1 Introduction
Most coral reef organisms (i.e., molluscs, fish and crustaceans) have stage-structured life histories with two distinct stages: a benthic stage (usually juveniles and adults); and a pelagic larval stage capable of long-distance dispersal that can last for weeks or months [1]. One of the greatest challenges facing the majority of marine organisms with larval stages that potentially disperse and develop in offshore waters, is how to relocate patchily distributed reef environments in a vast oceanic matrix. Indeed, the replenishment and persistence of most reef marine species depends on larvae finding suitable adult habitat at the end of the offshore dispersive stage [2,3].
The sensory and swimming capabilities of marine larvae have frequently surprised researchers in recent years [4–6]. Many marine species can swim either at hatching or soon thereafter, irrespective of the presence of environmental cues. Nevertheless, they have the potential to use these chemical and/or sound cues to control their position within the water column; increasing the probability that they will be transported to a suitable reef [3]. Once a larva of any taxon has located a reef, a suitable micro-habitat (small spatial scale ranging from one centimetre to tens of meters) on which to settle must be found. Recent studies have highlighted the role of larval sensory mechanisms in patch identification and patch selection at small spatial scales, including the detection of visual, chemical and sound cues from conspecifics or micro-habitats (e.g. among patches separated by centimeters to meters within a single reef) [7–10]. Overall, while evidence is mounting that larval reef fish and crustaceans are active participants in the process of dispersal and settlement due to their sensory and swimming abilities, little information is available about the habitat selection by cephalopod species settling on coral reefs [1,11].
In the present study, laboratory experiments were conducted to determine sensory mechanisms in habitat selection of sepioid larvae (Euprymna scolopes – [12]) captured at the Rangiroa atoll (French Polynesia). Sepioids occur on the continental shelf and upper continental slope of tropical and temperate areas in all oceans [13,14]. However, the biology and ecology of the sepioids are generally poorly known, especially on coral reefs [13]. Yet, many of the larger species of sepioids are important to fisheries in the Pacific [15]. The term “larvae” (or presettlement larvae) was used for the sepioids tested [16].
2 Material and methods
2.1 Study site and larvae capture
This study was carried out on the North coast of Rangiroa lagoon, French Polynesia (14°57’48 S, 147°38’79 W). Crest nets were placed on the reef crest of Rangiroa in order to capture sepioid larvae just before they entered the lagoon to settle [17]. Several papers showed that fish, crustacean and mollusk larvae caught with crest nets on the reef crest are competent to settle on reefs in the 24 hours following the colonization [1,5,8,17]. A total of 30 sepioid larvae (E. scolopes) were captured in April 2008. Larvae captured during the night were collected at dawn, transferred to the laboratory, and subsequently maintained individually in aquaria (0.3 × 0.2 × 0.2 m).
2.2 Experiment 1: habitat preferences
To test the habitat preferences of E. scolopes larvae, an aquarium (0.6 × 0.4 × 0.2 m) containing the four habitats (sand, macroalgae, living coral and dead coral) was built. The four different micro-habitats tested (i.e. the four main settlement habitats of marine species in coral reefs – [18]) were: spherical living coral colonies (5 cm radius) of Pocillopora sp. and Acropora sp., spherical dead coral colonies (5 cm radius) of the same two species, spherical piles of living macroalgae (Sargassum mangarevasae) with a radius of 5 cm and spherical piles of sand with a radius of 5 cm.
The aquarium was divided into four quadrants (0.2 × 0.2 m) in size, and separated into two pairs by a central area [19]. For each trial (one larva per trial), each of the four habitat types were randomly assigned to the four quadrants. Larvae were introduced one at a time to the centre of an aquarium (equidistant from the four habitat patches) via a small net. After 1 min of acclimation, the net was removed and larvae were free to choose among the four available habitats. Individuals were continuously observed, and a “choice” was recorded as the habitat upon which the individual first settled (after any initial exploration) and subsequently remained for at least 1 min. If the larvae were still in the central part of aquaria after 5 min, the trial was stopped (scored as “no choice”). After each trial, seawater was replaced and habitat types reassigned to quadrants. The distribution of choices for 15 larvae (15 trials) was recorded and analyzed with Chi-square tests.
2.3 Experiment 2: effect of conspecifics
One larva (different from those used in Exp. 1) was introduced into the centre of one of the aquaria used for the habitat selection experiment (Exp. 1), but with the plexiglass partition removed resulting in only two quadrants. Both quadrants contained the same quantity of the most preferred habitat type (determined from Exp. 1) and five older conspecifics were added to one quadrant. The conspecifics, used as cue transmitters, were juveniles of E. scolopes caught with crest nets and maintained in aquaria over 10 days. The conspecifics remained associated with the habitat patch, and thus the quadrant to which they were assigned. Choices were recorded for 15 larvae. As with Experiment 1, water was replaced and the positions of the treatments were randomized for each trial. The distribution of choices exhibited by larvae was analysed with Chi-square tests.
