1 Introduction
Crops are the direct product of human selection on wild plant diversity. This domestication process has produced plants (crops) with unique features that make them suitable for agriculture. Among cereals, some of the traits commonly linked to the domestication process are larger seeds, nonshattering seeds, more compact plant development, and absence of seed dormancy. Each of these traits represents useful agricultural innovations. For instance, nonshattering crops allow a single harvest when the seeds are ripe. The development of crops has had a major impact on the organization of human societies. The domestication process is closely related to the development of sedentary human society and advanced civilisations. The timing of agricultural development coincides with the end of the last ice age from 12,000 B.P. onwards. Agriculture probably developed independently in several areas around the world, including Mesoamerica, South America, Middle East, Sahelian Africa, China and South-East Asia [1–3]. In these areas, different plants were domesticated independently. Each of these areas is the presumed birthplace of certain crops. Among cereals, maize originated from Mexico [4], wheat (einkorn and emmer) from the Middle East [5], pearl millet from western Sahelian Africa [6], japonica rice from southern China, and indica rice from the region south of the Himalayas [7]. Some authors [8] distinguish between different subregions, for instance in Africa: western Sahelian Africa, West African savannah and woodlands, West African rainforest, East Sudanese Africa and East African uplands. The distinction is mainly driven by the occurrence of different societies linked to different crops. However, whether domestication arose independently in each of these subregions or not is still an open question.
Harlan [9] counted around 360 cultivated species. The term ‘cultivated’ is rather restricted in its meaning. Several thousand plants are also collected in their wild habitats for food, fibre or medicine. However, only around 20 crops play a prominent role in the human diet, in particular cereals such as wheat, maize, rice, barley, sorghum and millet. Cultivation of these six groups of species covered 683 million hectares harvested in 2008 (Table 1), representing about half the total area under crops worldwide. Another group of crops which make a significant contribution to our carbon diet are root and tuber crops like cassava, potatoes, yams and sweet potatoes. These different cereals, root and tuber crops are the main starch component of the human diet.
Area of cultivated land under the main cereal crops.
Crop(s) | Cultivated land in millions of hectares |
Wheat | 224 |
Maize | 161 |
Rice, paddy | 159 |
Barley | 57 |
Sorghum | 45 |
Millet | 37 |
In this article, we illustrate how the diversity found in a cultivated crop has been continuously shaped by demographic as well as by selection processes. Understanding the diversity of cultivated plants today requires an understanding of the impact of the domestication process as well as that of ongoing human actions. We illustrate these points with the example of maize domestication up to the development of hybrid varieties in the 1930s. We then review some recent studies focusing on the impact of farmers on crop diversity today, which is most pronounced in smallholder agricultural systems. Finally, we show that selection continues today, using the example of the adaptive response of pearl millet to climate variation.
2 Maize history: from domestication to the development of hybrids
Maize is a good example of the history of the domestication process because of the spread of this cultivated plant at continental and worldwide scales. Phylogenetic analyses point to the Balsa River basin in Mexico as the area where the closest living relatives are found [4]. Genetic data suggest domestication occurred 9200 years B.P. with a wide confidence interval (5600–13,000 years B.P.). The oldest cob was found in Mexico and dates back 6250 years [10]. However, a recent archaeological study based on starch grains and phytoliths suggests maize was cultivated earlier, at around 8700 B.P., in the Balsa River basin [11]. Both archaeological and genetic analyses have provided evidence for the very early domestication of maize in Mexico around 9000 B.P. (Fig. 1). Moreover, these studies suggest that the domestication process took place in the Balsa River basin. The closest plant related to today's cultivated maize is Zea mays ssp parviglumis, a teosinte which grows at low elevations. Another teosinte (Zea mays ssp mexicana) prefers higher elevations and is also found as a weed in fields of maize [12]. A scenario for the origin and the spread of maize across America has been proposed based on several genetic studies [4,13]. Probably the initial cultivation of maize spread quickly at both high and low latitudes in Mexico and from there to lower altitudes in the tropics (i.e. Guatemala and the Caribbean). Later on, cultivation rapidly spread to South America and the Andes (Fig. 1). A recent study identified maize starch residues dating back to 5300 years B.P. in Ecuador [14]. Maize spread to North America later. Maize residues dated 3200 years B.P. were found in south-eastern USA, but maize became a staple crop in north-eastern America only 1200 years ago [15]. After the arrival of Europeans in the Americas, maize and other American crops migrated from the Americas to Europe and subsequently worldwide. One of the first painted images of maize in Europe is in the Villa Farnesina in the suburb of Rome (Italy) [16]. This painting dates from 1515 to 1517 A.D., i.e. 22 to 24 years after Christopher Columbus’ voyage to the American continent [16]. It is still possible to visit this villa today, and the painted ceiling illustrates the luxuriant fruits and crops that one would certainly expect in Eden.
