45 E | Pont-Saint-Esprit (30) | |||
∑ | 607 | 239 (39) | 108 (18) | 259 (43) | |||||||
S1 | 12 | 12 (100) | A | 42°13.34 N, 1°20.43 W | Castilliscar | ||||||
S2 | 87 | 64 (74) | 23 (26) | B | 42°04.35 N, 1°08.70 W | Sadaba | |||||
S3 | 13 | 13 (100) | A | 42°13.46 N, 1°16.06 W | Ejea de los Caballeros | ||||||
S4 | 6 | 4 (67) | 2 (33) | B | 42°11.62 N, 1°10.94 W | Sabrinan (Rio loma negra) | |||||
∑ | 118 | 96 (79) | 25 (21) |
The frogs were caught by hand at night or by using modified fish traps of different sizes. We measured their snout-vent length (SVL) to the nearest 1 mm in the laboratory, using an electronic calliper. Taxon identification was based on genetic analysis of allozymes, conducted on starch gels and cellulose acetate plates using standard procedures [16] (Table 2). We used the loci 6pgdh, aat-2, gapdh, gpi, and ldh-b to distinguish R. ridibunda, R. esculenta, and R. lessonae. R. ridibunda was distinguished from R. perezi and R. grafi using the loci 6pgdh, ahh, ck, gapdh, αgdh, ldh-b, and mpr-1 [17].
Allozyme systems used to distinguish between the taxa
Enzyme | Tissue | Buffer | EC |
αgdh | Liver | TG 8.5 | 1.1.1.8 |
6pgdh | Muscle | TB 8.9 | 1.1.1.44 |
aat | Liver | TC 8.2 | 2.6.1.1 |
ahh | Liver | TG 8.5 | 3.3.1.1 |
ck | Muscle | TG 8.5 | 2.7.3.2 |
ldh | Liver | TG 8.5 | 1.1.1.27 |
mpi | Liver | TM 7.0 | 5.3.1.8 |
mpr | Muscle | TB 8.9 | undefined |
pgi | Liver | TG 8.5 | 5.3.1.9 |
pgm | Muscle | TM 7.0 | 5.4.2.2 |
A detailed ecological description of sites using 13 variables [10] showed that sites inhabited by water frogs strongly differ in their ecological characteristics and provided evidence of a gradient of freshwater influence and oxygen availability. In that study, the environmental parameters with the highest explanatory power were found to be acidity (pH), relative amount of oxygen (rel. O2), and salinity (μS), which we therefore chose to measure for the current study and habitat description. Salinity was measured as the conductivity of water to a standard KCl solution. The measures were taken twice a breeding season and site to categorize the habitats investigated here, based on the earlier reported variables [10]. We performed a PCA to describe environmentally the individual locations. Three habitat groups were defined. The first group (A) comprises habitats connected to freshwater sources (brooks, rivers, freshwater channels), characterized by low salinity, high rel. O2, and near-to-neutral pH. The second group (B) is defined by intermediate flood influence, characterized by medium rel. O2, medium to low salinity, and low pH. The third group (C) covers marshes and small water bodies more remote to freshwater sources. These habitats show a high to very high salinity, high acidity of water, and low rel. O2. The term ‘freshwater’ is used in opposition to saltwater.
We used skeletochronological analyses of bone cross-sections to determine the age of each specimen [18–20]. Skeletochronology relies on visible lines of arrested growth (LAGs) in bone cross-sections, which are built during hibernations. In water frogs, LAGs are usually very distinct, allowing a good inference of age [21]. One finger was used for skeletochronological age determination and was decalcified, dehydrated and embedded in Historesin® (LEITZ, Wetzlar, Germany). After embedding, we cut 11-μm layers using a rotation microtome and transferred every tenth cut (about 40 in total) to a microscope slide. The dried sections were then stained with cresyl-violet to visualize the LAGs and were subsequently covered with glass cover slips. LAGs were counted under the microscope at magnifications of 200× or 400×. In R. perezi, age could not be unambiguously determined for nine individuals, which have been excluded from the analysis. To analyse the differences in age between taxa, we performed a mixed model ANOVA (incomplete design;
Generally, two potential factors may affect the ratio of R. grafi to R. perezi in a population: (i) ecological conditions and habitat preferences, and (ii) the presence and absence of R. ridibunda. We employed a Kruskal–Wallis ANOVA to test for differences of the frequency of a taxon according to habitat types and Fisher's exact test to explore effects of the presence or absence of R. ridibunda on the ratio of R. grafi to R. perezi in a population or a region. All populations in Spain (S1–4) and three populations in France (1, 2, 4) are assumed to lay outside of the current distribution of R. ridibunda, as no occurrence has been reported for these regions. Populations within the range of the current distribution of R. ridibunda are populations 5 to 24.
