1 Introduction
Triarylmethyl derivatives are an important class of organic compounds which have received considerable attention in recent years due to their wide range of activities and interesting properties [1–3]. Some TAM derivatives have been known to exhibit antioxidant properties, antitumor activity and inhibitory activity toward the histidine protein kinase [4]. These compounds have also been used as dyes [5,6] and as protecting groups for nucleosides and carbohydrates [3,7]. Due to their importance, considerable attention has been paid to develop efficient methods for the synthesis of TAMs.
Several synthetic methods such as Suzuki-Miyaura coupling of diarylmethyl carbonates with arylboronic acids in the presence of [Pd(η3-C3H5)Cl]2-DPPPent (1,5-bis(diphenylphosphino) pentane) catalyst [8] and alkylation of arenes with aromatic aldehydes catalyzed by trifluoromethanesulfonic acid (TFSA) or TFSA-trifluoroacetic acid (TFA) [9]), sulfuric acid [10], [Ir(COD)Cl]2-SnCl4 [11], AuCl3 or AuCl3/AgOTf [12], Cu(OTf)2 [13], FeCl3 [14], Yb(OTf)3 [15] and AcBr/ZnBr2/SiO2 [16] have been developed for the preparation of TAMs. However, in spite of their potential utility, most of the reported methods require high reaction temperatures [8,11,14], long reaction times [8,9,12,14,15] and excess amounts of catalyst [9,10] for successful reactions. Further, the use of toxic organic solvents [9,10,12–14,16], a corrosive catalyst [10] and formation of a side product [9] limit the usefulness of some of these methods, especially in large scale operation and lead to serious environmental and safety problems. Another disadvantage of the existing methods is that the catalysts are destroyed during the work-up procedure and can not be recovered and reused [8–16]. Consequently, the design of simple, efficient and green approaches for the synthesis of TAMs is still in demand.
In recent years, the use of solid acid catalysts has gained considerable attention in a wide range of organic transformations [17–20]. The ease of separation, recovery and re-use render the processes employing solid acid catalysts as green and economically viable processes. Among several solid acid catalysts used in organic reactions, silica sulfuric acid is one of the most attractive, because of its reusability, environmental compatibility, low cost, non-toxicity and operational simplicity. Due to these advantages, silica sulfuric acid has been used as an efficient catalyst in some important organic transformations [21–27]. This catalyst is prepared easily by the reaction of silica gel with chlorosulfonic acid [21].
In continuation of our interest towards development of useful synthetic methodologies [28–31], herein we wish to report a facile and environmentally-friendly method for the synthesis TAMs using silica sulfuric acid as a safe, inexpensive and reusable solid acid catalyst under solvent-free conditions (Scheme 1).

2 Experimental
Silica sulfuric acid (SSA) was prepared according to the literature [21]. The chemicals used in this work were purchased from Fluka and Merck chemical companies. The progress of reactions was monitored by TLC using 0.25 μm pre-coated silica gel plates. Melting points were determined using Stuart Scientific SMP2 apparatus and are uncorrected. 1H and 13C NMR (500 and 125 MHz) spectra were recorded on a Bruker-AC 500 spectrometer in CDCl3 using TMS as internal standard. FT-IR spectra were obtained as KBr pellets using a Nicolet-Impact 400D instrument in the range of 400–4000 cm−1. Elemental analysis was done on LECO, CHNS-932. Mass spectra were recorded on a Platform II spectrometer from Micromass; EI mode at 70 eV.
2.1 General procedure for the synthesis of diveratrylmethanes (DVMs) under solvent-free conditions
A mixture of veratrole 1 (402 mg, 3 mmol), aldehyde (1 mmol) and SSA (150 mg, 0.39 mmol) was heated at 65 °C for the appropriate time according to Table 2. The progress of the reaction was checked by TLC (n-hexane/ethyl acetate 10:4). After completion of the reaction, the mixture was cooled to room temperature and absolute EtOH (2 × 5 mL) was added. The catalyst was filtered by simple filtration and the pure product was obtained by recrystallization from EtOH (In the case of liquid products, the crude material was purified by column chromatography on silica gel).
Synthesis of diveratrylmethanes from the reaction of veratrole with aldehydes catalyzed by SSA under solvent-free conditionsa.
