1 Introduction
L-Tartaric acid is a polar, polyfunctional and chiral molecule with two asymmetric carbon atoms with the absolute configuration (2R,3R), and with a C2 axis of symmetry. The natural L-(+)-tartaric acid is one of the cheapest enantiomerically pure organic compound derived from salts present in different fruits and especially in grapes. Therefore, tartaric acid is an important by-product of the winemaking industry.
Within the framework of a research programme devoted to the development of non-food new applications of renewable raw materials, we have undertaken an exploratory study of new simple processes of transformation of L-(+)-tartaric acid. Tartaric acid is not directly obtained during the winemaking process, the obtained raw materials are tartrates. The actual natural compound, hydrogeno potassium tartrate (HPT), commonly called tartar or cream of tartar, precipitates during the fermentation process, where the formation of ethyl alcohol reduces its solubility. This salt is collected during the extraction and the mechanical descaling of the tanks to give from 100 to 350 g of HPT per hectolitre. The second important raw material, the potassium sodium tartrate, also called Seignette’s salt, is obtained by action of sodium hydroxide on the crude tartar and collection of these alkaline descaling solutions. Tartrates collected as HPT or Seignette’s salt are then transformed into pure tartaric acid via the formation of another salt: the calcium tartrate, by acidic treatment and crystallisation. The refining of tartrates into tartaric acid leads to an annual production of about 35 000 tons in the world.
Tartaric acid is mainly used industrially as additive in various fields: food industry (manufacture of sweets, drinks, cakes...), building industry (concrete and plaster), pharmaceutical and cosmetic industry (formulation of medicines, cosmetics...), and oenology (adjustment of the final acidity of wines). The market prizes for tartaric acid considerably decreased since 2000 from 7 to 2.5 €/kg, due to the international competition and the appearance on the market of enantiomerically pure synthetic tartaric acid from China. Therefore, it is really interesting to find some new applications for tartaric acid and its derivatives.
Tartaric acid played an important role in the field of stereochemistry from both historical and chemical-application points of view. Pasteur discovered the molecular asymmetry with the physical separation of the two optical isomers of sodium ammonium tartrate [1]. Within the large development of the asymmetric synthesis in the last decades, several applications of tartaric acid were reported, as optically pure building block (chiron) or as more or less derivatized ligands [2,3]. Simple derivatives of tartaric acid such as esters were used as chiral ligands, for example in asymmetric epoxidation [4], asymmetric Diels-Alder reactions [5], and asymmetric cyclopropanation [6]. On the other hand, more complex derivatives such as TADDOLs proved to be efficient ligands for enantioselective catalysis [7] as well as for complexing species in inclusion complex formation [8].
Our purpose was to develop new applications of tartaric acid using a few chemical transformations, cheap reagents and environmentally benign processes. Tartramides seemed to be interesting target compounds: they were not so much exploited [2], and a wide variety of simple or functionalized amines are commercially available. On the other hand, a direct condensation, as much as possible without using an activated form of the acid, should give a clean process with only water as by-product. Our goal was achieved using microwaves activation. A great deal of interest has been paid recently to microwaves technology in chemistry, to take profit of both its fast and homogeneous heating power and specific effects on a polarized substrate or medium [9,10,11].
2 Results
2.1 Synthesis of the tartramides
We reported recently a detailed account on the synthesis of tartramides under microwaves activation, using simple or functionalized amines [12]. This technology proved to be well adapted, as it was simultaneously observed by Loupy’s group on simple acids and amines [13]. The main features and results of our study, useful for the purpose of this paper, are summarised in the following schemes, tables and comments.
- • Using a monomode reactor equipped for power and temperature control of the reaction mixture [14], satisfactory conditions were determined (reaction between tartaric acid and benzylamine as model) to be: 40% excess of the primary amine (2.8 equiv.), no solvent, 12-min heating at 180 °C.
- • These conditions worked well for primary amines non substituted at C-1.
- • Conventional heating in an oil bath applied to the same mixture needed 16 h for 68% yield.
- • Water is the only by-product in these reactions.
- • The reaction works efficiently, with good yields, with primary amines but is very less efficient with hindered primary amines such as cyclohexylamine, as generally observed [15] and does not work with secondary amines (Table 1).
Synthesis of tartramides from aliphatic or aromatic primary amines under microwaves irradiation
Entry | R | Product (yield%) |
1 | CH2Ph | 1 (80) |
2 | C10H21 | 2 (82) |
3 | Cyclohexyl | 3 (30) |
4 | Phenyl | 4 (83) |
These reaction conditions were applied to functionalized primary amines (aminoalcohol, aminoethers, aminopyridine) in order to have access to multidentate derivatives. Good yields were obtained, except for N,N′-dimethylaminopropylamine, where only 15% of the corresponding diamide was isolated (Table 2). However, a high yielding synthesis of this compound (91%) was achieved by conventional condensation of the diamine with dimethyl L-tartrate.