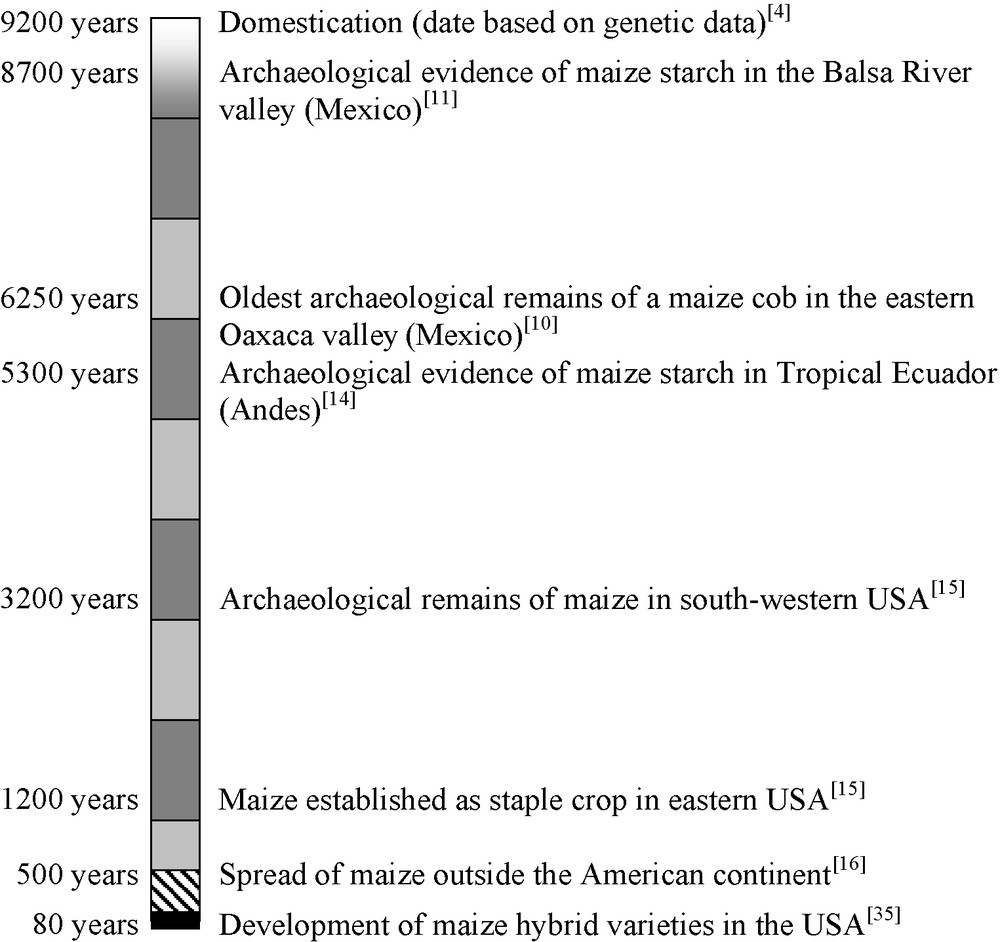
Agricultural history of maize from domestication to the development of hybrids. Key events from domestication to the development of hybrid varieties.