Entry | RCHO | Product | Time (min) | Yield (%)b |
1 | PhCHO (2a) | 3a | 20 | 78 |
2 | 3-NO2C6H4CHO (2b) | 3b | 15 | 94 |
3 | 2-ClC6H4CHO (2c) | 3c | 20 | 95 |
4 | 2,4-(Cl)2C6H3CHO (2d) | 3d | 18 | 82 |
5 | 4-BrC6H4CHO (2e) | 3e | 15 | 91 |
6 | 4-NCC6H4CHO (2f) | 3f | 20 | 92 |
7c | 4-OHCC6H4CHO (2g) | 3g | 8 | 86 |
8 | 4-FC6H4CHO (2h) | 3h | 20 | 85 |
9 | 3-CH3OC6H4CHO (2i) | 3i | 25 | 85 |
10 | 2-Naphtaldehyde (2j) | 3j | 25 | 87 |
11d | 4-HOC6H4CHO (2k) | 3k | 60 | Traces |
12d | 4-CH3OC6H4CHO (2l) | 3l | 60 | Traces |
13e | n-PrCHO (2m) | 3m | 10 | 93 |
14e | 2-Phenylpropionaldehyde (2n) | 3n | 10 | 94 |
a Reaction conditions: 3 mmol veratrole, 1 mmol aldehyde, 150 mg SSA at 65 °C.
b Isolated yield.
c Reaction condition: 1 mmol terephthalaldehyde 2g and 2.5 mmol veratrole 1.
d The starting materials were recovered from the reaction mixture.
e Reaction of aliphatic aldehydes were preformed at room temperature.
2.2 General procedure for the synthesis of TAMs under solvent-free conditions
A mixture of arene (3 mmol), aldehyde (1 mmol) and SSA (200 mg, 0.52 mmol) was heated at 65 °C for the time given in Table 3. After completion of the reaction as indicated by TLC (n-hexane/ethyl acetate 10:4), the reaction mixture was cooled to room temperature and absolute EtOH (2 × 5 mL) was added. The catalyst was filtered by simple filtration and the crude product was purified by recrystallization from EtOH or EtOH/H2O.
Synthesis of TAMs from the reaction of arenes with aldehydes catalyzed by SSA under solvent-free conditionsa.
Entry | Ar-H | Ar’CHO | Product | Time (min) | Yield (%)b |
1 | 25 | 91 | |||
2c | 60 | 93 | |||
3 | 15 | 94 | |||
4 | 15 | 89 | |||
5 | 25 | 86 | |||
6 | 25 | 87 | |||
7 | 5 | 95 | |||
8 | 5 | 94 |
a Reaction conditions: 3 mmol arene, 1 mmol aldehyde, 200 mg SSA at 65 °C.
b Isolated yield.
c The reaction was carried out with 7 mmol anisole and 1 mmol terephthalaldehyde in the presence of 350 mg of SSA.
2.3 Physical and spectroscopic data
1,2-Dimethoxy-4-((3,4-dimethoxyphenyl)(phenyl)methyl)benzene (Table 2, compound 3a). Mp 122–124 °C; IR (KBr): ν (cm−1) 2999, 2960, 1589, 1512, 1463, 1448, 1340, 1265, 1224, 1138, 1074, 1026, 958, 871, 817, 744, 704. 1H–NMR (500 MHz, CDCl3): δ (ppm) 7.32 (2H, t, J = 7.6 Hz, 3,5-H phenyl), 7.22 (1H, t, J = 7.5 Hz, 4-H phenyl), 7.13 (2H, d, J = 7.4 Hz, 2,6-H phenyl), 6.79 (2H, d, J = 8.3 Hz, 5-H veratryl), 6.68 (2H, d, J = 1.7 Hz, 2-H veratryl), 6.51 (2H, dd, J = 8.2 Hz, J = 1.9 Hz, 6-H veratryl), 5.45 (1H, s, Ar3CH), 3.86 (6H, s, OCH3), 3.77 (6H, s, OCH3). 13C–NMR (125 MHz, CDCl3): δ 148.85, 147.56, 144.36, 136.79, 129.30, 128.26, 126.27, 121.47, 112.94, 111.01, 55.96, 55.89, 55.85. MS (EI, 70 eV) (m/z, %): 365.16 ([M + 1]+, 21.07), 364.16 (M+, 85.95), 333.14 (100), 287.16 (46.9), 227.15 (22.93), 165.08 (35.33), 143.18 (34.50), 115.02 (29.75), 77.00 (24.59). Anal. Calcd for C23H24O4: C, 75.80; H, 6.64. Found: C, 75.37; H, 6.67.
