1 Introduction
Imidazolinone herbicides (imazapyr, imazapic, imazethapyr, imazamox, imazamethabenz, acid or methyl, and imazaquin) present low toxicity for humans, being an alternative to phenoxy herbicides, such as 2,4-D and 2,4,5-T, or to triazine herbicides, especially those of the atrazine family.
Pesticides and their metabolites are usual contaminants of groundwater [1, and references cited therein] but imidazolinone herbicides, such as those here investigated, have low toxicity for humans. The reductions in a wide pH range of imazapyr, imazethapyr, imazaquin, imazamethabenz methyl and imazamethabenz acid have been studied by polarography and voltammnetry [2–4]. For the herbicides other than imazamethabenz, two sets of pH-dependent signals were found at pH < 7, which were assigned to the reduction of the two reducible parts of the molecule, i.e., the pyridinic (or quinolinic) and the imidazolinone rings (Scheme 1). The results confirmed that the set of signals appearing at more negative potentials are related to the pyridinic/quinolinic ring reduction, being the assignation of the peaks appearing at less negative potentials more difficult.

Structures of Herbicides. Left: R = H–imazapyr (IMZP), R = CH3–imazapic (IMZC), R = CH3–CH2–imazetapyr (IMZE), R = CH3CO–imazamox (IMZM). Center: imazaquin (IMZQ). Rigth: R1 = H−, R2 = CH3–imazamethabenz methyl (IMBM). R1 = CH3−, R2 = H imazamethabenz acid (IMBA).
The knowledge of the protonation state of the different species in solution is essential in both the soil sorption in the natural environment and the elucidation of the reduction mechanisms. From ultraviolet-visible absorption spectra of Imazamethabenz-methyl, IMBM, the pK values 3.4 ± 0.2 and 9.4 ± 0.2 were obtained for protonation of the imidazol (= N–) nitrogen and dissociation of the NH imidazol nitrogen of IMBM, respectively [5]. For Imazamethabenz-acid, IMBA, the dissociation pK of the carboxylic group is very close to that of the imidazol (= N–) nitrogen, both being close to 3.3. The dissociation pK of the NH imidazol nitrogen for IMBA is 9.6 ± 0.2.
In the literature were found three pK values for the imidazolinone herbicides [6–13] but the structures of the molecules contain an additional acid-base center with respect to IMBA, namely the pyridinic or quinolinic nitrogen, as can be seen in Scheme 1. So, one of the abovementioned pK values must correspond to a double protonation equilibrium.
On the other hand, the electroreduction of such compounds in strongly acidic media has not been examined in deep.
So, the aim of this paper was, first, the identification of the species present in the solution as a function of the pH, in relation with the soil sorption, and second, to present the reduction mechanisms in very acidic solutions.
2 Experimental
In all cases, Merck analytical grade reagents were used with the exception of herbicides which were from Riedel de Haën (analytical standard); IMBA was prepared by alkaline hydrolysis of IMBM as follows [14]: 10 mL of 2 M NaOH solution were added to 500 mg of IMBM and the suspension was stirred at room temperature until clear (about 3 h). The solution was washed with chloroform, and concentrated HCl was added until precipitation of the acid that was filtered and re-crystallized from ethanol. All other reactants were used without further purification. The working concentration of reactant was 1 × 10−4 M, for polarographic measurements and 1 × 10−3 M, for voltammetric measurements, with the evident exception of the experiments in which the influence of this variable was studied. Solutions of either sulfuric acid, for pH < 2, 0.05 M in both acetic and phosphoric acids for 2 < pH < 8 or 0.05 M in both sodium carbonate and phosphoric acid for pH > 8 were used as supporting electrolytes. Stock solutions of imazamethabenz methyl were stored at 4 °C to avoid decomposition. The ionic strength was adjusted to 0.3 M with NaCl. The pH was adjusted with solid NaOH. All potentials were measured against the Ag/AgCl/KClsat electrode. Solutions were purged with purified nitrogen and the temperature was kept at 25 ± 0.1 °C. The aqueous solutions were prepared using ultrapure water type I (resistivity 18.2 MΩ.cm at 25 °C) obtained by an ultrapure water system Millipore Simplicity®.
Spectrophotometric studies were performed with the following buffers: solutions of perchloric acid at pH < 1.5, 0.1 M phosphoric acid + 0.1 M acetic acid for 1.5 < pH < 8 and 0.1 M sodium bicarbonate + 0.1 M phosphoric acid for pH > 8.5. UV–vis absorption spectra (190–390 nm) were recorded with a double beam Varian Cary 100BIO spectrophotometer. Hanna quartz cuvettes of 1 cm pathlength were used.
