1 Introduction
To understand the functioning of different phenomena in planarians, it is crucial to study, at first, the expression of several genes using classic but essential techniques such as PCR, RT-PCR, and in situ hybridization (ISH). The frequently used species were Dugesia japonica, Dugesia tigrina and Schmidtea mediterranea, but in our work we have focused on the Tunisian specimen Dugesia sicula Lepori, and therefore this extends molecular research. This work is considered as the mandatory stage maintained before carrying out specific studies on this selected species. Planarians are the best model to understand the regeneration phenomenon that fascinates humans since decades. For this reason, we targeted stem cells, responsible factors of regenerative property in flatworms [1]. We choose DjFoxG, since it is the first gene found in planarian stem cells populations [2]. It belongs to the Fox gene family, whose name is forkhead box, and it is related to the Drosophila gene fork head, corresponding to transcription factors [3]. Then we targeted the central nervous system (CNS); there are several genes having major roles in the CNS, such as the neural and cervical ones, coding for protein receptors, hormones, peptides, and others [4]. The DjPC2 gene was selected and it is a neuropeptide expressed profusely in the planarian central nervous system and previously found in the sensory and motor neurons clusters [5]. It is affiliated to the subtilisin/kexin family containing the precursor convertases, possessing activities in endocrine and neuroendocrine systems [6]. DjotxA is a good visual cells marker, it was expressed in the cephalic ganglion, but more medially in the termini of the visual axons in D. japonica [7]. Finally, we targeted the aspartyl protease Cathepsin-D gene to stain the digestive system of D. sicula. This protease acts in the process of hemoglobin digestion by schistosomes after blood feeding from humans [8], as it contributes to protein digestion in the gut of planarians [9].
2 Material and methods
2.1 Planarians
D. sicula specimens were provided by Dr. Mohamed Charni, as explained previously [10,11] and maintained as described by Molina et al. (2007) [12] as currently for D. japonica due to their phylogenetic rapprochement [13]. We denote the specimens collected from the Joumin dam by s1 and from Boussadia by s2.
2.2 In silico sequences analysis
Several software tools have been exploited in our work. The alignment concerns only the species maintained in the laboratory, which are S. mediterranea, D. japonica, and D. sicula and it is based on their COI (cytochrome c oxidase subunit I) mitochondrial genes. We downloaded the nucleotide sequences of Cathepsin-D, DjFoxG, DjotxA, and DjPC2 genes already identified in D. japonica due to its phylogenetic proximity with our species. To align the selected sequences, we used ClustalX, which also included phylogenetic analysis in coordination with Njplot to confirm this phylogeny [14]. We examined the percentage of homology between Dug. japonica and Dug. sicula sequences through DNAMAN data [15]. The BLAST (Basic Local Alignment Search Tool) program, based on GeneBank, had also ensured alignment and analysis sequence similarities [16]. For the polymerase chain reaction (PCR), a silico simulation was carried out, and it is about OLIGO 7 [17]. The data obtained using the software also allowed us to design the primers.
2.3 Reverse transcriptase–PCR reaction
To start RNA extraction, we transferred five planarians from each specimen into 1.5-ml tubes. To lyse cells, we froze worms in liquid nitrogen for 5 to 10 s. After adding 400 μl of Trizol reagents and homogenizing worms, we appended 80 μl of chloroform. The mixture was centrifuged at 12,000 g for 15 min at 4 °C, then we collected the supernatant. For RNA precipitation, 200 μl of isopropanol were added and centrifuged for 10 min in the same conditions. We keep the pellet containing RNA, then we washed it with 1 ml of ethanol 75%. It was allowed to dry after centrifugation at 7500 g for 5 min at 4 °C, then dissolved in 20 μl of nuclease free water. Then, to synthesize cDNA, we added to the obtained RNA (2 μg) 1 μl of 50 μM oligo (dT)20 and nuclease-free water up to a total volume of 12 μl, then we incubated this synthesis reaction medium at 65 °C for 5 min. A mixture of reagents composed from 5x Reaction buffer, 10 mM dNTP Mix, Ribolock RNAase, an inhibitor (20 U/μl), a RevertAid H Minus M-Mulv and a Reverse Transcriptase (200 U/μl) in a total volume of 20 μl was added to the 12-μl synthesis reaction medium. It was incubated at 42 °C for 60 min, then at 70 °C for 5 min. After cDNA synthesis, a PCR reaction was prepared as described in Sandmann et al. (2011) [18]. The primers to amplify DjFoxG, DjPC2, DjOtxA and Cathepsin-D fragments were successively designed as displayed in Table 1.