1-(Bis(3,4-dimethoxyphenyl)methyl)-3-nitrobenzene (Table 2, compound 3b). Mp 154–155.5 °C; IR (KBr): ν (cm−1) 3084, 3003, 2947, 1589, 1516, 1463, 1342, 1028, 916, 869, 736. 1H–NMR (500 MHz, CDCl3): δ (ppm) 8.05–8.08 (1H, m, 4-H 3-nitrophenyl), 8.00 (1H, s, 2-H 3-nitrophenyl), 7.44–7.46 (2H, m, 5,6-H 3-nitrophenyl), 6.81 (2H, d, J = 8.3 Hz, 5-H veratryl), 6.65 (2H, d, J = 2.0 Hz, 2-H veratryl), 6.57 (2H, dd, J = 8.3 Hz, J = 2.0 Hz, 6-H veratryl), 5.53 (1H, s, Ar3CH), 3.86 (6H, s, OCH3), 3.77 (6H, s, OCH3). 13C–NMR (125 MHz, CDCl3): δ 149.16, 148.44, 148.04, 146.68, 135.35, 135.17, 129.12, 124.05, 121.51, 121.39, 112.72, 111.26, 55.91, 55.59. MS (EI, 70 eV) (m/z, %): 410.12 ([M + 1]+, 25.86), 409.11 (M+, 100), 378.06 (46.26), 287.18 (40.23), 226.13 (9.99), 152.10 (7.33), 139.08 (6.90), 76.93 (21.83). Anal. Calcd for C23H23NO6: C, 67.47; H, 5.66; N, 3.42. Found: C, 67.34; H, 5.64; N, 3.42.
4-((2-Chlorophenyl)(3,4-dimethoxyphenyl)methyl)-1,2-dimethoxybenzene (Table 2, compound 3c). Mp 136–137 °C; IR (KBr): ν (cm−1) 3078, 2997, 2933, 1589, 1514, 1465, 1413, 1332, 1263, 1139, 1026, 960, 869, 763, 704, 642. 1H–NMR (500 MHz, CDCl3): δ (ppm) 7.38 (1H, dd, J = 5.3 Hz, J = 3.7 Hz, 3-H 2-chlorophenyl), 7.17 (1H, t, J = 3.5 Hz, 4-H 2-chlorophenyl), 7.16 (1H, t, J = 3.6 Hz, 5-H 2-chlorophenyl), 6.96 (1H, dd, J = 5.5 Hz, J = 3.1 Hz, 6-H 2-chlorophenyl), 6.78 (2H, d, J = 8.3 Hz, 5-H veratryl), 6.64 (2H, d, J = 1.9 Hz, 2-H veratryl), 6.54 (2H, dd, J = 8.3 Hz, J = 1.9 Hz, 6-H veratryl), 5.84 (1H, s, Ar3CH), 3.86 (6H, s, OCH3), 3.77 (6H, s, OCH3). 13C–NMR (125 MHz, CDCl3): δ 148.93, 147.69, 142.01, 135.39, 134.54, 130.91, 129.65, 127.71, 126.55, 121.51, 113.03, 110.97, 55.86, 52.55. MS (EI, 70 eV) (m/z, %): 400.07 ([M + 2]+, 44.57), 398.07 (M+, 76.71), 367.05 (77.12), 287.09 (59.72), 143.05 (100), 127.53 (54.09), 100.91 (32.58), 76.90 (12.69). Anal. Calcd for C23H23ClO4: C, 69.26; H, 5.81. Found: C, 69.17; H, 5.78.
4-((2,4-Dichlorophenyl)(3,4-dimethoxyphenyl)methyl)-1,2-dimethoxybenzene (Table 2, compound 3d). Mp 151–152 °C IR (KBr): ν (cm−1) 3072, 2999, 2933, 1587, 1516, 1463, 1328, 1250, 1138, 1028, 960, 866, 815, 754, 638. 1H–NMR (500 MHz, CDCl3): δ (ppm) 7.39 (1H, d, J = 2.2 Hz, 3-H 2,4-dichlorophenyl), 7.15 (1H, dd, J = 8.5 Hz, J = 2.2 Hz, 5-H 2,4-dichlorophenyl), 6.88 (1H, d, J = 8.4 Hz, 6-H 2,4-dichlorophenyl), 6.78 (2H, d, J = 8.3 Hz, 5-H veratryl), 6.61 (2H, d, J = 2 Hz, 2-H veratryl), 6.51 (2H, dd, J = 8.3 Hz, J = 2.0 Hz, 6-H veratryl), 5.76 (1H, s, Ar3CH), 3.86 (6H, s, OCH3), 3.77 (6H, s, OCH3). 13C–NMR (125 MHz, CDCl3): δ 149.03, 147.85, 140.76, 135.15, 134.82, 132.77, 131.74, 129.41, 126.83, 121.41, 112.89, 111.03, 55.89, 55.86, 52.16. MS (EI, 70 eV) (m/z, %): 436.09 (M+ +4, 2.40), 434.06 ([M + 2]+, 10.16), 432.08 (M+, 18.97), 365.07 (7.59), 287.12 (9.77), 139.05 (30.80), 94.00 (37.72), 78.99 (100), 77.00 (50.89). Anal. Calcd for C23H22Cl2O4: C, 63.75; H, 5.12. Found: C, 63.49; H, 5.09.
