1 Introduction
Molecular self-assembly plays a fundamental role in biology, and is a widely used principle in the preparation of supramolecular structures and nanodevices [1]. In biology, the multiple cooperative non-covalent interactions (e.g., H-bonding, π-stacking, hydrophobic forces, electrostatics, etc.) between partners give rise to complex architectures, which span dimensions from a few nanometers to millimeters and provide the basis for the storage and relay of genetic information. With regards to the latter, nucleic acids can form various structures in solution and thus the specific H-bonding and π-stacking interactions are of fundamental importance. The formation of a boundary by the self-assembly of lipids is also a central feature of all living systems. Lipid bilayers act as boundaries and determine the compartmentalization of nucleic acids requested for life. Accordingly, nucleic acids and lipids biomolecules are of primary importance because they determine how living systems develop and function.
Currently there is a substantial interest in the design of hybrid molecules having a double functionality based on the combination of nucleic acids and lipid characteristics [2]. The design of assemblies that mimic molecular organization and compartmentalization observed in biological systems is of widespread interest for both fundamental aspects and applications. The use of nucleoside-based lipids has been mainly motivated by three fundamental objectives; (i) construct novel supramolecular materials an/or devices, (ii) develop new drug delivery systems and (iii) find new therapeutic molecules.
Since 2002, our group has been designing novel amphiphilic structures derived from nucleobases for multiple applications ranging from molecular building blocks for self-assemblies, to drug or biomolecule delivery systems.
The aim of this article is to highlight some of the recent advances in the area of amphiphile structures derived from nucleosides and nucleotides. We wish to underline that these molecules offer new perspectives in the fields of soft materials and biotechnologies. First, we will focus on the previous works realized on supramolecular systems constructed with nucleoside-based lipids. This section includes several examples of zwitterionic, neutral, anionic and cationic nucleoside-based amphiphiles synthesized in our laboratory. Second, we present several nucleoside-based amphiphiles currently developed to transport nucleic acids into cells.
2 Supramolecular assemblies
A great challenge of our time in chemistry is to construct supramolecular systems. Examples of supramolecular assemblies that highlight the underlying principles are evident in numerous biological (e.g., lipids) and synthetic (e.g., nano-fibers) systems. In the 90s different groups demonstrated that the association of lipid moieties with nucleoside and/or nucleotide was an efficient strategy to obtain new supramolecular objects and behaviors [3–5]. As a novel type of bio-inspired structures different investigations on these nucleoside base-lipids were realized. For example, dioleylphosphatidyl derivatives bearing a nucleoside moiety were synthesized and their surface behavior was studied [6]. Interestingly, it was found that the interfacial orientation of nucleolipids provides a suitable environment for base–base pairing. In several other studies involving micelles or vesicles, base–base interactions through stacking and H-bonding were identified at the object surface [7]. This topic was extensively reviewed by Berti and Baglioni [8]. Recently, a new family of non-covalent nucleoside amphiphiles were developed where the nucleotides and the amphiphiles are complexed simply by electrostatic interactions [9]. Similarly as covalent derivatives, different types of aggregates including left handed helix or bilayers were observed in solution confirming the remarkable supramolecular capabilities of nucleoside-based lipids.
Our contribution to this research area has focused on the design of novel molecular structures for new supramolecular systems and biomedical applications. A thorough consideration of the possible molecular structures was a critical component for the effective design and development of these types of supramolecular building blocks. Toward this end, we chose to modify the molecular structure of conventional lipids and amphiphiles via the incorporation of biomolecules. We proposed new amphiphiles possessing both a nucleobase and a hydrophobic segment (Fig. 1). The impact of molecular structure variations on the physio-chemical and self-assembling properties of the nucleoside-based lipids was investigated by using different techniques. Hence, we synthesized different families of nucleoside and nucleotide-based structures. Most of these molecules, which posses both the molecular recognition features present in DNA or RNA and the aggregation properties of lipids, have shown remarkable physico-chemical properties. Interestingly, the nucleoside-based amphiphiles were found to self-organize into several supramolecular structures such as vesicles, fibers, hydrogels, organogels or hollow spheres. In this section, we present; (1) uridine and adenine phosphocholine derivatives, (2) the nanostructured assemblies formed by thymidine based lipids, (3) the 2D-supramolecular structures on graphite by self-assembly of physisorbed amphiphile nucleotides and (4) the spontaneous formation of hollow microspheres constructed from f-block elements and nucleoside-based amphiphiles.