Primers designed by the OLIGO 7 data for DjFoxG, DjPC2, DjotxA, and Cathepsin-D gene fragments.
Genes | Forward (5’-3’) | Reverse (5’-3’) |
DjFoxG | TTGATAATGATGGCAATTCG | TTAATTTGCCTGTTGTTCC |
DjPC2 | ACACTGGACAAGCTGATGGA | AACGCCCTCTACAATTGCAC |
DjotxA | GCAGTTGTTATGTAGCTGGCA | CGTGTTGATGCTGATGTCGA |
Cathepsin-D | GGCTTATCCGTCCATTTCAGT | AAGAAGAAAATTGCGCCCAG |
PCR cycling conditions have been adjusted and each one had different annealing temperatures as follows: the first step was denaturation at 94 °C for 3 min, followed by 40 cycles of denaturation at 94 °C for 30 s, annealing at different temperatures: 35 °C; 40 °C; 45 °C; 50 °C and 55 °C for 45 s, and elongation at 72 °C for 1 min with a final elongation at 72 °C for 10 min. The prepared reaction media were investigated by electrophoresis on a 1% agarose gel containing ethidium bromide. We needed between 30 to 50 ng of DNA in a total volume of 30 μl to achieve sequencing. The technological method was Sanger dideoxy sequencing.
2.4 In Situ Hybridization (ISH)
For in situ probe generation, we made a PCR amplification of template cDNA. Primers selected to get labeled riboprobes were the same as for RT-PCR, but were associated with Dig-labeled probes: DjFoxG forward: 5’-taatacgactcactatagggTTGATAATGATGGCAATTCG-3; DjFoxG reverse: 5’-atttaggtgacactatagTTAATTTGCCTGTTGTTCC-3’; DjPC2 forward: 5’-taatacgactcactatagggACACTGGACAAGCTGATGGA-3’; DjPC2 reverse: 5’-atttaggtgacactatagAACGCCCTCTACAATTGCAC-3’; DjotxA forward: 5′-atttaggtgacactatag GCAGTTGTTATGTAGCTGGCA; DjotxA reverse: 5′-taatacgactcactataggg CGTGTTGATGCTGATGTCGA; Cathepsin-D forward: 5’-aattaggtgacactatagAAGAAGAAAATTGCGCCCAG-3’; Cathepsin-D reverse: 5’-taatacgactcactatagggACACTGGACAAGCTGATGGA-3’. To synthesize RNA probes in vitro, we used T7 polymerase and Digoxigenin (Dig) labeling reagents. For Dig-labeling, we needed a total volume of 11 μl; we used 1 μg of purified template DNA, 2 μl of 10x NTP Dig, 4 μl of 5× transcription buffer for T7 (Kpnl), 1 μl of Protector RNase inhibitor (R1 Ribolock), and finally 2 μl of T7 polymerase. After mixing gently and centrifuging briefly, we incubated the solution for 2 h at 37 °C. Then we added 1 μl of DNase 1 and 1 μl DNAse buffer to remove the template DNA. This mixture was incubated for 15 min at 37 °C, and finally we stopped the reaction by adding 2 μl of 0.2 M EDTA. After purification by RNeasy Kit (Qiagen), we added 25 μl of nuclease-free water and measured the RNA concentration.