4-((4-Bromophenyl)(3,4-dimethoxyphenyl)methyl)-1,2-dimethoxybenzene (Table 2, compound 3e). Mp 159–161 °C; IR (KBr): ν (cm−1) 2995, 2931, 1587, 1524, 1462, 1332, 1261, 1138, 1026, 947, 839, 804, 750, 572. 1H–NMR (500 MHz, CDCl3): δ (ppm) 7.40 (2H, d, J = 8.5 Hz, 3,5-H 4-bromophenyl), 6.99 (2H, d, J = 8.4 Hz, 2,6-H 4-bromophenyl), 6.79 (2H, d, J = 8.3 Hz, 5-H veratryl), 6.79 (2H, d, J = 1.9 Hz, 2-H veratryl), 6.58 (2H, dd, J = 8.3 Hz, J = 1.9 Hz, 6-H veratryl), 5.39 (1H, s, Ar3CH), 3.86 (6H, s, OCH3), 3.77 (6H, s, OCH3). 13C–NMR (125 MHz, CDCl3): δ 148.96, 147.75, 143.49, 136.12, 131.34, 131.03, 121.39, 120.19, 112.80, 111.09, 55.90, 55.89, 55.38. MS (EI, 70 eV) (m/z, %): 444.13 ([M + 2]+, 43.50), 442.12 (M+, 49.72), 413.09 (38.42), 411.08 (40.11), 287.12 (58.76), 151.93 (33.05), 142.79 (100), 100.90 (33.90), 77.00 (22.18). Anal. Calcd for C23H23BrO4: C, 62.31; H, 5.23. Found: C, 62.10; H, 5.22.
4-(Bis(3,4-dimethoxyphenyl)methyl)benzonitrile (Table 2, compound 3f). Mp 115–116 °C; IR (KBr): ν (cm−1) 2989, 2935, 2227, 1604, 1587, 1462, 1334, 1261, 1138, 1024, 960, 856, 810, 748. 1H–NMR (500 MHz, CDCl3): δ (ppm) 8.00–8.08 (1H, m, 3 or 5-H 4-cyanophenyl), 8.00 (1H, s, 3 or 5-H 4-cyanophenyl), 7.43–7.46 (2H, m, 2,6-H 4-cyanophenyl), 6.80 (2H, d, J = 8.3 Hz, 5-H veratryl), 6.65 (2H, d, J = 2.0 Hz, 2-H veratryl), 6.57 (2H, dd, J = 8.2 Hz, J = 2 Hz, 6-H veratryl), 5.53 (1H, s, Ar3CH), 3.86 (6H, s, OCH3), 3.76 (6H, s, OCH3). 13C–NMR (125 MHz, CDCl3): δ 149.15, 148.44, 148.03, 146.68, 135.35, 135.17, 129.13, 124.05, 121.52, 121.39, 112.70, 111.24, 55.91, 55.59. MS (EI, 70 eV) (m/z, %): 390.07 ([M + 1]+, 73.79), 389.05 (M+, 100), 385.04 (83.5), 287.05 (86.44), 252.06 (64.41), 190.04 (62.15), 151.03 (57.63), 143.19 (65.54), 120.76 (67.80), 77.02 (29.38).
4-(Bis(3,4-dimethoxyphenyl)methyl)benzaldehyde (Table 2, compound 3 g). Mp 128–129 °C; IR (KBr): ν (cm−1) 2999, 2929, 2839, 1699, 1602, 1516, 1465, 1344, 1267, 1138, 1026, 858, 794, 756. 1H–NMR (500 MHz, CDCl3): δ (ppm) 9.99 (1H, s. CHO), 7.81 (2H, d, J = 8.2 Hz, 3,5-H 4-formylphenyl), 7.3 (2H, d, J = 8.2 Hz, 2,6-H 4-formylphenyl), 6.81 (2H, d, J = 8.3 Hz, 5-H veratryl), 6.65 (2H, d, J = 2.0 Hz, 2-H veratryl), 6.59 (2H, dd, J = 8.3 Hz, J = 2.0 Hz, 6-H veratryl), 5.51 (1H, s, Ar3CH), 3.87 (6H, s, OCH3), 3.77 (6H, s, OCH3). 13C–NMR (125 MHz, CDCl3): δ 191.83, 151.61, 149.04, 147.89, 135.58, 134.82, 129.97, 129.77, 121.45, 112.79, 111.14, 56.10, 55.90. MS (EI, 70 eV) (m/z, %): 393.14 ([M + 1]+, 60.45), 392.13 (M+, 99.25), 361.11 (97.76), 287.09 (94.03), 255.06 (47.56), 164.97 (54.48), 127.80 (40.86), 114.88 (51.49), 100.91 (41.60), 78.87 (32.09), 76.89 (30.04). Anal. Calcd for C24H24O5: C, 73.45; H, 6.16. Found: C, 73.33; H, 6.15.