Modification of a nucleoside (left). Conceptual view showing our approach (right). In order to take advantage of forces that hold nucleic acid helices together, (Watson–Crick/Hoogsteen hydrogen bonding and base stacking) we have proposed new amphiphiles possessing a nucleoside and hydrophobic chains.
2.1 Nucleoside phosphocholine derivatives
One of the biggest challenges for bio-inspired amphiphiles is finding easy ways to take advantages of their building blocks to construct complex supramolecular systems. To achieve this objective, a clear understanding of the chemical structural parameters that dictate self-assembly is of high importance. Our general approach was to modify the molecular structures of conventional lipids such as fatty acids via the incorporation of either a nucleoside or a nucleotide. A first family of zwitterionic uridine phosphocholine amphiphiles was initially synthesized as shown in Scheme 1 [10]. This four-step synthetic strategy was developed to afford the phosphocholine uridine amphiphiles 5a through 5e. As expected, the uridine derivatives synthesized provided an easy access to supramolecular assemblies in aqueous and non-aqueous media. Interestingly, by changing the temperature, the nucleoside amphiphiles form fibers or bilayers in aqueous solvent. This transformation between the two supramolecular structures, which depends on the melting temperature (Tm) of the amphiphiles, was found to be fully reversible (Fig. 2). The hydrogels stabilized by the nano-fibers can be prepared in the presence of plasmid DNA, suggesting that the system could be used as functional delivery matrix.
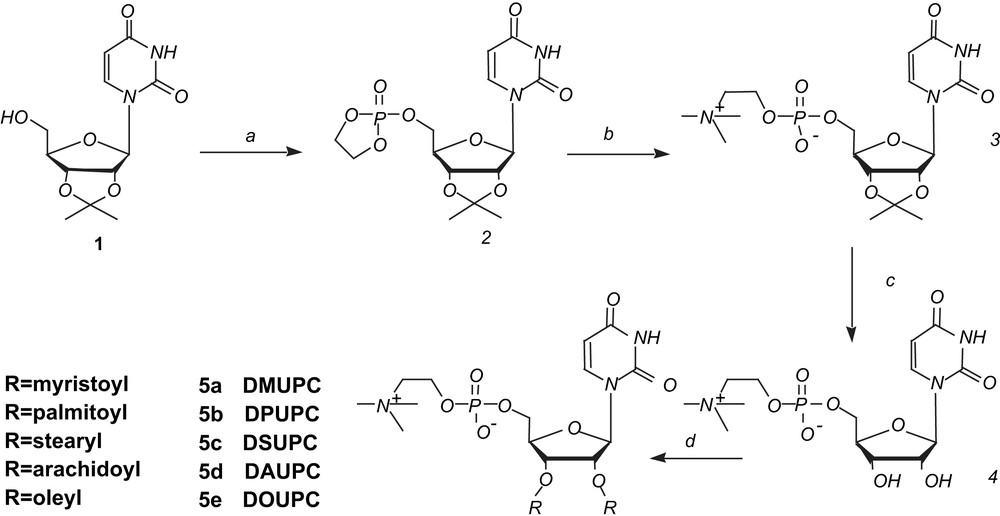
Synthesis of uridine based amphiphiles (a) chloro-oxo-dioxaphospholane, TEA, THF, 0 °C, 15 h; (b) trimethylamine, AcCN, THF, 60 °C, 24 h; (c) HCl in HCO2H/H2O; (d) myristic/palmitic/stearic/arachidic/oleic acids, DCC, DMAP, DMF, RT.

The thermoreversible helical–bilayer transition observed in aqueous media.
An adenosine phosphocholine derivative with fatty esters on the 2′ and 3′ positions was also prepared by using a convenient synthetic route involving unprotected intermediates [11]. Similarly as for uridine amphiphiles, the adenine moiety does not prohibit the formation of lamellar organizations above the Tm and stable small unilamellar vesicles were prepared by using extrusion techniques.