The mercury working electrode was a BAS MF-9024 controlled growth electrode, with an area of 6.70 × 10−3 cm2. The glassy carbon electrode was from IJCambria (geometrical area = 38.5 mm2). Triply distilled mercury was used.
3 Results and discussion
3.1 Distribution of species as a function of the pH in relation with the soil sorption
Structures of the imidazolinone herbicides imazapyr, imazapic, imazethapyr and imazamox have a pyridine ring instead the benzene ring of the IMBA structure. For imazaquin, the ring is a quinoline. In any case, the pyridine or quinoline nitrogen has basic character and is easily protonated. The protonation pK in nicotinic acid is 4.8 (where the dissociation pK of the carboxylic group is 2.1) [15,16]; this pK is 3.7 for nicotinamide [17], 3.5 for N’-methylnicotinamide [18] and 3.2 for niazid [19]. In all these cases there is only a substituent in position 3 with respect the pyridinic nitrogen. For diflufenzopyr the pK value is 3.2 [20] and for niflumic acid, 4.9 [21]. In these cases there are substituents having different electroatractor or electrodonor character in position 2. As stated above, the existence of an additional pyridine nitrogen in the herbicides compared with IMBA must originate an additional protonation-deprotonation equilibrium. So, since IMBA has three dissociation constants, the herbicides must have four.
Table 1 compiles the pK values for the imidazolinone herbicides found in the literature.
pK values of the imidazolinone herbicides.
Compound | pK1 | pK2 | pK3 |
Imazapyr (IMZP) | 1.9 [6–10] | 3.6 [6–10] | 10.8 [10] |
Imazapic (IMZC) | 2.1 [7] | 3.9 [7] | 11.1 [11] |
Imazethapyr (IMZE) | 2.1 [7,8] | 3.9 [7,8] | 10.5 [12] |
Imazamox (IMZM) | 2.09 [10] | 5.04 [10] | 10.8 [13] |
Imazaquin (IMZQ) | 1.8 [7] | 3.7 [7] | 11.03 [11] |
Taking into account that for IMBA there are two very close pK values, it is expected that for the rest of the herbicides this applies also. Potentiometric titrations have been made and in all cases the results were similar to those shown in Fig. 1 for imazapyr.

Potentiometric titration of a solution consisting of 4.97 × 10−3 M imazapyr and 2.48 × 10−3 M HCl with 4.825 × 10−3 M NaOH (standardized with potassium hydrogenftalate).
The results indicate that only one H+ ion is titrated in such conditions, being the pK values obtained close to those of pK2 shown in Table I. This means that the acidic pK values must correspond to a double protonation/dissociation equilibrium.
UV-visible spectra confirm the above conclusions. As can be seen in Fig. 2A for imazapyr, at pH < 3 the spectra show a main band centered about 194.5 nm, virtually pH-independent, and a second band having less intensity, having a maximum around 233.5 nm, which decreases as the pH becomes less acidic.
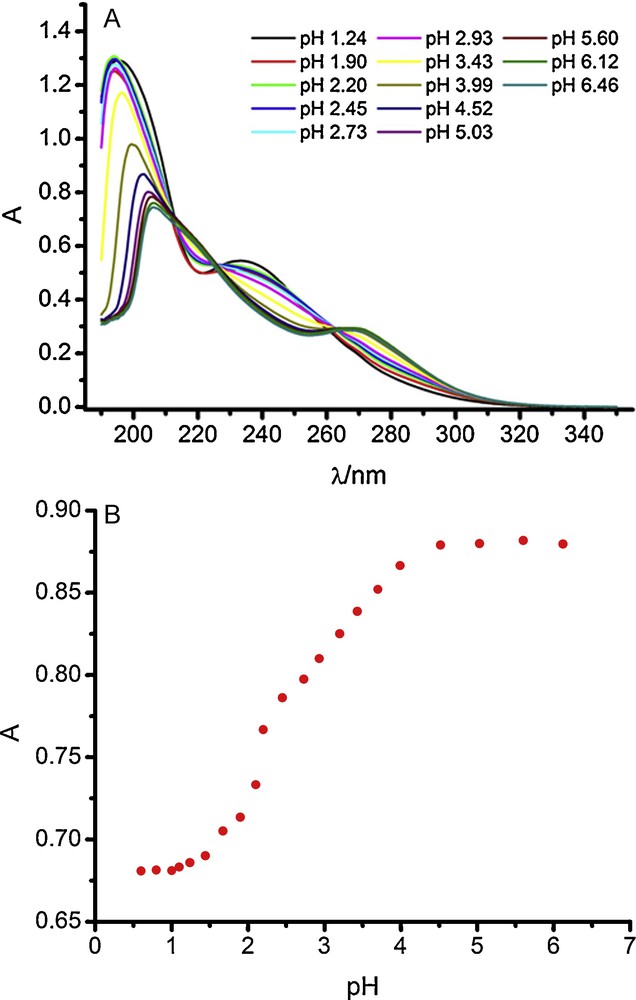
A. UV-visible spectra of 5 × 10−4 M imazapyr (IMZP). B. Plot of the absorbance vs. pH at 266 nm and a concentration of 1.5 × 10−4 M.