3 Impact of human selection on maize diversity
A large number of alleles were selected during the domestication process and the spread of maize [17–20]. However, the number of genes that we can call domestication genes is quite low [21]. Two of these genes were recently cloned [22,23]. One of the first ever genes cloned after its identification by quantitative trait locus (QTL) analysis is the domestication gene Teosinte branched 1 (tb1). This gene belongs to the TCP transcription factor family. The cultivated allele of tb1 shows a high level of expression [22]. A genetic study found that in cultivated maize, diversity at the 5′ of tb1 is reduced to almost a single haplotype [22]. This low diversity is observed in a window of 80 kb upstream from tb1. One likely explanation for this pattern is the strong reduction of recombination [24] in noncoding regions (up to 100 times lower than in coding region) and selection for a modification in the regulation of the gene in noncoding regions. Such a region was identified 58–69 kb upstream from tb1 [25]. To date, the exact molecular mechanism and mutation in the noncoding region of tb1 is unknown. Mutations related to the cultivated haplotype were also been identified in all the archaeological remains analyzed [26]. This result suggests that selection for the maize tb1 allele was an early event during the domestication process. Another recently identified domestication gene [23] is Teosinte glume architecture 1 gene (tga1). This gene is partly responsible for the absence of a seed coat in cultivated maize. In Teosinte, the seeds are enclosed in an enduring tegument which makes them difficult to harvest and process. In maize, the seeds are accessible and easily harvested, thanks to the limited development of the tegument. The likely (though not totally proven) polymorphism linked to this modification is a single amino-acid change [23]. The tga1 gene also belongs to a transcription gene family, the squamosa promoter binding protein family.
Human selection was not only linked to the domestication process per se but also to the resulting spread of maize to different climates which, in turn, resulted in the selection on new traits. Maize is a tropical plant and varieties found in Mexico generally do not flower in North America. So during the spread of maize, new genotypes were selected for their ability to flower at high latitudes. Some of the flowering time genes linked to maize adaptation to high latitudes were recently identified [27,28]. Different seed qualities were also selected [29–32]. For example, sweet corn was selected relatively early during the spread of maize [26]. Some specific traits were also selected very recently, such as the yellow/orange endosperm phenotype. The ancestral colour of endosperm in maize is white [33]. During the 20th century, the yellow/orange colour of the maize endosperm was selected to increase carotenoid content [33]. Most modern varieties harbour the yellow/orange phenotype but traditional varieties present a wide range of seed colours from white, dark red to blue. The recent selection of yellow/orange seeds resulted in depleted diversity at one particular allele at the Y1 locus [33]. In maize, although human selection has rarely had an impact at the chromosome scale [34], observed diversity is mainly driven by demographic effects rather than by selection for specific traits [18,19].
4 Demographic impact on maize diversity
One consequence of the domestication process on diversity is the genome-wide demographic effect. By choosing individuals that are morphologically adapted to agriculture, farmers established new populations based on a limited number of genotypes. This founding effect increases drift intensity and has a genome-wide impact on the diversity of cultivated populations, which is usually less than the wild ancestor of the crop. Several authors investigated genome-wide diversity to understand how selection and demography explain the diversity found in cultivated maize [18,19]. These studies showed that 2 to 5% of maize genes display evidence of selection during or after the domestication process. This result suggests that human selection has influenced a relatively high number of genes. However, at the genome scale, the demographic process linked to maize domestication is the main phenomenon explaining the diversity observed today. Based on DNA sequence studies, the diversity in traditional varieties represents 57% of the diversity observed in the wild ancestor [19].
The diversity of cultivated varieties in Northern America and in the Andes was considerably reduced during this diffusion/demographic process. In traditional varieties of maize, the highest diversity is found in Mexico and in tropical lowlands [13].