The behavior of the perfluorocarbon analogues was explored more recently. In the context of fluorinated molecules, it was straightforward to achieve the substitution of the 2′ and 3′ nucleoside ribose positions by fluorocarbon chains. Consequently, the 2H,2H,3H,3H-perfluoroundecanoic acid was DCC coupled to the 2′ and 3′ secondary hydroxyls of 5′-phosphocholine uridine 4 (Scheme 1) to afford the fluorinated nucleoside-based amphiphile (2′,3′-O-di-2H,2H,3H,3H-perfluoro-undecanoyl-uridine-5′-phosphocholine, DiF17UPC) shown in Fig. 3 [12]. Surprisingly, despite the high hydrophobic character of the amphiphile, lamellar systems were observed (Fig. 3). Indeed, hydrocarbon analogues possessing saturated alkyl chains such as DSUPC (1,2-Distearoyluridinophosphocholine) self-assemble into helical structures stabilizing nano-fibers in the crystalline state below its Tm. The lamellar systems, observed in the case of the fluorinated derivative, were explained by the greater stiffness and volume of the fluorocarbon chains, which avoid the chain–chain packing requested for the formation of the nano-fibers.

TEM image of lamellar systems obtained after sonication of DiF17UPC (bar 250 μm). The chemical structure of DiF17UPC is shown in the insert.
2.2 Nucleotide-based lipids
There are several ways of designing novel bio-inspired amphiphiles featuring nucleobases. One of the simplest ways consists of using a nucleotide instead of a nucleoside. A variety of synthetic strategies have been explored to synthesize nucleotide-based amphiphiles [13], The large literature dedicated to this class of amphiphiles reports mainly hydrophobic modification of nucleoside-5′-monophosphate derivatives. Another strategy was to develop a synthetic route to 3′-O-alkyl nucleotide. The rationale behind this synthetic approach was to use the 3′-phosphoramidites 5′-protected nucleosides commercially available as starting materials for the preparation of the nucleotide amphiphiles. Accordingly, single chain nucleoside-3′-monophosphate amphiphiles were synthesized as shown in Fig. 4. Briefly stated, starting material 6 was reacted with different fatty alcohols in the presence of tetrazole, the resulting lipidic intermediates were oxidized with iodine in a THF/pyridine/water mixture at room temperature. Removal of the cyanoethyl and dimethoxytrityl protecting groups led to a series of nucleotide-based amphiphiles 8. It was discovered that the aqueous dispersion of these nucleotide amphiphiles could induce the formation of nano-objects. The collected information that emerged from numerous investigations (TEM, SEM, FT-IR and WAXS) of these nucleotide amphiphiles demonstrated that these nucleotide amphiphiles possess different aggregating properties compared to both their non-nucleotide analogues and simple nucleotides. Nano-structured assemblies such as ribbon-like structures for the thymidine derivatives (Fig. 5) and small aggregates for adenine amphiphiles were observed, indicating that the nature of the base has a direct impact on the aggregates morphology.

Synthesis of nucleotides based amphiphiles. (a) CH3(CH2)nCH2OH; tetrazole 0.45 m in MeCN; THF; RT; N2; 19 h. (b) I2 0.02 m in THF/Pyr/H2O 70:20:10; RT; N2; 24 h. (c) NH4OH 30% in water; 60 °C; 24 h. (d) CCl3COOH 3% in DCM; RT; 2 h.

Transmission electronic microscopy (TEM) image of C20-3′TMP (8c) from a sample dispersed in deionized water (1 mg/mL). Negative staining ammonium molybdate 1% in water.
2.3 2D-supramolecular assemblies on graphite surface
Beside nano-structured assemblies in colloidal suspensions, the preparation of functional surfaces with nanometer dimensions is also currently attracting a substantial interest from both a fundamental and an applied point of view [14]. In many cases the functionalization of a surface is required to take advantage of the properties of a given material. For example, to make nanotubes more easily dispersible in liquids, it is necessary to physically or chemically attach certain molecules, or functional groups, to their smooth sidewalls. This process, which is called functionalization, has to be achieved for biological applications especially for sensing [15]. In this context, nucleoside amphiphiphiles are ideal candidates for surface nanopatterning and/or functionalization.