4-((4-Fluorophenyl)(3,4-dimethoxyphenyl)methyl)-1,2-dimethoxybenzene (Table 2, compound 3 h). Mp 124–125 °C; IR (KBr): ν (cm−1) 2999, 2960, 1602, 1506, 1463, 1446, 1330, 1263, 1159, 1095, 1026, 958, 858, 790, 750. 1H–NMR (500 MHz, CDCl3): δ (ppm) 7.06–7.10 (2H, m, 3,5-H 4-fluorophenyl), 6.97 (2H, t, J = 8.7 Hz, 2,6-H 4-fluorophenyl), 6.79 (2H, d, J = 8.3 Hz, 5-H veratryl), 6.65 (2H, d, J = 1.8 Hz, 2-H veratryl), 6.59 (2H, dd, J = 8.3 Hz, J = 1.6 Hz, 6-H veratryl), 5.43 (1H, s, Ar3CH), 3.86 (6H, s, OCH3), 3.77 (6H, s, OCH3). 13C–NMR (125 MHz, CDCl3): δ 161.41(d, 1JCF = 243.3 Hz), 148.92, 147.66, 140.12 (d, 4JCF = 3.1 Hz), 136.58, 130.70 (d, 3JCF = 7.8 Hz), 121.36, 115.02 (d, 2JCF = 21.0 Hz), 112.79, 111.05, 55.88, 55.85, 55.16. MS (EI, 70 eV) (m/z, %): 383.09 ([M + 1]+, 62.03), 382.09 (M+, 85.56), 367.04 (28.87), 352.08 (62.03), 351.09 (82.88), 287.06 (73.53), 245.05 (73.55), 213.01 (56.08), 198.97 (66.27), 182.97 (78.04), 169.95 (72.16), 150.98 (67.84), 142.99 (89.02), 127.53 (100), 108.92 (69.02), 78.87 (79.22), 76.92 (69.02).
1,2-Dimethoxy-4-((3,4-dimethoxyphenyl)(3-methoxyphenyl)methyl)benzene (Table 2, compound 3i). Mp 120–122 °C; IR (KBr): ν (cm−1) 3010, 2935, 1602, 1581, 1463, 1317, 1246, 1139, 1091, 1026, 958, 860, 767. 1H–NMR (500 MHz, CDCl3): δ (ppm) 7.21 (1H, t, J = 8.0 Hz, 5-H 3-methoxyphenyl), 6.78 (2H, d, J = 8.3 Hz, 5-H veratryl), 6.76 (1H, dd, J = 8.2 Hz, J = 2.2 Hz, 6-H 3-methoxyphenyl), 6.72 (1H, d, J = 7.7 Hz, 4-H 3-methoxyphenyl), 6.68–6.69 (3H, m, 2-H veratryl and 2-H 3-methoxyphenyl), 6.62 (2H, dd, J = 8.3 Hz, J = 1.9 Hz, 6-H veratryl), 5.41 (1H, s, Ar3CH), 3.86 (6H, s, OCH3 of veratryl), 3.77 (6H, s, OCH3 of veratryl), 3.74 (3H, s, OCH3 of 3-methoxyphenyl). 13C–NMR (125 MHz, CDCl3): δ 159.63, 148.83, 147.57, 146.01, 136.62, 129.16, 121.85, 121.44, 115.46, 112.89, 111.32, 111.00, 55.96, 55.88, 55.86, 55.13. MS (EI, 70 eV) (m/z, %): 395.10 ([M + 1]+, 73.79), 394.10 (M+, 100), 364.07 (71.56), 363.07 (98.93), 287.05 (93.51), 257.06 (81.08), 241.05 (57.30), 225.05 (57.30), 181.07 (68.11), 152.01 (69.73), 138.97 (64.86), 114.99 (51.35), 94.87 (39.46), 76.98 (55.68). Anal. Calcd for C24H26O5: C, 73.08; H, 6.64. Found: C, 72.89; H, 6.65.