Synthesis of tartramides from functionalized amines under microwaves irradiation
Entry | n | Y | Product (yield%) |
1 | 2 | OH | 5 (68) |
2 | 2 | OMe | 6 (65) |
3 | 3 | OMe | 7 (60) |
4 | 1 | 2-pyridyl | 8 (73) |
5 | 3 | NMe2 | 9 (15) |
A potential application of these new compounds is their use as complexing agent for metallic cations. We have started an exploratory study of the chelating properties of some synthesized tartramides towards Cu2+ as a model cation.
2.2 Complexing ability of functionalized tartramides with copper (II)
The complexing ability of three functionalized tartramides (with Y = OMe, NMe2 and 2-pyridyl) with copper (II) was investigated in aqueous medium at 25°C and ionic strength I = 0.1 adjusted with sodium nitrate.
For each ligand, solutions of variable ratio R = CL/CM (total ligand to total metal concentration) were neutralized by NaOH until pH 11.5. If complexation occurs, neutralization curves of metal-ligand solutions show a decrease in pH for a same volume of NaOH added and a displacement of the equivalent points, compared with those of ligands alone at the same concentration (Fig. 1). Qualitative analysis of the shape of the titration curves leads to the probable stoichiometry of the main complexes formed.
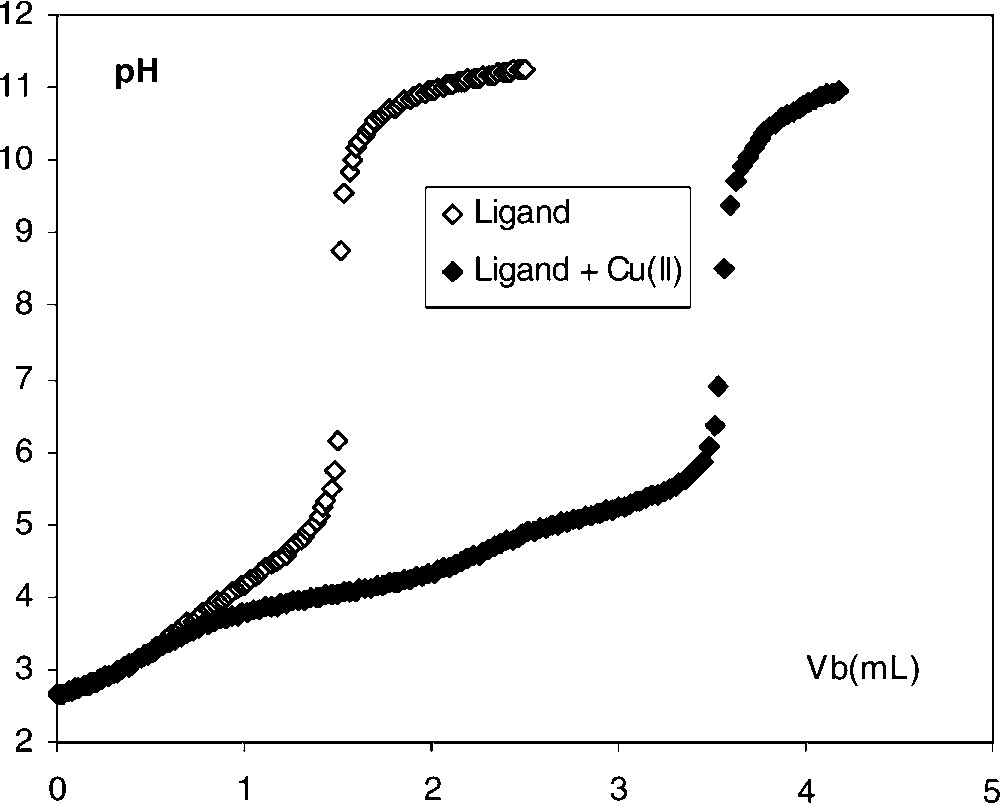
Neutralization curves of TAR–CH2-2-pyridyl in the absence and in the presence of copper (II). 25 ml of solution were titrated by NaOH 0.1 mol l–1; CL = 0.002 mol l–1; CM = 0.004 mol l–1; CHNO3 = 0.006 mol l–1.
As an example, we reported in Fig. 1 the titration curves of TAR–CH2-2-pyridyl alone and in the presence of copper (II) ions. The ratio R = CL/CM is equal to 0.5. For both curves, we observed an equivalent point around pH 8.5. The difference of the volumes of NaOH added between the two equivalent points corresponds to the neutralisation of 2 H+ per Cu(II) and the formation of the dinuclear species Cu2LH–4. In our experimental conditions, if a mononuclear species CuLH–2 was formed, the presence in the solution of an excess of free copper (II) would lead to the formation of a precipitate of Cu(OH)2 above pH 6.5. Similar titration curves were obtained for the two other ligands in the presence of copper (II). Consequently, we consider the formation of dinuclear species with the three tartramides studied.