In situ hybridization (ISH) was performed as mentioned previously [19]. We selected intact (not regenerating) s1 and s2 D. sicula specimens; they were starved for one week before ISH inauguration. Briefly, in a first step, we used 2%HCL/5/8 Holtfreter, Canoy, MetOH, and 5%H2O2/MetOH as solutions to remove mucus, fix, and bleach planarians. Animals were selected (same length and size) in 2 ml of each solution. Hybridization is performed on the second day after rehydration by decreasing volumes of EtOH/5/8/PBS, preheat hybridization using a TPBS solution, protease digestion using 20 μg of proteinase K in 1 ml of TPBS, post-fixation by 5/8 PBS, 4%PFA/5/8 Holtfreter and TEA (0.1 M), acetylation by acetic anhydride and PBST, and prehybridization by prehybridization-mix (–20 °C) [20]. On day 3, posthybridization (washing) was carried out using decreasing volumes of formaldehyde (CH2O), then 2x SSC/0.1% Triton-X, Buffer I (malic acid buffer, Triton 10%, and H2O) and Buffer II (Buffer I, horse serum). For anti-body incubation, we added 1:2000 anti-DIG/Buffer II for 2 h, then washed planarians by Buffer I, keeping them overnight. On the last day, the color reaction take place in the dark, employing Buffer I, TMN, and 20 μl of NBT/BCIP ml TMN. To fix the color reaction, we used 1× PBS, 4%PFA/PBS, increasing then decreasing volumes of EtOH/PBS successively. Images of ISH samples were observed using a Leica M165 FC stereomicroscope.
3 Results
3.1 Bioinformatic analysis
The D. japonica genome is phylogenetically closer to D. sicula than S. mediterranea. The analyses were confirmed in silico by a ClustalX and Njplot software combination by drawing a phylogenetic tree (Annex 1). We downloaded DjFoxG, DjPC2, DjotxA and Cathepsin-D and genes nucleotide sequences of D. japonica to treat and deduce primers, by OLIGO7 data, among which we selected the best couple for each gene. The choice is fixed after performing several analyses using the same piece of software. The designed forward and reverse primers were stable and temperature conditions were optimal for all genes. We carried out the alignment (Fig. 1) of DjFoxG (Fig. 1a), DjPC2 (Fig. 1b), and Cathepsin-D (Fig. 1c) fragments, obtained from D. sicula, with selected partial sequences from D. japonica, by Clustalx and DNAMAN software combinations performance. We found similarity between the D. sicula and D. japonica selected sequences through the BLAST program too. The homology analyses achieved by the DNAMAN software for both species were close to those obtained by the BLAST program for both genes DjFoxG and DjPC2. Although there is a wide difference in Cathepsin-D genes homology results, the DNAMAN software shows about 53% between both species, whereas the BLAST program reveals 83% of homology. For the DjotxA gene, the PCR and RT-PCR reactions were not significant, so automatically it was not sequenced. The resulting PCR bands were sequenced and have been assigned under accession numbers in GenBank as displayed in Table 2. The accession numbers of their homologue sequences in D. japonica were mentioned in the same table.
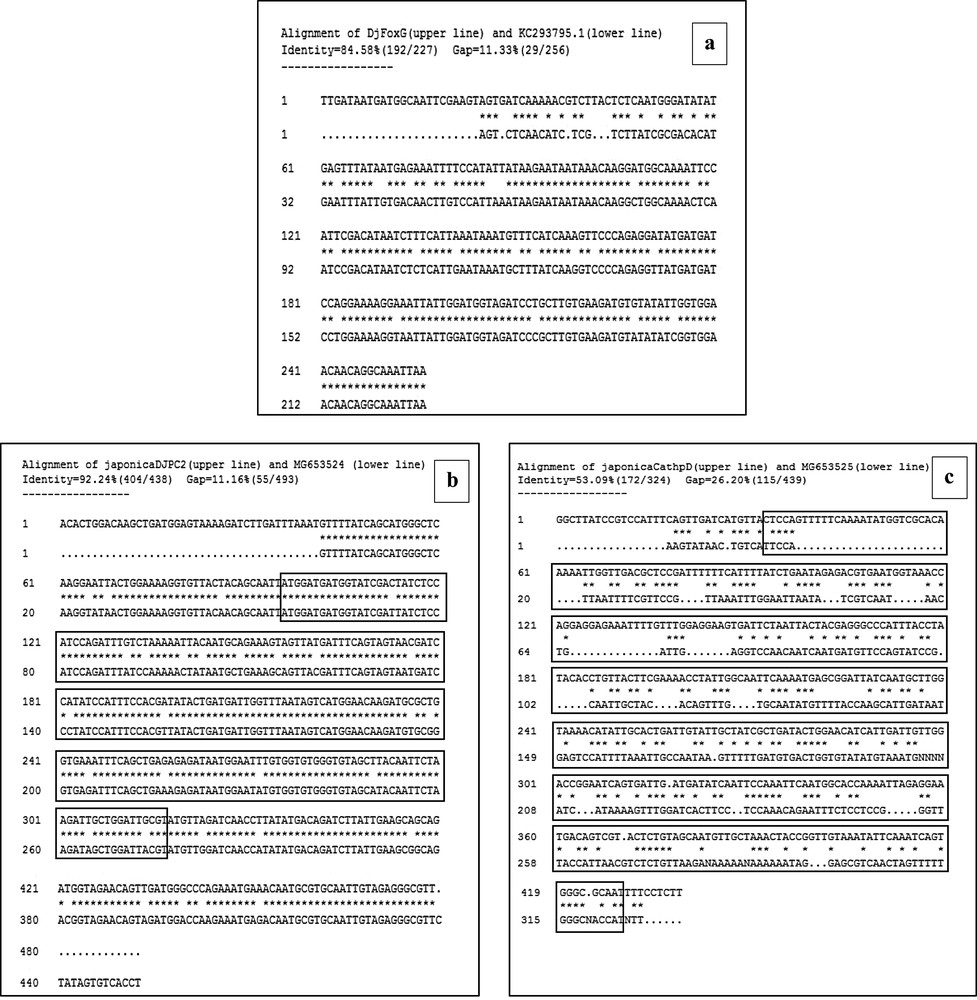