2-(Bis(3,4-dimeyhoxyphenyl)methyl)naphthalene (Table 2, compound 3j). Mp 133–135 °C; IR (KBr): ν (cm−1) 3005, 2954, 1629, 1587, 1462, 1365, 1261, 1184, 1028, 964, 862, 761. 1H–NMR (500 MHz, CDCl3): δ (ppm) 7.81–7.83 (1H, m, 6-H naphthyl), 7.79 (1H, d, J = 8.5 Hz, 4-H naphthyl), 7.73–7.75 (1H, m, 7-H naphthyl), 7.52 (1H, s, 1-H naphthyl), 7.46 (1H, d, J = 3.5 Hz, 5-H naphthyl), 7.44 (1H, d, J = 3.4 Hz, 8-H naphthyl), 7.36 (1H, dd, J = 8.5 Hz, J = 1.6 Hz, 3-H naphthyl), 6.81 (2H, d, J = 8.3 Hz, 5-H veratryl), 6.79 (2H, d, J = 1.9 Hz, 2-H veratryl), 6.69 (2H, dd, J = 8.3, J = 1.9, 6-H veratryl), 5.65 (1H, s, Ar3CH), 3.88 (6H, s, OCH3), 3.79 (6H, s, OCH3). 13C–NMR (125 MHz, CDCl3): δ 148.97, 147.70, 142.07, 136.60, 133.49, 132.25, 128.09, 127.93, 127.84, 127.63, 127.60, 126.04, 125.66, 121.68, 113.05, 111.12, 56.13, 55.91, 55.90. MS (EI, 70 eV) (m/z, %): 415.11 ([M + 1]+, 69.51), 414.09 (M+, 100), 384.08 (63.11), 383.07 (91.97), 287.05 (80.00), 277.07 (65.88), 245.07 (57.06), 215.06 (52.94), 127.96 (61.18), 126.02 (71.18), 119.46 (68.24), 77.00 (25.29). Anal. Calcd for C27H26O4: C, 78.24; H, 6.32. Found: C, 77.95; H, 6.34.
1,1-Bis(3,4-dimethoxyphenyl)butane (Table 2, compound 3m). Oil; IR (KBr): ν (cm−1) 2995, 2931, 1589, 1463, 1425, 1259, 1141, 1028, 952, 856, 808, 754. 1H–NMR (500 MHz, CDCl3): δ (ppm) 6.79 (4H, s, 5,6-H veratryl), 6.74 (2H, s, 2-H veratryl), 3.88 (6H, s, OCH3), 3.87 (6H, s, OCH3), 3.81 (1H, t, J = 6.8 Hz, Ar2CH), 1.97 (2H, q, J = 7.7 Hz, CH2 (1) of n-propyl), 1.29 (2H, sext, J = 7.6 Hz, CH2 (2) of n-propyl), 0.93 (3H, t, J = 7.3 Hz, CH3 of n-propyl). 13C–NMR (125 MHz, CDCl3): δ 148.87, 147.36, 138.28, 119.58, 111.50, 111.24, 55.89, 50.17, 38.34, 21.19, 14.07. MS (EI, 70 eV) (m/z, %): 331.15 ([M + 1]+, 29.55), 330.14 (M+, 64.09), 288.13(62.27), 287.13 (84.55), 166.94 (90.91), 148.90 (100), 127.95 (61.82), 114.81 (66.36), 112.98 (85.91), 103.89 (65.91), 82.92 (80.45), 76.89 (49.09).
1,2-Dimethoxy-4-(1-(3,4-dimethoxyphenyl)-2-phenylpropyl)benzene (Table 2, compound 3n). Oil; IR (KBr): ν (cm−1) 3057, 2931, 1589, 1463, 1334, 1263, 1184, 1028, 856, 810, 761, 700. 1H–NMR (500 MHz, CDCl3): δ (ppm) 7.15–7.2 (4H, m, 2,3,5,6-H phenyl), 7.08 (1H, t, J = 6.9 Hz, 4-H phenyl), 6.96 (1H, dd, J = 8.2 Hz, J = 1.8 Hz, 6-H veratyll), 6.88 (1H, d, J = 1.7 Hz, 2-H veratryl), 6.85 (1H, d, J = 8.2 Hz, 5-H veratryl), 6.68 (1H, dd, J = 8.2 Hz, J = 1.9 Hz, 5’-H veratyl), 6.61 (1H, d, J = 8.3 Hz, 4’-H veratryl), 6.55 (1H, d, J = 1.8 Hz, 1’-H veratryl), 3.97 (1H, d, J = 11.1 Hz, Ar2CH), 3.89 (3H, s, OCH3), 3.86 (3H, s, OCH3), 3.67 (3H, s, OCH3), 3.66 (3H, s, OCH3), 3.45–3.49 (1H, m, PhCHCH3), 1.24 (3H, d, J = 6.8 Hz, CH3CHPh). 13C–NMR (125 MHz, CDCl3): δ 148.99, 148.26, 147.58, 146.94, 146.06, 136.97, 136.78, 128.12, 127.69, 125.82, 120.25, 120.16, 112.20, 111.95, 111.45, 110.92, 58.39, 55.97, 55.91, 55.74, 55.69, 44.89, 21.93. MS (EI, 70 eV) (m/z, %): 393.06 ([M + 1]+, 2.04), 392.05 (M+, 7.72), 287.09 (100), 257.03 (75.72), 197.04 (62.17), 167.01 (64.78), 152.03 (71.30), 128.04 (72.17), 115.00 (80.43), 105.02 (90.87), 77.00 (85.65).