In the pH range 3–6.5 the main band decreases as the pH increases and the maximum wavelength shifts from 194.5 to 206 nm. At the same time, a new band centered at 269 nm appears.
The decrease of the main band with pH corresponds to only a pKa around 3.5. Nevertheless, the dependence of the band at 269 nm shown in Fig. 2B clearly corresponds to the occurrence of two pK values, the first of them around pH 2, as expected from the values given in Table I. The change in absorbance corresponding to this pK is very narrow, as is expected for two very close acid-base equilibria as in the case of IMBA [5]. This applies also for the rest of imidazolinone herbicides.
So, the pK1 values shown in Table I correspond to the protonation/dissociation equilibria of the pyridinic nitrogen and the carboxyl group, pK2 to the imminium nitrogen and pK3 to the dissociation of the imido nitrogen, as shown in Scheme 2 (similar structures can be written for imazaquin).

Dissociation pattern of imidazolinone herbicides.
Sorption to soil affects the activity and persistence of these herbicides [22], pH being one of the most important factors determining the strength of their binding to soil. It was assumed that the anionic form predominates from pH 6 to 9, this form being weakly bound to the soil or repulsed due to the presence of the negative charges in the soil colloids, this being the cause of their low adsorption to neutral and high pH soils. As the pH of the soil decreases, it was supposed that the amounts of the neutral and cationic forms of these herbicides increase and soil adsorption increases in consequence. Nevertheless, the Scheme 2 indicates that below pH 6 and down to pH c.a. 2.5, the dominant form of the herbicide is the double ion, having both positive and negative charges. This must be taken into account in discussing the effect of pH in the natural dynamics of imidazolinone herbicides, especially in their soil sorption behaviour.
3.2 Electrochemical reduction in acidic media
On mercury electrodes and at pH > 2, dc polarograms show, as a general feature, two reduction waves that will be denominated “wave 1” and “wave 2” according to the lower or higher reduction potential values at which they appear. Such waves are separated by 0.4-0.5 V, as can be seen in Fig. 3A for three representative herbicides. The limiting currents of the waves 1 and 2 are very similar, this meaning that the same number of electrons is involved in both reduction processes. From the comparison with the dc polarograms corresponding to IMBM [3] and IMBA [4] reductions it can be concluded that the waves 1 and 2 correspond to the uptake of two electrons per herbicide molecule.