Alignment of sequences by the DNAMAN software in combination with clustalX, which also reveals the percentage of homology between Dugesia sicula and Dugesia japonica. The framed sequences correspond to fragments that we identified in D. sicula (upper line): DjFoxG (KC293795.1); DjPC2 (MG653524) and Cathepsin-D (MG653525). Alignment of DjFoxG amino acids fragments identified in D. sicula and already found in D. japonica (a). Alignment of DjPC2 fragments identified in Dug. sicula and downloaded sequence from GenBank corresponding to Dug. japonica (b). Alignment of Cathepsin-D sequences in these species (c).
GenBank accession numbers of the submitted Dugesia sicula nucleotide fragments and of their homologues already identified in Dugesia japonica.
GenBank accession numbers | ||
Nucleotide sequences | Dugesia sicula | Dugesia japonica |
DjFoxG | KC293795 | AB091061 |
DjPC2 | MG653524 | AK388877 |
Cathepsin-D | MG653525 | AK389015 |
3.2 Expression of the selected genes
The identified fragments were expressed by RT-PCR performance, except the DjotxA gene. The DjFoxG transcript had its highest expression under annealing at 35 °C, 40 °C and 45 °C, with a low expression at 50 °C and no expression at 55 °C. The DjPC2 transcript had high expression under all chosen annealing temperatures. The Cathepsin-D transcript had low expression, except under annealing at 45 °C. We note the absence of DjotxA bands in all revelations. Different annealing temperatures were adapted to select the best commune degree. For sequencing as for hybridization, the PCR cycling program was moderate, with a best annealing temperature (45 °C) for all performed genes. Electrophoresis analysis made on a 1% agarose gel of PCR reaction for sequencing reveals clear bands in expected size without nonspecific bands. We clean up the PCR product of DjFoxG, DjPC2, and Cathepsin-D with the Qiagen kit (Qiagen, Münster, Germany), then we measured their concentrations for sequencing; the final products have been treated to have an appropriate concentration of 50 ng. Even though there is no band for DjotxA, we tried anyway in situ hybridization. Contrary to what was expected in PCR amplification and sequencing results, ISH for DjFoxG gene provided a doubtful result for both specimens, despite the optimization conditions (Fig. 2), but significant results for DjotxA. Hybridizations for DjPC2 and Cathepsin-D were successfully revealed. In Fig. 3, the expression of DjPC2 in the brain was indicated by white arrows, whereas it was represented by green arrows in the pair of longitudinal nerve cords (VNC). Furthermore, the brain branches and cephalic ganglions were clearly stained, which explains that this neuropeptide plays an important role in this cerebral parts. These results prove the expression of DjPC2 in the central nervous system (CNS) of the D. sicula flatworm (Fig. 3). Otherwise, in situ hybridization results revealed that DjotxA is finally expressed (Fig. 4). For the s1 D. sicula specimen, DjotxA is clearly expressed in the visual cells, but is slightly stained in the brain (Fig. 4a) contrary to the s2 specimen, in which DjotxA was abundantly expressed on the cephalic ganglion (Fig. 4b). Cathepsin-D is expressed in the D. sicula gut (Fig. 5). Accurately, this ventral view shows a clear staining of the flatworm digestive system, specifically the anterior branch, posterior trunks and diverticulum of the gastrovascular cavity, except the pharynx and the mouth.