After the 1930s in the United States, the average yield of maize increased [35]. The average yield of 1.8 T/ha in 1930 doubled in the 1950s and had reached 8 T/ha by 1995 [35]. An increase in yield is the result of a combination of different factors including the development of improved varieties, the development of more intensive agronomic techniques (the use of fertilizers and herbicides) and not cultivating maize on less productive land (in south-western United States, for example). Only five traditional maize varieties represent 87% of the genetic basis of hybrid varieties [35]. A single variety, ‘Reed Yellow Dent’, contributes 47% of the gene pool used for the creation of hybrid varieties. So the current diversity in the cultivated varieties is significantly less than the initial variability in traditional varieties. Moreover the hybrid Reed Yellow Dent is based on northern and southern American landraces, i.e. local varieties. These varieties already displayed less diversity than landraces located in the tropical lowlands or highlands of Mexico [13].
Maize is an example of the patterns of diversity observed in all other major crops [36]. The advancement of agriculture industrialization led breeders to concentrate on a few genotypes and to the development of improved and hybrid varieties. This approach led to standardization of the diversity in farmers’ fields [36]. The decrease in the diversity of varieties used for agriculture mainly occurred in the most developed countries which adopted very intensive agricultural systems. In developing countries, smallholder agriculture still boasts high diversity [37]. This diversity is continuously shaped both by demographic and selection processes.
5 Human action and diversity in smallholder agriculture
Despite the expansion of modern agriculture, today millions of hectares are still under smallholder/traditional farming systems [38]. In modern agriculture, farmers have no impact on plant evolution because they rely on seed suppliers, while in traditional agriculture farmers are still directly involved in plant evolution because they regenerate their own seeds. These agroecosystems are mainly self-reliant and self-provisioning as far as planting material is concerned [39]. As a result, farmers’ practices play a key role in shaping the genetic structure of crops and their evolution [40]. In traditional farming systems, in which farmers had limited access to inputs and pesticides, they relied on wide infra-specific diversity to cope with the risk of crop failure owing to climatic vagaries, diseases, pests, and soil limitations [39,41]. This diversity also is often culturally important. A worldwide survey of on-farm crop diversity of 27 major food subsistence crops showed that wide crop genetic diversity is still maintained on farms through the use of landraces [37]. High levels of genetic diversity are indeed common features of both sexually and clonally propagated crops. For instance, 52% of the allelic diversity of a worldwide core collection of sorghum (Sorghum bicolor ssp bicolor) was found in a single village in northern Cameroon [42]. Similar results were obtained for clonally propagated crops and up to 74 yam landraces (Dioscorea rotundata) were found in a village located in northern Benin [43].
Farmer practices strongly affect the dynamics of genetic diversity of crops by acting on drift, selection, and gene flow. Each year, farmers choose their planting material among their harvest. This selection has a direct impact on genetic diversity and its structure. Furthermore, farmers only need a rather small proportion of their harvest for the next growing season, a feature that generates genetic drift. For instance, maize farmers in Mexico use less than 100 spikes per landrace, leading to a high probability of loosing alleles present at a frequency of less than 5% [44]. In addition to their harvest, farmers still rely on local seed systems to acquire new material. It is notable that seeds are not randomly exchanged. Several studies showed that farmers prefer to acquire seeds from farmers in the same community [43,45,46]. These exchanges are based on trust or are part of the global exchange system of the society concerned (seed inheritance and weeding). Finally, farmers have an impact on pollen flow through the spatial organization of their fields. Unlike in modern agriculture, finding several landraces mixed in one field is not rare [47]. The impacts of these practices vary to a great extent depending on the reproductive system of the crop.
Nowadays, wild relatives are still found in sympatry with crops, specifically in domestication centres, leading to natural hybridization and introgression [48–50]. These gene flows may be a source of new genetic combinations that are valuable for the adaptation of the crop [50]. However, they can also threaten crops by increasing weediness of wild populations and by decreasing the genetic integrity of crops. Ultimately, gene flow is highly influenced by the farmers’ management of wild plants. For instance, wild beans (Phaseolus vulgaris) are harvested and stored together with cultivated beans, and may thus be sown inadvertently [51]. Farmers can also voluntarily use these gene flows by selecting and introducing hybrid yams (Dioscorea spp.) in their fields [52]. Conversely, farmers actively select sorghum against hybrid morphotypes in Cameroon, because a high frequency of hybrids in fields is believed to result in lower yields [53].