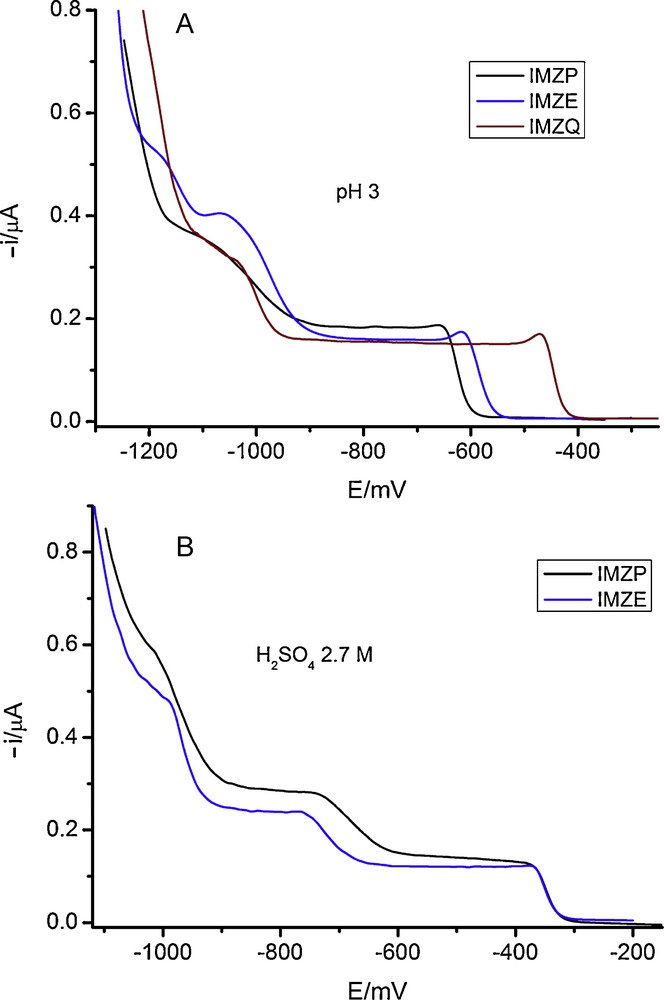
DC polarography of 1 × 10−4 M solutions of some imidazolinone herbicides.
In Fig. 3 it can be also shown that in strongly acidic media, the dc polarograms show a new wave (wave 3) appearing at more negative potentials, close to the background discharge. This wave changes with pH, approaching to the supporting electrolyte discharge and this effect preventing the analysis of the wave, other than the approximate half wave potential and limiting current values. In the case of imazaquin the wave is always much overlapped with the background discharge and its measurement is virtually impossible. So, differential pulse polarography will be used for the analysis of the reduction mechanism.
The observation of wave 3 caused the re-examination of the electrochemical behavior of IMBM and IMBA in strongly acidic media. For IMBM a shoulder very close to the background discharge is observed but for IMBA a well-defined peak (peak 3) was found close to the background discharge as can be seen in Fig. 4A.
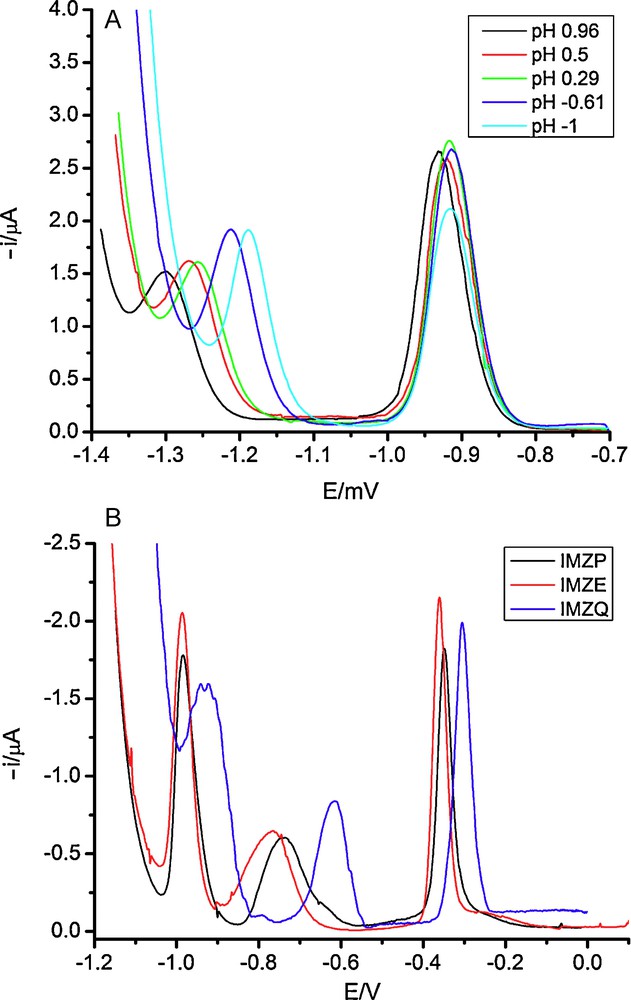
A. DP polarograms of 1 × 10−4 M Imazamethabenz acid in sulfuric media. pH values correspond to the acidity function H0 obtained from the sulfuric acid concentrations[23]. B. DP polarograms of 1 × 10−4 M of imidazolinone herbicides in 2.7 M H2SO4.
The waves shifted towards more negative potentials when the medium pH was increased. Wave 1 decreases in intensity above pH 7.5, disappearing at pH c.a. 10 for all herbicides studied.