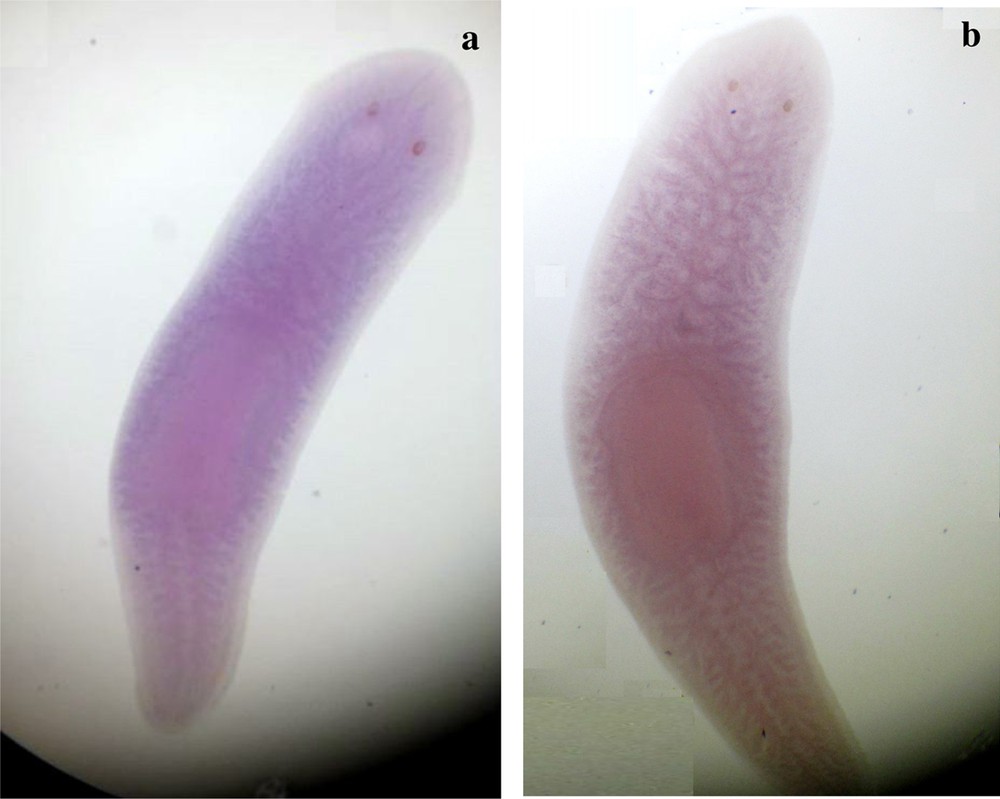
Ventral view of s1 and s2 specimens of Dugesia sicula showing the in situ hybridization of DjFoxG. s1 was a bit labeled but results could not be crucial (a). Results were not significant for the s2 D. sicula specimen (b).