Since the assembly processes of amphiphilic molecules on surfaces and interfaces can be imaged at the nanometer scale by using scanning probe microscopies, The behavior of nucleotide amphiphiphiles at the liquid/solid interface was investigated in collaboration with Dr. Fichou's group (CEA Saclay, France). Interestingly, two-dimensional supramolecular organizations on graphite were successfully imaged by high resolution Scanning Tunnelling Microscopy (STM) by self-assembly of physisorbed amphiphile nucleotides [16]. Here again, it was found that the organization of the molecules was dictated by the nature of nucleobases. In the case of the thymidine amphiphiles a head-to-tail self-assembly was imaged (Fig. 6C), whereas a head-to-head organization was observed for adenosine derivatives (Fig. 6A). Surprisingly, the co-adsorption of complementary mixtures allowed the formation of a two-dimensional supramolecular structure likely stabilized via base pairing (Fig. 6E). Several examples of 2D structures observed on the graphite surface and their corresponding calculated models are shown in Fig. 6.

Typical STM images of different physisorbed nucleotide-based amphiphiles (A, B and C) and their corresponding 3D supramolecular assemblies (molecular calculations, B, C and D. Yellow and blue marks show the H-bonds between neighbouring molecules and base–base stacking, respectively). (A) STM image of 8a (C20-3′AMP) on graphite (image width is 30 nm), the adenosine self-assemblies show a head-to-head arrangement of the molecules where the bases are stacked to the surface (B). (C) STM image of 8c (C20-3′TMP) on graphite (image width is 71 nm), the thymidine self-assemblies show a head-to-tail arrangement of the molecules where the chains are interdigitated and the nucleobases are desorbed from the surface (D). (E) STM image of an A + T mixture (1:1, 8a + 8c, image width is 12.6 nm). (F) Computer generated model revealing the association between A and T through H-bonds into a head-to-head fashion [16].
2.4 Hollow spheres
An important extension of the nucleoside-based lipids involves the formation of supramolecular assemblies composed of f-block elements. These objects, in particular hollow spheres, could be of interest for many applications in several fields including photonics, catalysis, energy storage, and medicine [17]. In order to construct the microspheres, we took advantages of the unique self-organization capabilities of nucleoside-based lipids. Accordingly, microspheres featuring a hybrid lipid-cation multilamellar shell were prepared simply by hydration of nucleoside-based lipids with aqueous solutions containing actinide or lanthanide salts. It is worthy to note that due to the lack of nucleobase natural phosphocholine lipids cannot induce the formation of microsphere. It was demonstrated that the microsphere is stabilized thanks to both hydrophobic and base–base interactions. In addition, phosphate/f-block element salt binding allows the formation of the hollow supramolecular structures shown in Fig. 7.

a) Microspheres are prepared by hydration of a nucleoside-based amphiphile with an aqueous solution containing either actinide or lanthanide salts. These supramolecular structures possess a hybrid lipid-cation shell where the cations are entrapped into the multilamellar systems. (b) Fluorocarbon derivative such as DiF17UPC can be used to prepared hollow microspheres. (c) Example of microspheres obtained in the presence of DSUPC.
3 Nucleoside-based lipids for transfection
As mentioned previously, the conjugation of molecular recognition principles (e.g., nucleoside, nucleotides, oligonucleotides, etc.) to lipid features provides an efficient and powerful means to prepare supramolecular systems with tunable physico-chemical properties. One of the basic ideas behind these complex systems is to create new vehicles for the delivery of bioactive principles. Conceptually, the base pairing capabilities render nucleoside-based lipids ideal candidates for the transport of oligonucleotides, DNA, RNA, and siRNA. A question worth asking at this stage is: Can we take advantage of the base–base interactions between nucleic acids and nucleoside to entrap the biomolecules and stabilize DNA–lipid systems?
In this section we will describe nucleoside-based lipids systems currently used to transport nucleic acids into cells using a synthetic-DNA supramolecular assembly.
3.1 Cationic species
A feature of supramolecular systems is hierarchy, where primary building blocks associate into more complex secondary structures that are integrated into the next size level. These hierarchical constructions may provide useful systems for drug delivery applications. In particular, when bioactive molecules such as oligonucleotides are inserted in the hierarchical self-assemblies constructed with building blocks such as lipids or amphiphiles. Such combinations can lead to effective drug vehicles capable to transport and deliver polynucleotides. In the framework of biomedical research, the delivery of nucleic acids to cells and the resulting ability to correct a defective gene, introduce a new gene, or knock-down a gene provides a method to potentially treat many diseases. Cationic-based synthetic nonviral systems are routinely used in vitro as vehicles for nucleic acids.