2.4 Experiment 3: sensory modality
To test the sensory modality of E. scolopes larvae, an experimental arena was built. The arena consisted of a large rectangular aquarium subdivided into three inter-connected compartments along the long-axis by transparent panels, and with two additional smaller aquaria at each end (Fig. 1). Two types of sensory modes (vision and olfaction) were tested on two cue types (heterospecifics vs. conspecifics; and most preferred habitat vs. least preferred habitat according to the results in Exp. 1). To test the potential role of vision on choice, competing stimuli (conspecifics vs. heterospecifics and habitat 1 vs. habitat 2) were randomly assigned to tanks 1 and 2. To test the potential role of olfaction, ten liters of seawater in which conspecifics vs. heterospecifics and the two habitat types had been immersed for three hours were randomly assigned to compartments B or C (Fig. 1). Sepioid larvae were introduced into compartment A, and subsequent movement of the test subject into the adjoining compartments (B or C) within 5 min was scored as a “choice”, whereas retention in the central compartment was scored as “no choice”. Each larva used in the experiment was also tested in a control where no sensory stimuli were introduced to the aquaria. The order of experimental exposure and control were randomly assigned to avoid order effects.
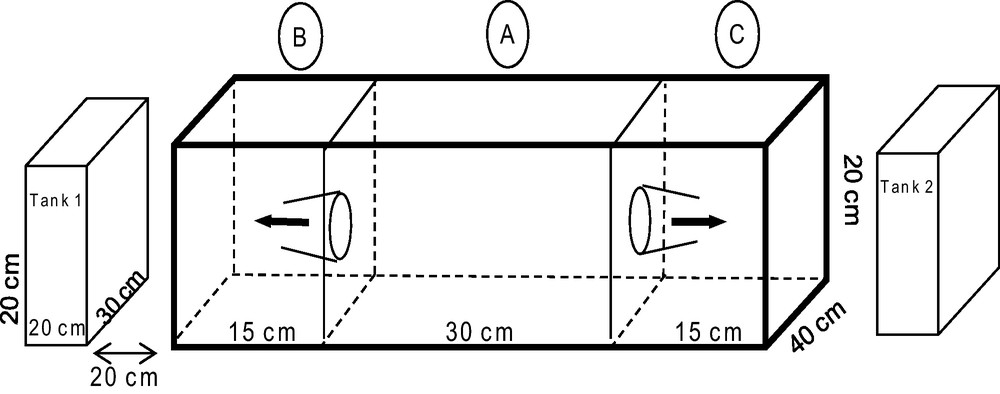
Experimental arena set up used to evaluate visual and chemical cues underlying habitat selection. The arena consisted of an aquarium with three compartments (A, B and C) interconnected via funnels. Additional tanks on either side of the aquarium with three compartments (no. 1 and 2) were isolated from it and mounted upon separate platforms to prevent the transfer of vibratory signals.
Experiment 3A tested the response of sepioids to conspecifics (five E. scolopes juveniles) versus heterospecifics (five juvenile crabs: Calappa calappa that were readily available at the time of the experiment), using the 15 larvae that had been used in Experiment 1. If predators, prey or competitors of E. scolopes were used as heterospecifics (instead of C. calappa), so when E. scolopes larvae moved toward compartment B (side of conspecifics), we could not know if this movement was due to attraction by conspecifics or repulsion by heterospecifics. Therefore, C. calappa was used as heterospecifics as there was no repulsion on E. scolopes larvae.
In Experiment 3B, the response of sepioids to the most preferred versus the least preferred habitat (according to the results in Experiment 1) used the same 15 larvae that had been used in Experiment 2. For each experiment, a Chi2 test was carried out to compare the observed distribution to the control distribution (number of larvae in compartments A, B and C in the absence of external effects on the aquarium system).
3 Results
3.1 Experiment 1: habitat preferences
Eighty-six percent of E. scolopes larvae made active habitat choices: dead coral (40%), then living coral (20%), macro-algae (13%) and sand (13%) (Table 1A). However, this distribution did not differ significantly from the null distribution (equal repartition of larvae among the five options: four habitats and “no choice” – Chi2 test: X32 = 4.0, p-value = 0.31).
Percentage of sepioid larvae (15 individuals tested) A that chose each of the four habitat types (living coral, dead coral, macro-algae and sand); B that chose between the presence or absence of five conspecifics on dead coral habitat (the preferred habitat in Experiment 1). If larvae were still in the central part of aquaria after 5 min, the trial was stopped (scored as “no choice”).
A | |||||
Living coral | Dead coral | Macroalgae | Sand | No choice | |
% of sepioid larvae | 20 | 40 | 13 | 13 | 14 |
B | |||||
Dead coral without conspecifics | Dead coral with conspecifics | ||||
% of sepioid larvae | 26 | 74 |
3.2 Experiment 2: effect of conspecifics
E. scolopes larvae discriminated between the presence or absence of conspecifics on their preferred habitat (X12 = 5.4, p-value = 0.02). Seventy-four percent of sepioid larvae were attracted by their preferred habitat (dead coral) with conspecifics (Table 1B).