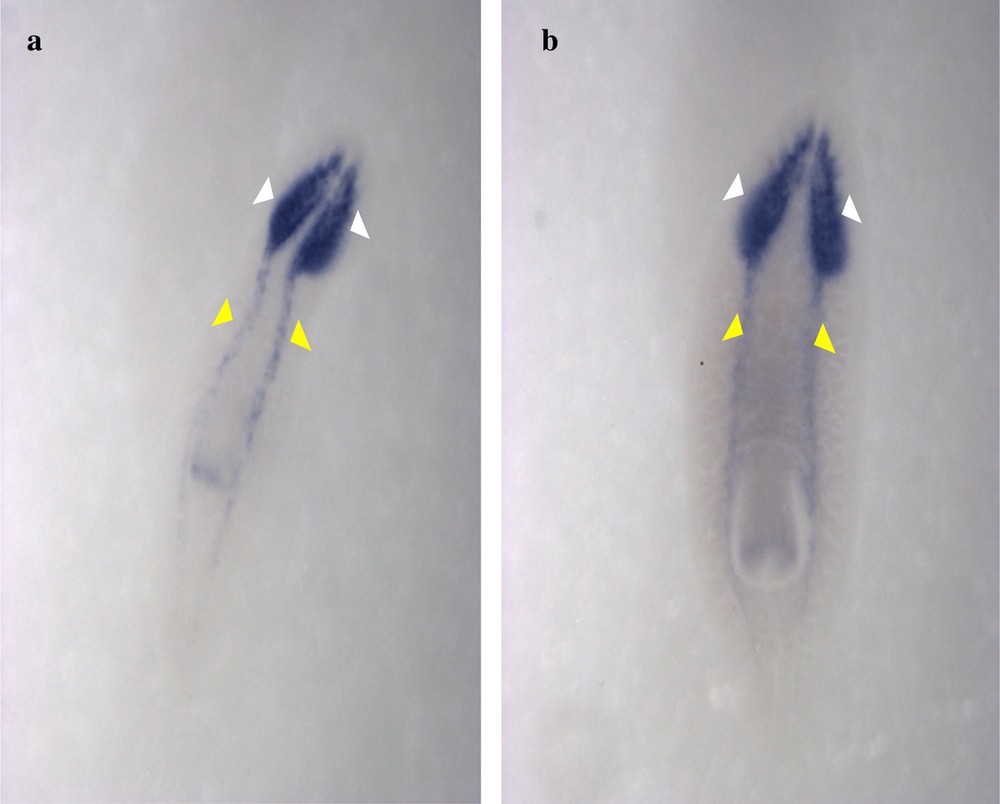
Expression patterns of DjPC2 genes in Dugesia sicula s1 (a) and s2 (b) specimens. Ventral view of the in situ hybridization performance (a and b). DjPC2 expression was detected on the central nervous system of D. sicula. It was expressed on the head (white arrows) and the ventral-dorsal nerve cords (yellow arrows).
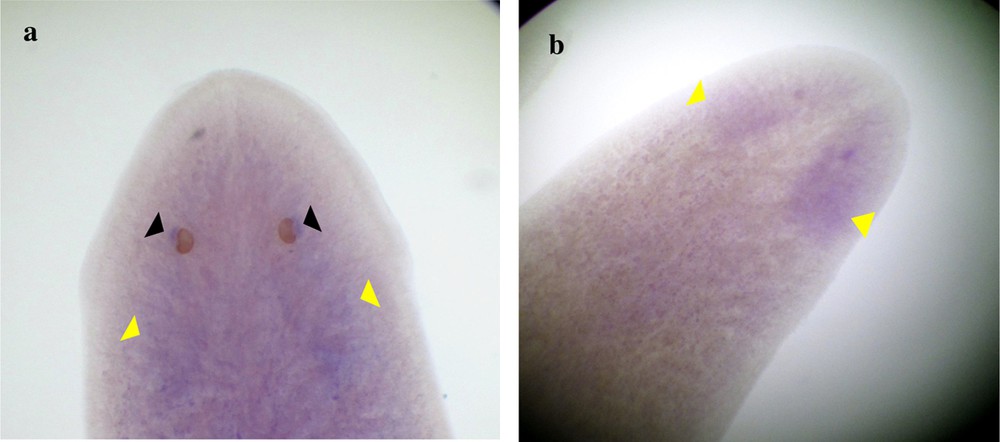
Expression of DjotxA in Dugesia sicula. Specimen s1 shows significant results, and the visual cells were clearly labeled (black arrows); we can almost discern expression in the brain (a). Expression of DjotxA in the head of specimen s2 (cephalic ganglion) (b).