As outlined in this article, farmers’ management practices have a major impact on evolutionary processes. Farmers’ practices are influenced by agronomical considerations in addition to the traditional ecological knowledge associated with the crop concerned. This knowledge could be the most fragile component of agricultural systems and is subject to rapid change. One of the important challenges in agriculture today is facing a major increase of food demand in developing countries. Smallholder agriculture needs to increase crop yields and to adapt to rapid societal and environmental changes. Among environmental changes, climate change is expected to have a serious impact in the near future. Understanding how crops and society respond to climate change is of utmost importance to allow adaptation of agriculture to future conditions.
6 Crop biodiversity and adaptation to climatic variation: the example of pearl millet
The diversity observed in traditional agriculture could be a significant asset to respond to environmental variability. Here, we describe a study in the Sahel region of Niger, one of the driest agroecosystems in the country. Niger is characterized by a very strong population growth rate, with a doubling of its population every 20 years (Fig. 2). There has been no increase in yield to match the needs of this growing population, but there has been an increase in the area used for the cultivation of the two main crops, sorghum and pearl millet (Fig. 2). Pearl millet covers 65% of the cultivated area, followed by sorghum. Pearl millet is an allogamous crop which was domesticated in Africa. In Niger, farmers mainly use their own seeds the following season [54]. In the case of seed shortage, farmers rely on seeds from family members and as a last resort, on seeds found in local markets [46,54]. The crop is rainfed during the monsoon season from June to September. In the wetter part of Niger, the rainy season can last four months, while at the northern limit of rainfed agriculture; it rarely lasts more than two months.
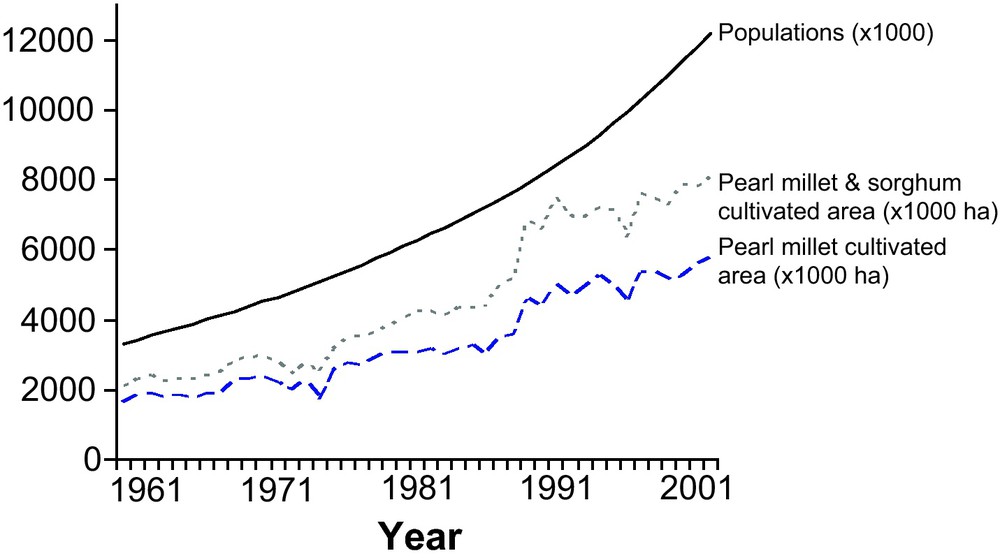
Evolution of cultivated area and population size in Niger. The increase in the human population and in the cultivated area of pearl millet and of pearl millet and sorghum combined. The population increased from 3.5 million inhabitants in 1961 to 12 million in 2003. The cultivated areas of the main cultivated crops followed the same trend, with a slight tendency to level off in the last decade (Source FAO-Stats).