3.3 Experiment 3: sensory modality
In the absence of added stimuli, most E. scolopes larvae remained in the central compartment (exhibiting “no choice”) and the remaining larvae (which did display a “choice”) showed no preference between compartments B versus C (all X12-values < 3.84, p-value > 0.05, Fig. 2A,B). In Experiment 3A (Fig. 2A), the distribution of larvae when presented with visual or olfactory cues separately was significantly different to the null distribution (X22 = 21.9, p-value = 0.001 for visual cues; X22 = 12.2, p-value = 0.005 for chemical cues). E. scolopes larvae preferred conspecifics over heterospecifics with both visual and olfactory cues. In Experiment 3B (Fig. 2B), the distributions of larvae were similar to the null distribution when presented with visual or olfactory cues separately (X22 = 0.4, p-value = 0.81 for visual cues; X22 = 0.5, p-value = 0.73 for chemical cues). E. scolopes larvae did not differentiate between their preferred habitat (dead coral) versus the least preferred habitat (sand) from Experiment 1.

Percentage of sepioid larvae presented with no cue (unshaded bars) or two competing cues (visual or chemical cues - gray and black bars). A. Choices offered in compartments B and C are indicated by “conspecifics” or “heterospecifics”. B. Choices offered in compartments B and C are indicated by “preferred habitat” (the most preferred habitat in Experiment 1) or “other habitat” (the least preferred habitat in Experiment 1). “No choice” refers to sepioid larvae that remained in compartment A (see Fig. 1). The star represents a significant attraction of sepioid larvae toward conspecifics.
4 Discussion
The present study showed that sepioid larvae settled mainly on dead coral (Table 1A), and the presence of conspecifics on the settlement habitat influenced their selective choice (Table 1B). Sensory experiments showed that E. scolopes larvae differentiated between conspecifics and heterospecifics (and not between their preferred habitat versus the least preferred habitat) using both visual and olfactory cues (Fig. 2). These results must be, nevertheless, taken with caution as some experimental artefacts may result from enclosing marine species in aquaria [8,19].
It is highly unlikely that marine larvae possessing a pelagic phase of development in open water (mainly fish, crustaceans and cephalopods), can successfully settle to suitable habitat solely as a matter of chance [18]. In fact it is known that many such marine larvae have swimming abilities sufficient to control their pattern of oceanic dispersion and their return to adult habitat [1]. However, these swimming abilities must be directed by choices made according to sensory detection of the surroundings in order for larvae to successfully arrive at their settlement habitat. In previous experiments, Lecchini et al. [8,9,19] showed that larval fish and crustacean species used visual, chemical and/or acoustic cues to recognize their settlement habitat. Yet, until now, the coleiod cephalopods (octopuses, cuttlefishes, squids and sepioids) have been considered to be primarily visual animals. In behavioral reviews of cephalopod behaviour, emphasis has been placed on the importance of visual body patterning for social communication while the significance of chemical cues has been largely ignored [20,21]. Chemotaxis has been demonstrated in some cephalopods at adult stage using chemicals related to foods or for the purposes of reproduction [20–23]. Our study has highlighted, for the first time, that squids at larval stage could use both visual and chemical cues to detect and recognize their conspecifics present on the settlement habitat.
Thus, our sensory experiments showed that sepioid larvae were attracted only by conspecifics (and not by the habitat types). In previous experiments, Lecchini et al. [8,9] showed that 25 larval fish species were attracted to visual and chemical cues emitted by conspecifics compared to the habitat itself (coral or algal habitat). Similarly, grasshoppers [24] and territorial lizards [25] use the presence of conspecifics to evaluate the potential quality of particular microhabitats. This social aggregation with older conspecifics may be the result of individuals using conspecific guides to find potentially beneficial resources [8]. Indeed, marine larvae suffer high predation rates immediately following settlement (60–90% of larvae disappeared by predation in the first post-settlement month) [18,26]. This mortality rate can vary markedly according to characteristics of the habitat, but is often reduced in the presence of conspecifics in the settlement habitat [27]. The presence of conspecifics could be an indicator of the quality of the habitat (e.g., availability of resources and low mortality) [18,28].
Overall, although it is still not known how sepioid larvae find their way back to a particular reef, their differential ability to respond to visual and olfactory stimuli from conspecifics is clearly implicated in this paper.
Disclosure of interest
The author declares that he has no conflicts of interest concerning this article.
Acknowledgements
This research was supported by grants from ANR (ANR-06-JCJC-0012-01), MOM (06 PF 15), CRISP program (Coral Reef Initiatives in the South Pacific – C2A1).