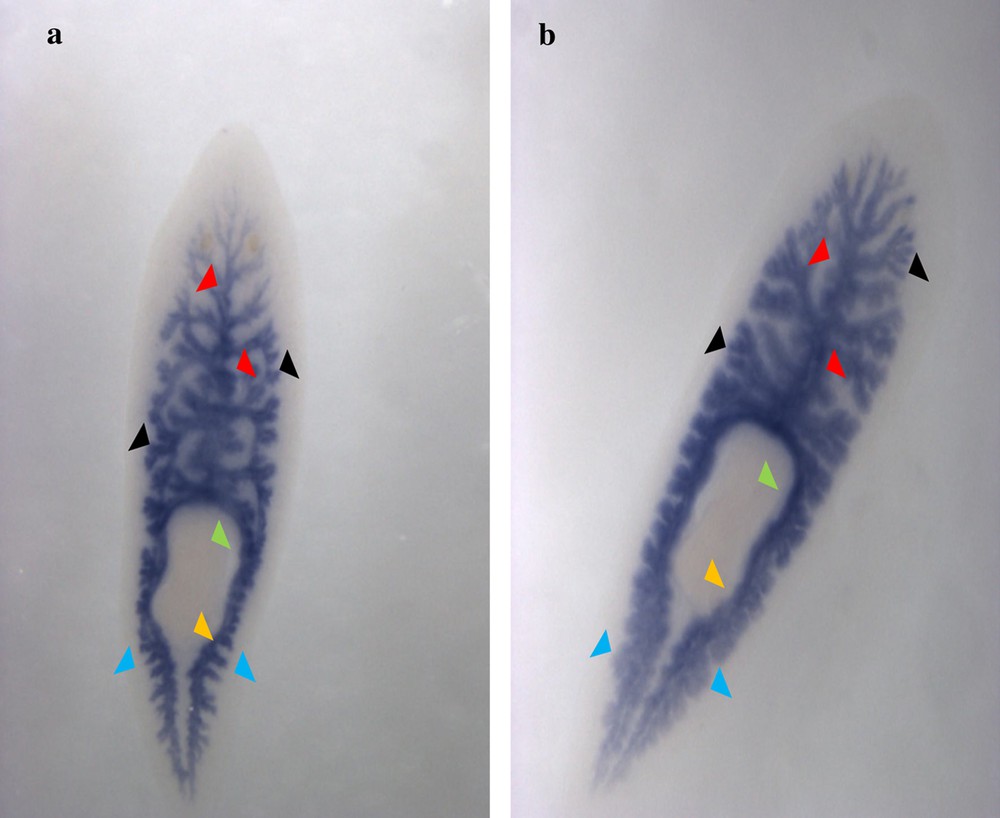
Ventral view of the in situ hybridization revelation of the Cathepsin-D gene in s1 (a) and s2 (b) Dugesia sicula specimens. Expression of Cathepsin-D on the planarian's gut (a and b). Staining of the gastrovascular cavity in the anterior (red arrows) and posterior (blue arrows) parts of the flatworm with its diverticulum. Absence of staining in the pharynx (green arrow) and the mouth (orange arrow).
4 Discussion
The OLIGO7 database performs several analyses, which are essential to achieve the nucleotide sequences amplification selectively for best PCR results [17]. The primers size is usually between 20 and 30 nucleotides in order to ensure a sufficiently specific hybridization to RNA and cDNA sequences. They have to show the best possible complementarities with both ends of the amplified sequence, so several criteria must be considered: for example, starting with ensuring the primers specificity by avoiding the formation of binding sites and having minimum mismatches with all the sequences. Secondly, avoiding the pairing primers between each other (Duplex), and forming dimmers and hairpin loops that reduce the concentration of primers, by resulting secondary sites of fixation [21]. It is necessary that primers hybridize to the template cDNA under the same temperature conditions. Their stability is determined by the free energy ΔG (kcal/mol), which must be less negative, to avoid the fixation of primers on each other, and also to avoid their binding sites, bases mismatches, duplexes and hairpin formations [22]. Actually, we choose to amplify partial fragments from selected genes in order to get rid of ambiguous and stretched bases that ensure favorite conditions for the polymerase chain reaction. ClustalX, ensuring a progressive alignment, and the DNAMAN software for multiple sequence alignment, have been used to confirm the species phylogeny rapprochement and to know the percentage of homology [16,23,24].