1,4-Bis(bis(3,4-dimethoxyphenyl)methyl)benzene (4). Mp 173–176 °C; IR (KBr): ν (cm−1) 2993, 2924, 1587, 1514, 1463, 1332, 1263, 1186, 1028, 866, 788, 746. 1H–NMR (500 MHz, CDCl3): δ (ppm) 7.4 (4H, s, 2,3,5,6-H terephthyl), 6.78 (4H, d, J = 8.3 Hz, 5,5’-H veratryl), 6.67 (4H, d, J = 1.9 Hz, 2,2’-H veratryl), 6.61 (4H, dd, J = 8.3 Hz, J = 1.8 Hz, 6,6’-H veratryl), 5.41 (2H, s, Ar3CH), 3.86 (12H, s, OCH3), 3.76 (12H, s, OCH3). 13C–NMR (125 MHz, CDCl3): δ 148.81, 147.54, 142.27, 136.84, 129.14, 121.39, 112.94, 110.96, 55.88, 55.85, 55.61. Anal. Calcd for C40H42O8: C, 73.83; H, 6.51. Found: C, 73.46; H, 6.49.
1-(Bis(4-methoxyphenyl)methyl)-3-nitrobenzene (Table 3, compound 6a). Oil; IR (KBr): ν (cm−1) 3007, 2937, 1593, 1485, 1402, 1242, 1120, 1028, 808, 702. 1H–NMR (500 MHz, CDCl3): δ (ppm) 8.10–8.12 (1H, m, 4-H 3-nitrophenyl), 8.03 (1H, s, 2-H 3-nitrophenyl), 7.45–7.50 (2H, m, 5,6-H 3-nitrophenyl), 7.05 (4H, d, J = 8.7 Hz, 2,6-H of anisyl), 6.90 (4H, d, J = 8.7 Hz, 3,5-H anisyl), 5.59 (1H, s, Ar3CH), 3.85 (6H, s, OCH3). 13C–NMR (125 MHz, CDCl3): δ 158.85, 148.85, 147.40, 135.85, 135.31, 130.62, 129.33, 124.48, 121.87, 114.47, 55.70, 55.30. MS (EI, 70 eV) (m/z, %): 351.14 ([M + 2]+, 3.7) 350.14 ([M + 1]+, 23.53), 349.14 (M+, 100), 332.13 (22.15), 318.11 (37.13), 227.22 (98.53), 152.11 (57.72), 77.13 (14.98).
1,4-Bis(bis(4-methoxyphenyl)methyl)benzene (Table 3, compound 6b). Mp 114–116C; IR (KBr): ν (cm−1) 3001, 2951, 1608, 1581, 1462, 1301, 1246, 1111, 1033, 813, 759. 1H–NMR (500 MHz, CDCl3): δ (ppm) 7.04–7.08 (12H, m, 2,2’,6,6’-H of anisyl and the hydrogens of terephthyl), 6.85 (8 H, d, J = 16.7 Hz, 3,3’,6,6’-H anisyl), 5.46 (2H, s, Ar3CH), 3.81 (12H, s, OCH3). 13C–NMR (125 MHz, CDCl3): δ 158.39, 142.76, 137.02, 130.70, 129.56, 114.06, 55.67, 55.30. MS (EI, 70 eV) (m/z, %): 532.29 ([M + 2]+, 7.58), 531.26 ([M + 1]+, 28.79), 530.26 (M+, 100), 423.20 (16.48), 303.14 (66.67), 227.19 (60.98), 152.10 (37.12), 121.18 (51.14), 77.17 (19.03). Anal. Calcd for C36H34O4: C, 81.48; H, 6.46. Found: C, 81.36; H, 6.45.