In the early 1970s, a major change in rainfall was observed in the Sahel. Several droughts occurred in the early 1970s, and in the 1980s and 1990s. The Sahel climate is characterized by very strong interannual variability (Fig. 3), but from 1950 to 2003, rainfall decreased by 4 mm per year in Niamey (Niger), i.e. a total of 200 mm in 50 years. The same trend was observed throughout the Sahel region for the same period, generally with a decrease in rainfall occurring after the 1970s.
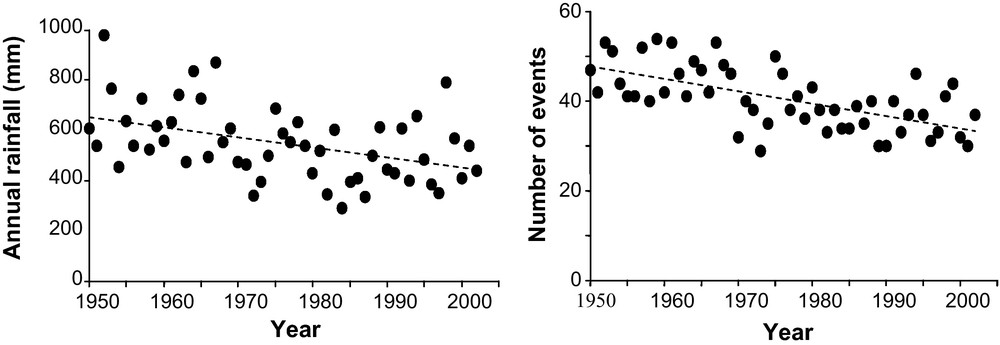
Analysis of rainfall and number of rainfall events over a yearly time course. Annual rainfall in mm and the number of rainfall events of 1 mm or more for the period 1950 to 2003 in Niamey (Niger). Annual rainfall was correlated with the year using a generalized least square fit (A). Correlation between errors was 0.0067 (95% CI–0.27 to +0.28). The regression coefficient of the correlation was −4.0 (t = −3.3, p < 0.002). The decrease in rainfall was 4 mm per year giving a total decrease of 200 mm in 50 years. The number of rainfall events of 1 mm or more also decreased, the regression coefficient was −0.27 (t = −5.71, p < 0.001). The between error correlation was 0.077 (95% CI–0.20 to +0.34). In 50 years, the number of annual rainfall events of 1 mm or more decreased from 50 to 37.
Adaptation to climatic variation can proceed by the adoption of new varieties, the spread of varieties adapted to particular conditions (e.g. early varieties), or adaptation of varieties to a fluctuating environment. Pearl millet and sorghum varieties were sampled in 1976 and in 2003 (Niger) to assess the consequences of environmental change [55]. The comparative analysis was based on a very large dataset of 612 accessions for pearl millet and 741 accessions for sorghum. One surprising result was that there was no significant change in the relative frequency of the different sorghum and pearl millet varieties sampled in Niger in 1976 and 2003 [55,56]. However, some varieties showed some minor changes in their distribution. No genetic erosion was observed between 1976 and 2003 for pearl millet (Fig. 4) or sorghum [56].