3 Results
In the French samples, the most frequently observed taxon was the hybrid R. grafi (43% of the total sample), followed by R. ridibunda (39%) and R. perezi (18%). In the Spanish samples, only R. perezi (79%) and R. grafi (21%) were present.
All habitats dominated by R. ridibunda were of habitat type A (Table 1). In the Rhone delta, habitats dominated by either R. grafi or R. perezi included small ditches, shallow ponds, and various types of channels (habitat types B, C). The comparison of the frequency of R. perezi and R. grafi in the different populations belonging to different habitat types did not show any difference (
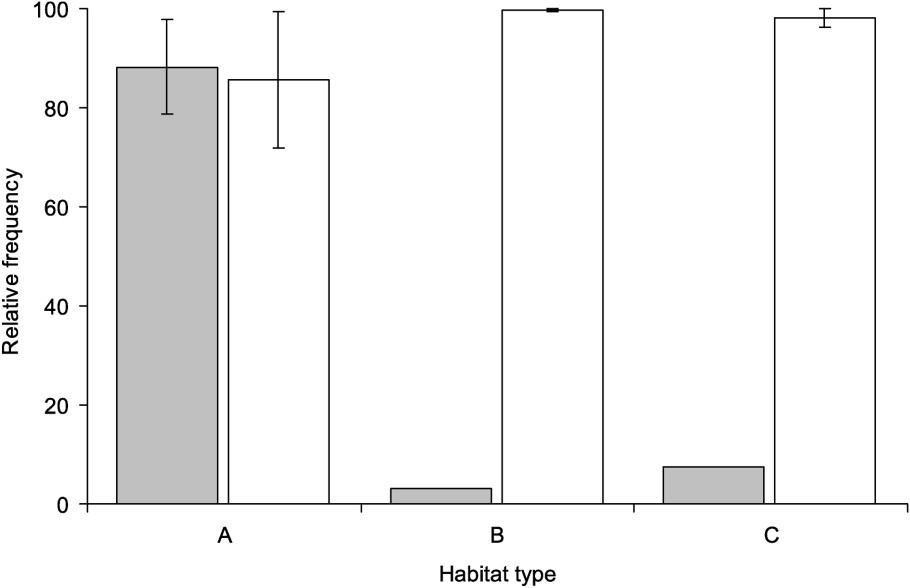
Occupation of habitat types of R. ridibunda (grey), R. perezi and R. grafi (white). The frequency differences of R. ridibunda are found to be significant, while this is not true for the combined frequency of R. perezi and R. grafi.
The oldest frog was a R. grafi specimen in its 6th year of age (
Results of the comparison of the mean snout-to-vent length (SVL) of individuals from the first three age classes of R. ridibunda (RR), R. perezi (PP), and R. grafi (RP)
Age class 1 | Age class 2 | Age class 3 | |||||||
SVL | SE | N | SVL | SE | N | SVL | SE | N | |
R. ridibunda | 53.58 | 3.80 | 16 | 62.02 | 1.91 | 24 | 68.28 | 2.28 | 5 |
R. perezi | 33.76 | 0.76 | 53 | 40.00 | 1.33 | 28 | 46.74 | 2.85 | 4 |
R. grafi | 42.92 | 1.27 | 123 | 52.00 | 1.45 | 84 | 51.12 | 2.61 | 23 |
t | df | p | t | df | p | t | df | p | |
RR×PP | 8.00 | 67 | <0.001 | 9.66 | 50 | <0.001 | 5.98 | 7 | 0.001 |
RR×RP | 2.82 | 137 | 0.005 | 3.45 | 106 | 0.001 | 2.97 | 26 | 0.006 |
RP×PP | 4.58 | 174 | <0.001 | 4.56 | 110 | <0.001 | 0.68 | 25 | 0.504 |
4 Discussion
We investigated the habitat choice of three water frog species in France and assessed the potential risk of replacement of the indigenous species R. perezi and R. grafi by introduced R. ridibunda. We found differences in habitat preferences of R. ridibunda, but not in R. perezi and R. grafi. While the latter frogs occurred in comparable frequencies in all the habitat types investigated here, R. ridibunda was found with high frequencies in habitats with high river influence. Our data is in accordance to earlier reports that R. ridibunda prefers habitats with high amounts of relative oxygen [9,22], which is assumed to be related to a high metabolic rate and low physiological performances [23]. Our results do also support an influence of the presence of R. ridibunda on the habitat use of indigenous species, especially of R. perezi, and support a real risk of replacement for indigenous species by R. ridibunda. Indeed, the higher longevity and faster growth relative to R. perezi and R. grafi, as found in our study, indicate a potentially higher competitiveness for R. ridibunda.