Refinement of protometric curves using the software PROTAF [16] were then monitored to select a chemical model and to determine the global formation constants of the main soluble complexes. Structural investigation by ESR spectroscopy and mass ionisation electrospray are in progress in order to check the validity of our hypothesis. The complete study will be described in a forthcoming full paper. Our preliminary results will be used to compare the chelating ability of the three tartramides towards copper (II) with the ones of tartaric acid itself.
In Fig. 2, we plotted the molar fraction (on a logarithmic scale) of the free metal cations as a function of pH, for a given ligand/metal ratio, for the three tartramides and tartaric acid. Computation was done with the software HYSS 2 [17], using the stability constants and taking into account the hydroxo species CuOH+ and Cu2OH22+. The stability constants for copper (II)-tartaric acid were available in the compilation Critical Stability Constants [18]. Such a plot shows clearly the dramatic difference in stability between the complexes formed with the four ligands. In the case of tartaric acid, chelation occurs and is more efficient at lower pH than for two functionalized tartramides (Y = OMe, NMe2). An increase of pH leads to the opposite trend, indeed the fraction of free metal ions is lower at alkaline pH for two functionalized tartramides (Y = NMe2, 2-pyridyl) than for tartaric acid. This opposite trend is attributed to the formation of a chelate between the heteroatom of the substituent Y and the deprotonated nitrogen atom of the amidic moieties. The deprotonation of amidic groups occurs at neutral or alkaline pH. The chelating ability of functionalized tartramides decreases in the order:
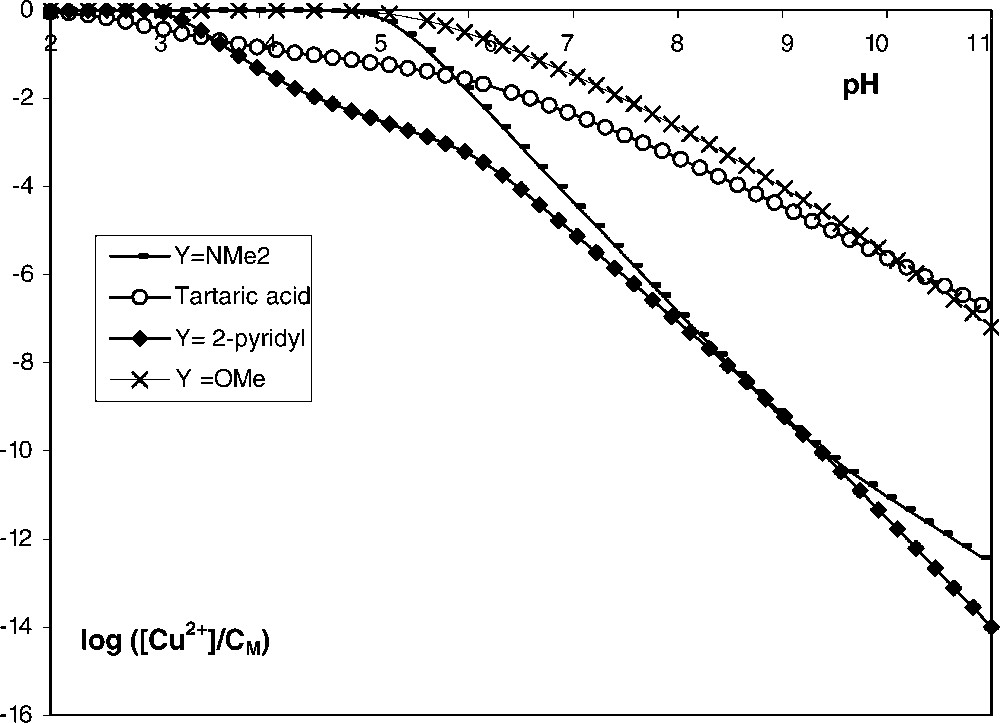
Plots of log([Cu2+]/CM) for Cu2+–ligand systems as a function of pH (CM = 2 × 10–3 mol l–1; CL = 2 × 10–3 mol l–1).
The better complexing ability of the pyridinium groups compared to N-dimethylamino ones is related to the ease of copper (II) ions to compete with protons bound on pyridinium groups, as well as to the formation of the five-membered chelated ring species.
3 Conclusion
The potentiality of some new functionalized tartramides as chelating agents towards metallic ions was investigated. Functionalized tartramides were synthesized from tartaric acid in one step under ‘green conditions’. Our preliminary results concerning the interactions between these ligands and copper (II) show that tartramides are able to bind strongly metal ions at neutral or alkaline media, especially. The chelating potentialities of the tartramides can be useful in applications that require selective complexation of metallic ions such as several cleaning-up processes of industrial effluents [19,20,21,22].
Acknowledgements
The authors thank the ‘Contrat de plan État–Région’ (Programme Glycoval) and the ‘Université de Reims–Champagne-Ardenne’ (Programme BQR) for their financial supports, and the ‘Fondation du site Paris–Reims’ for a postdoctoral fellowship (A.V.R.L.S., F.M.).