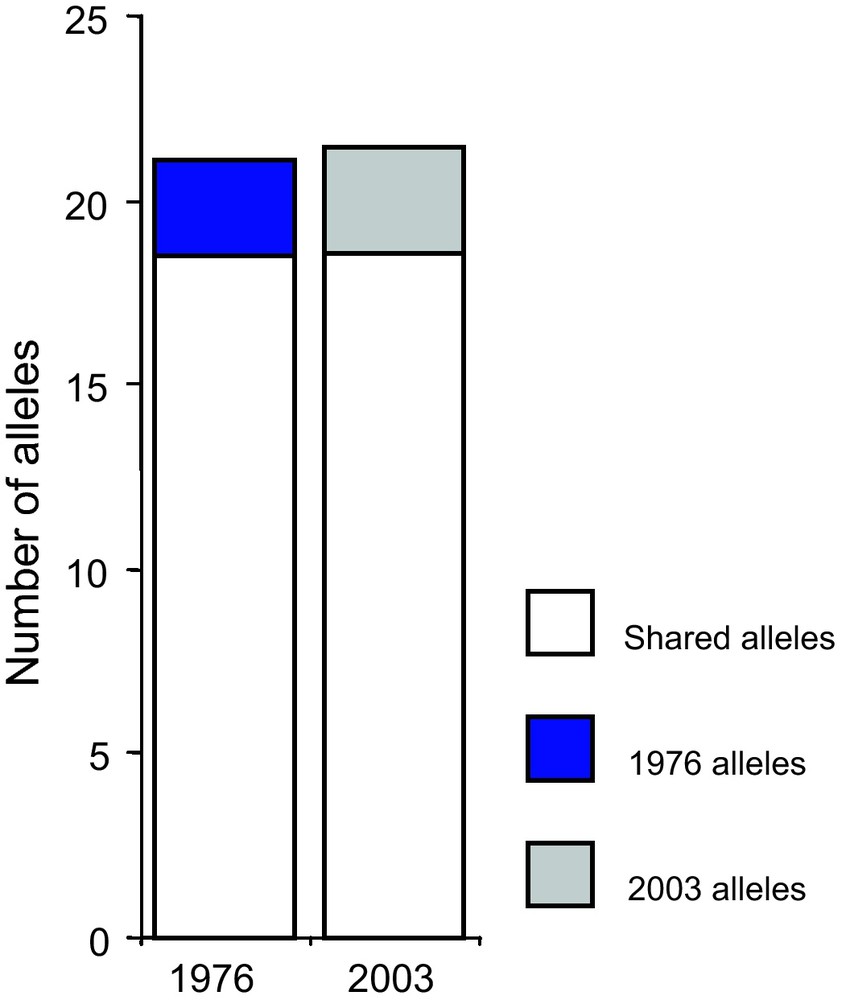
Evolution of allele numbers between 1976 and 2003 for pearl millet. Genetic diversity of samples collected in 1976 and 2003 was compared using 25 microsatellite markers [57,58]. The figure represents the total number of alleles and the number of alleles specific to a given sampling year (1976 or 2003). Overall, we found no differences in gene diversity and allele number between 1976 and 2003 (p = 0.27 and p = 0.08 respectively).
Samples of pearl millet accessions collected in 1976 and 2003 were evaluated in three seasons (2004, 2005 and 2007) in a common garden experiment in Sadore (Niger) to asses changes in morphological and phenological characteristics (flowering time, plant height and spike length) [57,58]. The survey showed a significant shift in adaptive traits in pearl millet: in 2003, varieties flowered slightly earlier and had shorter spike than in 1976 [59,60], suggesting an evolutionary change. Two genes linked to variation in flowering time were recently identified in pearl millet: PHYC [59] and PgMADS11 [60]. Polymorphisms for early and late flowering alleles are present in the varieties from Niger for these two genes [59,60]. Both genes exhibit a selection signature [59,60] suggesting they play a role in the adaptation of pearl millet varieties. Moreover, some recent results suggest that PHYC is associated with the changes in flowering time between 1976 and 2003, supporting the hypothesis of an evolutionary change [57,58]. The presence of standing variation for PHYC and PgMADS11 genes and certainly for many other genes may explain this rapid response to selection.
As flowering time is correlated with annual rainfall (Fig. 5), taken together, these results suggest adaptation of pearl millet to climatic variation. This change results from the product and the interaction between human and natural selection. Increased temperature and/or a decrease in rainfall are among likely candidates for environmental selection but their respective roles remain to be determined. In the context of climate change, a shorter life cycle may mitigate the effect of climate change by allowing flowering and seed production in drier environments than 30 years ago. Diversity among varieties is certainly an asset for adaptation to climatic variation.