The competitive strength of R. ridibunda is linked to the high fertility of older females, as the number of eggs, body size, and age are strongly correlated in anurans [24–26]. Therefore, R. ridibunda will have a higher individual reproductive success than the much smaller R. perezi. Currently, it seems that the superiority of R. ridibunda may vanish under environmental conditions as given in B- and C-habitats, simply because R. ridibunda tadpoles barely survive under such conditions [23]. Our sampling in the Rhone delta suggests this, too. Despite the possibility of inhabiting numerous habitats of categories B and C in close vicinity to the river floodplains (<3 km distance), we found only a low proportion of R. ridibunda in few of such habitats. The dominance of R. ridibunda in A-habitats, however, suggests that under constant freshwater inflow and constantly high oxygen levels, R. ridibunda has to be considered a strong competitor, increasing the risk of replacement for indigenous species. In addition, the hybrids R. grafi and R. esculenta, reproductively mimicking R. ridibunda, reinforce the reproductive success of R. ridibunda by mating with that species [11,27]. This is especially important for R. ridibunda, as the sex ratio of hybridogens is biased towards females (this study, Table 1, but see also [28,29]). Hence, considering the reproductive cost to produce eggs (e.g., [30]), R. ridibunda has a cheap possibility to produce offspring by mating of R. ridibunda males with females of R. grafi and R. esculenta [11].
The distribution pattern of species and the composition of the here- investigated populations suggest that once R. ridibunda has succeeded to install itself in a habitat, the replacement of indigenous species might be rapid. Our notion is supported by the fact that mixed populations with R. ridibunda, R. perezi, and R. grafi being sympatric are rarely observed, suggesting a low detection probability. In our study, only in four of all French populations (13%), all taxa were sympatric, while in three of those populations, R. ridibunda was rare (less than 7% of the total population). Our study lacks a temporal component and we therefore cannot determine if R. ridibunda has expanded its range recently and if population 6, an assemblage of all three species, but with a high proportion of R. ridibunda, shows a replacement in progress.
Besides pointing to a real risk of replacement, the here-presented data are of importance for monitoring the distribution process of R. ridibunda in France, because earlier distribution maps may have been inaccurate, relying on species identification by morphology only, as R. ridibunda is barely distinguishable morphologically from both R. grafi and R. esculenta [15]. By contributing to knowledge on populations of genetically verified R. ridibunda, we provide future analyses with a valuable temporal component. For future studies, aiming to monitor the distribution process of R. ridibunda in France and the replacement of indigenous water frogs, it is imperative to use genetic markers.
From our study, we learn that the establishment of new R. ridibunda populations is currently restricted to habitats of type A, characterized by a high level of oxygen or at least a connection to a freshwater source, and relatively low salinity. The high genetic variability reported for R. ridibunda in the study region [31], and the gene exchange with R. perezi and R. lessonae [12], however, may allow adaptation to low-oxygen environments. In the future, an ecological shift of R. ridibunda may occur and, after a lag time, all indigenous water frog species could be under risk of extinction. For conservation of diversity in water frog assemblages, future analyses and surveys are needed to monitor the range expansion and possible ecological shift of R. ridibunda.
Acknowledgements
We thank our various field assistants, who have spent countless hours catching and monitoring frogs, and Jodie Painter (Helsinki) for correcting the language. The study was supported by the Tour-du-Valat Foundation (Arles, France). Our thanks also go to three anonymous reviewers for their valuable comments.