After bioinformatic analysis, expression was performed. Our RT-PCR results showed expression of all studied genes in D. sicula as predicted, except DjotxA, but these results confirm its genetic rapprochement to D. japonica. In situ hybridization for DjFoxG gene were blurred, so we cannot confirm, in a detailed way, the regions where it is normally expressed. Planarians were intact and this gene is a factor of transcription, highly expressed during regeneration [25]; this could be the reason of ISH expression deficiency. Recent studies confirmed that DjFoxG is a neural gene implicated in brain formation and that it is expressed in the cephalic ganglia in planarians [26]. DjFoxG had an expression in the body also, a strong one in the mesenchyme between epithelial layer and gut [28]. Its homolog FoxG1 is a forkhead gene specifically expressed in the telencephalon and retinal precursor cells in vertebrates [27] as mice [28]. It is expressed during otocyst development in zebrafish and mammals [29]. Then, for DjPC2 (prohormone convertase 2), as previously demonstrated, it is a neuropeptide processing enzyme and it is known as a major neurotransmitter [10]. According to current research, it was expressed in the CNS during planarian regeneration [19]. The identified DjPC2 in planarians has a high similarity with other animals [30]. It is known as a regulator in hypothalamus centers of the Siberian hamster [31]. Its homolog in mammals, which is the PC2 gene, is expressed in the neuroendocrine tissue and it is responsible for neuropeptide proteolytic processing [32]. This gene mediates prohormones and proneuropeptides proteolytic maturation [33]. Only hybridization results for DjotxA were different between D. sicula species s1 collected from Joumin and s2 from Boussadia. This gene is normally expressed in both, brain and visual cells [7], and in a previous study it was restricted to a medial region of ganglia where they found the projection of the photoreceptor axons [34]. The Cathepsin-D gene was expressed as expected in D. sicula's gut. It was known that aspartyl protease (Cathepsin-D) is localized in the digestive vacuolar compartments [35]. Previous research [36] confirms that a proteolytic cascade of this enzyme is used by the Schistosoma mansori parasitic worm to degrade hemoglobin into amino acids. Moreover, aspartyl protease has a blood-feeding function in the gut. This enzyme could have an important function in regeneration processing in planarians, while it was present during the cell differentiation stage in D. tigrina [37]. Interestingly, Cathepsin-D is synthesized from the midintestinal gland in response to viral infection [38]; so it contributes possibly in pathogens elimination [39].
In conclusion, these results confirm the current phylogenetic hypothesis and add prospects for initiating more appropriate studies concerning the species D. sicula. Bioinformatics pieces of software were fundamental to carry out molecular analysis as they facilitate the understanding of expression processing. The winged helix transcription factor DjFoxG was expressed through the RT-PCR technique, but unfortunately in this study the in situ hybridization (ISH) performance was low, but it could be definitively guaranteed in the future if we use D. sicula planarians that are in the process of regeneration. RT-PCR and ISH performances had shown a high expression of DjPC2 and Cathepsin-D. The DjotxA expression noticed in our work was exactly in the same region, compared with the work by Umesono et al. (1999) [7], which was realized on D. japonica. According to the ISH performance of the central nervous system, digestive systems, visual and stem cells, it is clear that D. sicula specimens collected from different dams are anatomically slightly different, but are close to the species D. japonica, too [36,40]. We target a set of different genes because we believe that studying their expression in D. sicula is a basic, but useful work to carry out more developed research on this species and understand the function of some body regions, under well-defined biological conditions. Planarians have a remarkable regeneration processing ability and D. sicula could be a good model to extend molecular performance and enrich appropriate studies.
Acknowledgments
This work has been supported, in part, by a fellowship grant to Emna Meddeb, by recurrent funding from the Tunisian Ministry of Higher Education and Scientific Research.
We thank the Stem Cells and Regeneration Group (Max Planck Institute for Molecular Biomedicine, Münster, Germany) and Professor Alessandra Salvetti (University of Pisa, Italy) for help and technical support.
Appendix 1 Phylogenetic tree of COI mitochondrial genes corresponding to Dugesia sicula (DQ666035), Dugesia japonica (DQ666034), and Schmidtea mediterranea (AF178322) using Clustalx and NJPLOT combinations.