Numerous cationic lipids have been developed and evaluated as cationic agents for plasmid DNA delivery [18]. Different structural features have been modified on the basic cationic lipid structures to enhance the transfection efficiency. Importantly, most of the synthetic vectors currently in use rely either on electrostatic forces or electrostatic and hydrophobic interactions for DNA binding and gene delivery. Our group has pioneered the use of nucleoside-based lipids with additional hydrogen bonding and ð stacking capabilities to favor the interactions between nucleic acids and the synthetic vector [19]. Several promising nucleic acid vectors based on a cationic amphiphilic uridine were synthetized (Fig. 8). The in vitro transfection assays realized with these derivatives were very encouraging. For example, O-Et-DOUPC at high nucleolipid:DNA (w:w) ratios (18:1–36:1) was an efficient transfecting reagent in CHO cells using a reporter β-gal gene assay. These results compare favorably with DOTAP and Transfast both used under optimal conditions (Fig. 9). In contrast to conventional cationic transfecting reagents, the cytotoxicity of nucleoside-based lipids such as O-Et-DOUPC or DOTAU (Fig. 8) was shown to be negligible. Hence, nucleoside-based lipids can be used in a wider range of concentration compared to Lipofectamine and for cell lines sensitive to the toxicity of conventional cationic lipids.

Structures of uridine lipids used for transfection.
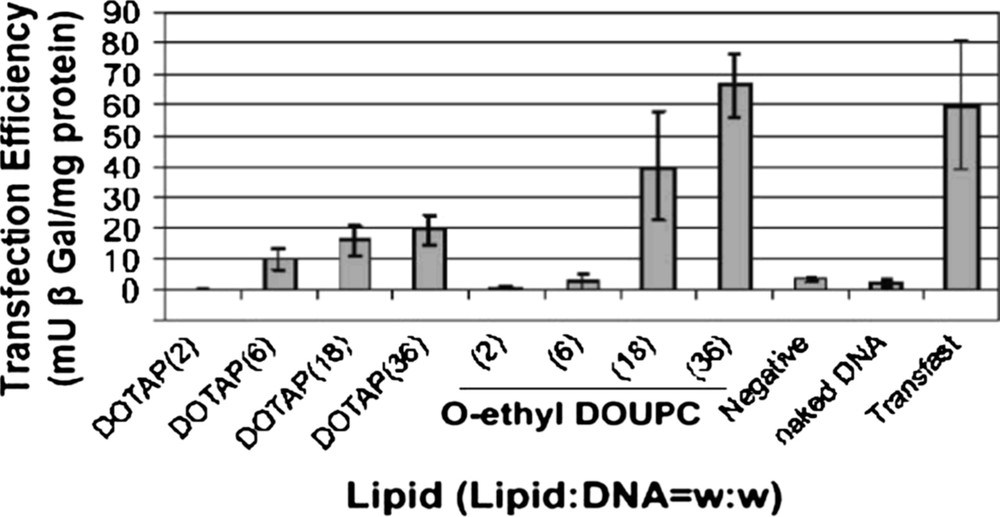
Transfection efficiencies of O-Et-DOUPC compared to two commercial transfection reagents (DOTAP and Transfast) [19].
3.2 Nucleoside-based lipids–DNA complexes
The supramolecular systems involving calf thymus DNA (CT-DNA) and nucleoside-based lipids feature particular characteristics. Data collected from several experiments, including SAXS (small angle X ray scattering) show that CT-DNA/nucleoside-based lipid complexes arrange into LαC phases (lamellar complexes, with DNA intercalated between planar lipid membranes) [19,20]. Notably, In the case of DOTAU, it was found that the DOTAU/poly A lipoplexes were more compact systems than DOTAP/poly A lipoplexes due to tighter association between the uracil base of DOTAU and adenine of the poly A single strand.
While most of the lipoplexes rely only on electrostatic interaction between the polyanionic DNA and cationic lipids, DNA complexes were also observed in the presence of neutral nucleoside-based lipids. For example, the nucleic acid binding properties of the glycosyl nucleoside derivatives (Fig. 10) were investigated by different techniques including quasi-elastic light scattering (QELS), UV–vis, transmission electronic microscopy (TEM), NMR, IR, and circular dichroism (CD) [21]. It was found that lipid–nucleic acid complexes are stabilized thanks to the amphiphilic character of the glycosyl derivatives and phosphate–sugar interactions. Note that the uridine moiety of the lipid contributes to the stabilization of the lipid/polyA–polyU complex.