The intensity of peak 3 (after elimination of the supporting electrolyte contribution) decreased as the pH was increased, disappearing at pH close to 2–3, depending on the concrete compound.
The analysis of the DP polarograms was performed by using the following equation (1) corresponding to first-order processes [24]:
(1) |
The signal was also observed in cyclic voltammetry, the overlapping with the electrolyte discharge being more evident. In all cases, no re-oxidation peaks were observed in the reverse scan, this indicating that the overall process is irreversible. When the glassy carbon electrode was used, the peak 3 was also observed as a shoulder in the more acidic solutions, but the irreversibility of the process was also evidenced.
Based on the above data it can be concluded that the rate-determining step of the reduction process taking place at the potentials corresponding to peak 3 is a chemical step placed after the first reversible electron transfer [24,26]. The peak area of peak 3 tended to be equal to the peak area of peak 2 in strongly acidic media, this meaning that the overall process is also bielectronic and the reduction mechanism is ECE [26]. Moreover, the decrease of the peak intensity with the pH indicates that at these potentials the species that is reduced is formed by protonation of the species generated at less negative potentials. In the case of IMBA the only reducible group is the imidazolinone ring [4] and the reduction process at the potentials corresponding to peak 3 must occur in this part of the molecule.
As can be seen in Fig. 4B, in the case of imidazolinone herbicides in strongly acidic media the DP polarograms show a peak appearing at very negative potentials, which must correspond to the same process as found for IMBA, that is, the reduction of the imidazole ring of the product obtained at less negative potentials. The peak intensity of these peaks, after elimination of the contribution of the supporting electrolyte, decreases when the pH was increased, and disappears at pH 2-3 in all cases.
Fig. 5 shows the peak potentials as functions of the pH for IMBM, IMBA and imazapir. The rest of herbicides are not shown to avoid complexity of the graph.
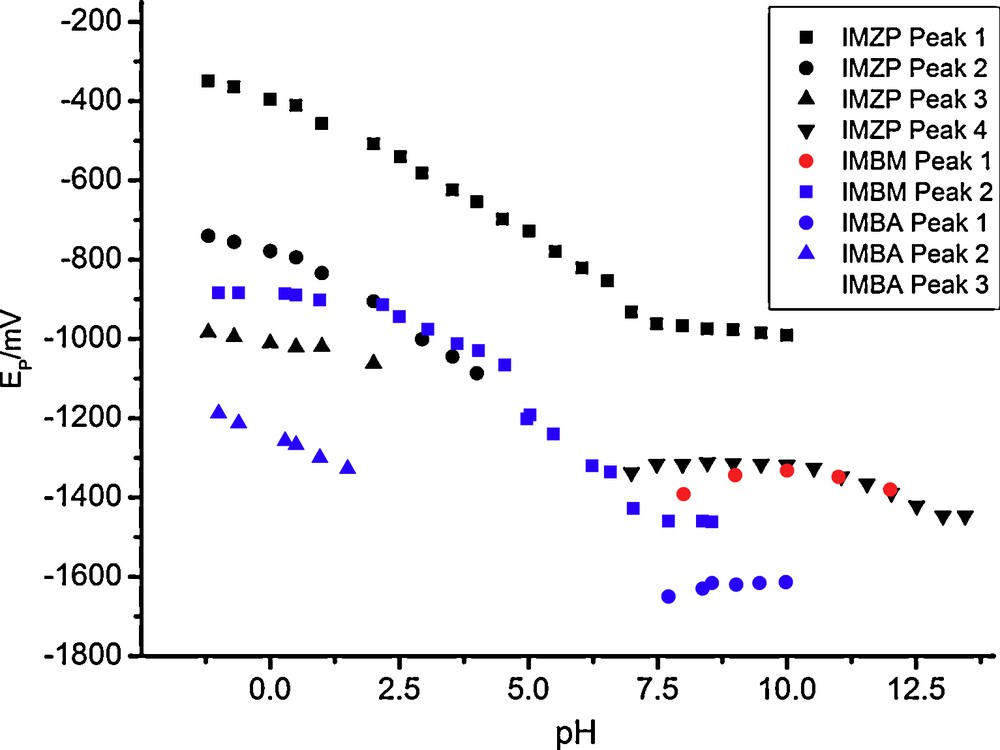
Plot of peak potentials vs. pH in DP polarography.