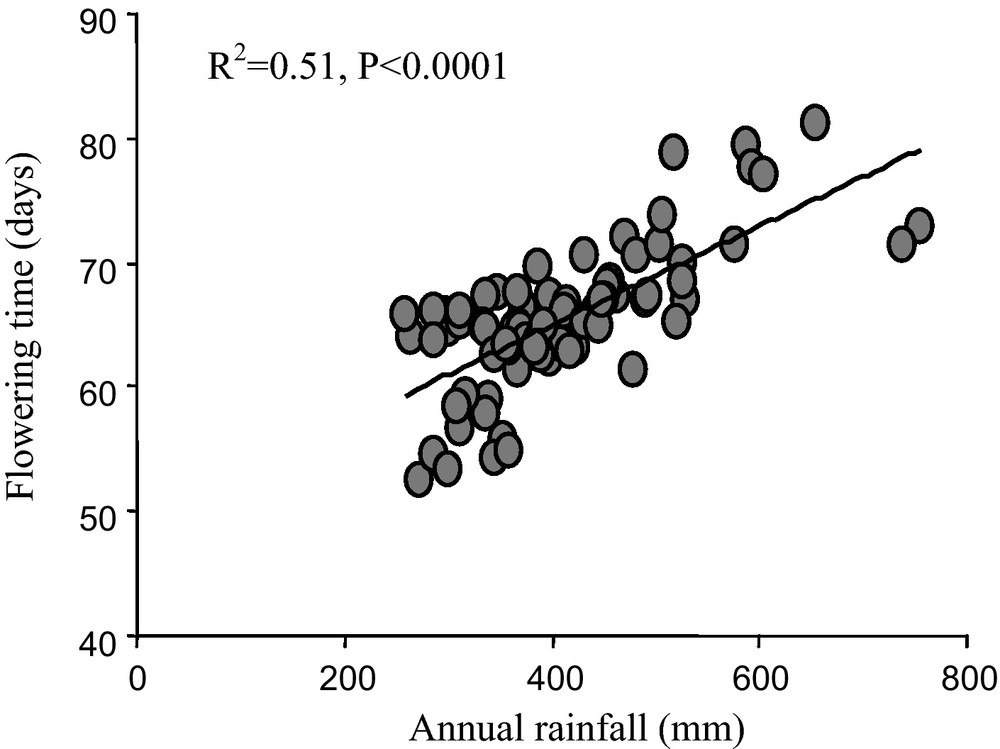
Average flowering time and correlation with annual rainfall. The average flowering time for 79 villages (listed in [55]) in Niger was calculated as the mean flowering time of the varieties collected in a given village in 2003. The annual rainfall was calculated based on rainfall data for the period1976–2003. There was a significant correlation between annual rainfall and flowering time (R2 = 0.51, p < 0.0001).
7 Conclusion
Domestication has had a significant impact on crop diversity associated with both the demographic history of cultivated crop and the selection of useful agronomic traits. Crops have spread from their original area of domestication to their present area of distribution, sometimes worldwide. Demographic events as well as new selections shape the diversity during this diffusion process. In developed countries, diversity has been considerably reduced by the selection of a few high yield genotypes. In developing countries, smallholder agriculture continues to maintain high genetic diversity in the fields. This diversity is shaped by the history of each crop, but is also continuously shaped both by human action and selection. Understanding the diversity present in farmers’ fields today requires the analysis of all the different steps in the history of the crop from domestication to in situ evolution in farmers’ fields today. The observed diversity in (and among) crops grown by smallholder farmers is certainly a significant asset to cope with a fluctuating environment. West Africa is an area where the prediction of future climate is still largely fuzzy (Intergovernmental Panel on Climate Change [IPCC]). Given such uncertainty, increasing yield while maintaining biodiversity in agroecosystems, the associated practices and knowledge, is certainly a good way to cope with the problems that will affect agriculture in the 21st century.
Disclosure of interest
The authors declare that they have no conflicts of interest concerning this article.
Acknowledgements
This study was supported by Agropolis Foundation through the Resource Center for Crop Conservation, Adaptation and Diversity project (ARCAD 2007–2013 to Y.V. and A.C.T.); and Agence Nationale de la Recherche grant (ANR-07-JCJC-0116-01 to Y.V.). We thank the two reviewers, Dr Décamps and Dr Lebreton for their helpful comments.