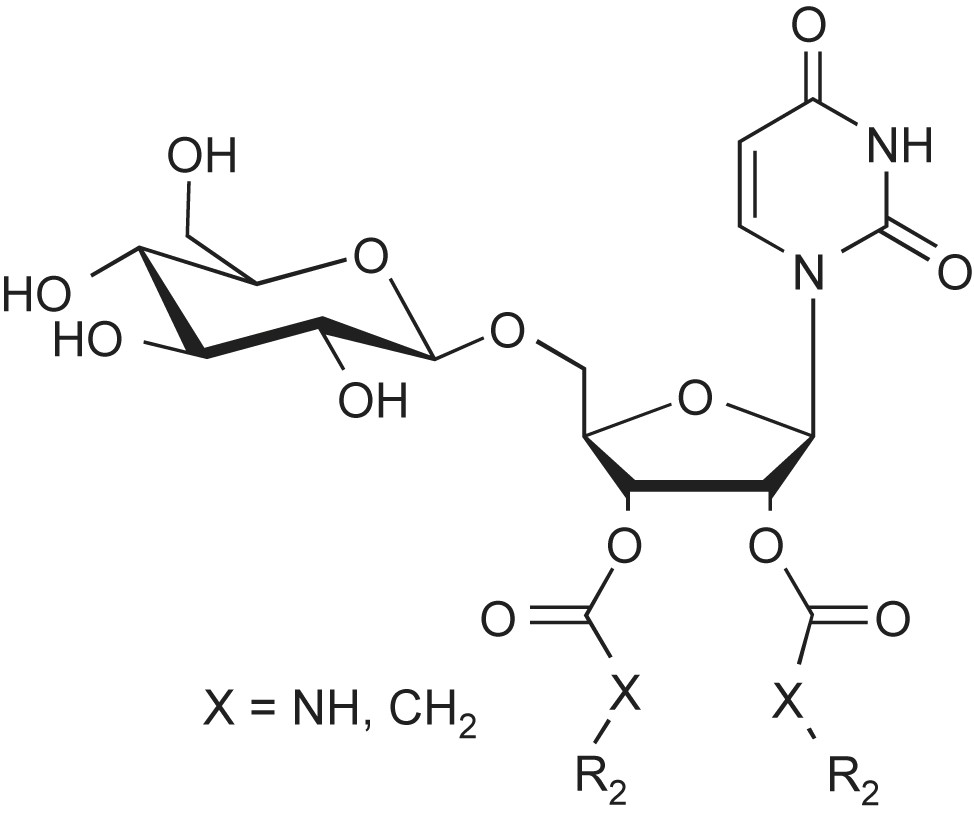
Example of a glycosyl nucleoside derivative (R2 = alkyl chains).
Interestingly, lipoplexes only stabilized via base pairing have been reported recently [22]. Baglioni and co-workers demonstrated the proof of the concept of “nucleolipoplexes” by exposing polynucleotides to anionic nucleolipids. It is worthy to note that these supramolecular systems were the results of complementary molecular recognition between micellar systems made of short chains nucleolipids and complementary polynucleotides. It will be interesting to watch the field of nucleolipoplexes in the years ahead to see if these systems are able to transfect polynucleotide.
4 Conclusions
It is an exciting time for the field of nucleoside-based lipids, which is clearly an integral and inseparable component of nano-sciences and biomedical technology. The emerging devices developed so far clearly open up interesting avenues for the design and development of supramolecular systems, biotechnological tools and therapeutic strategies. The possibility of invention and innovation using these amazing molecules seems endless and only limited by imagination and creativity. Hopefully this short look at the subject will convince the reader that the marriage of nucleic acids with lipids can lead to complex molecular structures to construct and develop soft and complex matters.
Acknowledgements
P.B. acknowledges financial support from the Army Research Office and ARC. Particular thanks are due to Dr. Mark W. Grinstaff (Boston University, USA) for many collaborations and discussions, to Dr. Michel Camplo (Faculté des Sciences de Luminy, Marseille, France) and Dr. Denis Fichou (CEA, Saclay) for their participation in physico-chemical and biological studies of these amphiphiles.