From this graph it can be concluded that peaks 1, 3 and 4 (that appearing in neutral-basic media) correspond to the reduction of the imidazolinone ring, the only reducible for IMBM and IMBA, being equivalent to peaks 1 and 2 of these compounds. The pH-dependence of such potentials is similar, though the presence of the pyridine ring instead the benzene ring leads to less negative reduction potentials for the herbicides (c.a. 250 mV for IMZP, IMZC, IMZE and IMZM and c.a. 350 mV for IMZQ). The peak not appearing for IMBM and IMBA but found for the rest of the imidazolinone herbicides, labeled as peak 2, must correspond to the pyridine ring reduction, probably in a similar manner as nicotinic acid and derivatives [15].
The b parameter in DP polarography of peaks 3 is in all cases close to 30 mV, different from the c.a. 60 mV found for this peak in the IMBA reduction (see an example for IMZE in Fig. 6). This means that the two electron transfers corresponding to this peak are both reversible.
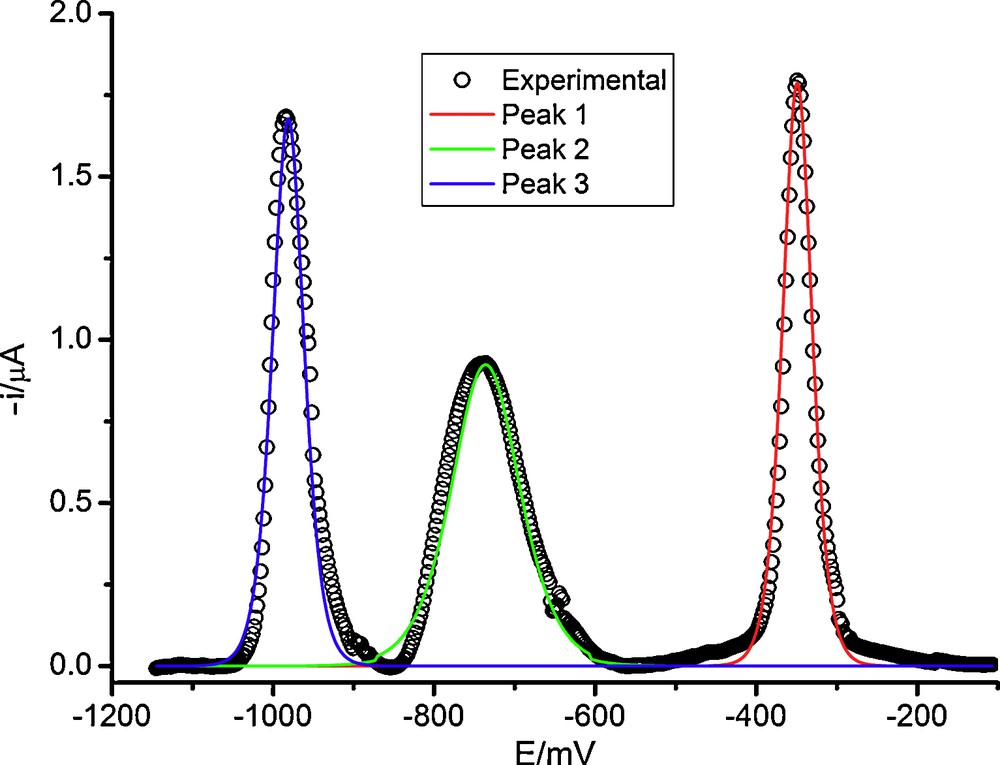
Comparison of experimental (circles) and theoretical (continuous lines) DP polarograms of IMZE in 0.7 M H2SO4.
Moreover, the cyclic voltammograms do not show oxidation peaks in the reverse scan, this meaning that the overall processes must be irreversible. The most probable pathway involves the occurrence of a chemical reaction placed after the two electron transfers and, since the medium is strongly acidic, this reaction must correspond probably to a protonation.
When a carbon electrode was used, the reduction signals shifted towards more negative potentials and the peak 3 was only observed as a shoulder in differential pulse voltammetry and at high contents in sulfuric acid. Linear-sweep voltammograms cannot be used to analyze these processes due to the proximity of the electrolyte discharge. So, mercury electrodes appear as more suitable for the examination of the redox properties of the imidazolinone herbicides in strongly acidic solutions.
Acknowledgements
Financial supports from Junta de Andalucía (Research Group FQM-0198), CICyT (Research Project CTQ2006-07224/PPQ) and Cordoba University are gratefully acknowledged.