2-((4-Chlorophenyl)(2-methoxy-5-methylphenyl)methyl)-1-methoxy-4-methylbenzene (Table 3, compound 6c). Mp 153–155 °C; IR (KBr): ν (cm−1) 3012, 2943, 1610, 1498, 1404, 1288, 1109, 1031, 885, 850, 752, 621. 1H–NMR (500 MHz, CDCl3): δ (ppm) 7.25 (2H, d, J = 8.2 Hz, 2,6-H 4-chlorophenyl), 7.04 (4H, t, J = 9.2 Hz, 4,5-H anisyl), 6.82 (2 H, d, J = 8.2 Hz, 3,5-H 4-chlorophenyl), 6.63 (2 H, d, J = 1.2 Hz, 2-H anisyl), 6.16 (1H, s, Ar3CH), 3.71 (6 H, s, OCH3), 2.25 (6H, s, Me). 13C–NMR (125 MHz, CDCl3): δ 155.63, 143.29, 132.30, 131.73, 131.09, 131.08, 129.71, 128.44, 128.18, 111.36, 56.36, 43.07, 21.21. MS (EI, 70 eV) (m/z, %): 368.09 ([M + 2]+, 36.45) 367.09 ([M + 1]+, 26.07), 366.08 (M+, 100), 351.06 (28.76), 209.13 (52.04), 165.07 (61.65), 135.04 (65.85), 105.14 (65.94), 77.04 (31.80). Anal. Calcd for C23H23ClO2: C, 75.30; H, 6.32. Found: C, 75.15; H, 6.33.
1-Methoxy-2-((2-methoxy-5-methylphenyl)(p-tolyl)-4-methylbenzene (Table 3, compound 6d). Mp 119–120 °C; IR (KBr): ν (cm−1) 3007, 2922, 1608, 1566, 1496, 1313, 1236, 1107, 1029, 889, 806, 704. 1H–NMR (500 MHz, CDCl3): δ (ppm) 7.09 (2H, d, J = 7.9 Hz, 2-H 4-methylphenyl), 7.03 (2H, dd, J = 8.2 Hz, J = 1.9 Hz, 4-H anisyl), 6.99 (2H, d, J = 8.0 Hz, 3-H 4-methylphenyl), 6.81 (2H, d, J = 8.2 Hz, 5-H anisyl), 6.68 (2H, d, J = 2.0 Hz, 2-H anisyl), 6.18 (1H, s, Ar3CH), 3.71 (6H, s, OCH3), 2.36 (3H, s, Me), 2.24 (6H, s, methyl of anisyl). 13C–NMR (125 MHz, CDCl3): δ 155.73, 141.35, 135.38, 133.19, 131.16, 129.66, 129.58, 129.06, 127.84, 111.41, 56.49, 43.02, 21.51, 21.24. MS (EI, 70 eV) (m/z, %): 347.23 ([M + 1]+, 24.58), 346.21 (M+, 90.83), 315.24 (63.33), 209.19 (31.46), 135.24 (47.29), 105.21 (100), 91.15 (42.57), 77.15 (22.5). Anal. Calcd for C24H26O2: C, 83.20; H, 7.56. Found: C, 82.97; H, 7.55.
4-Chloro-2-((5-chloro-2-methoxyphenyl)(4-nitrophenyl)methyl)-1-methoxybenzene (Table 3, compound 6e). Mp 168–170 °C; IR (KBr): ν (cm−1) 3007, 2935, 1593, 1485, 1435, 1402, 1290, 1240, 1120, 1068, 1026, 887, 835, 794, 702, 644. 1H–NMR (500 MHz, CDCl3): δ (ppm) 8.16 (2H, d, J = 8.6 Hz, 2, 6-H 4-nitrophenyl), 7.26 (2H, dd, J = 8.7 Hz, J = 2.3 Hz, 4-H anisyl), 7.23 (2H, d, J = 8.7 Hz, 5-H anisyl), 6.86 (2H, d, J = 8.7 Hz, 3, 5-H 4-nitrophenyl), 6.72 (2H, d, J = 2.3 Hz, 2-H anisyl), 6.15 (1H, s, Ar3CH), 3.72 (6 H, s, OCH3). 13C–NMR (125 MHz, CDCl3): δ 156.06, 150.83, 147.04, 132.34, 130.25, 129.99, 128.53, 125.96, 123.96, 112.51, 56.29, 44.03. MS (EI, 70 eV) (m/z, %): 420.95 ([M + 4]+, 12.25), 418.96 ([M + 2]+, 68.42), 417.98 ([M + 1]+, 23.48), 416.95 (M+, 100), 381.98 (90.28), 155.10 (93.93), 121.14 (78.95). Anal. Calcd for C21H17Cl2NO4: C, 60.30; H, 4.10; N, 3.35. Found: C, 60.17; H, 4.12; N, 3.34.
4-Chloro-2-((5-chloro-2-methoxyphenyl)(2-chlorophenyl)methyl)-1-methoxybenzene (Table 3, compound 6f). Mp 139–140 °C; IR (KBr): ν (cm−1) 3062, 2935, 1593, 1485, 1317, 1242, 1120, 1026, 893, 810, 754, 638. 1H–NMR (500 MHz, CDCl3): δ (ppm) 7.41 (1H, dd, J = 7.8 Hz, J = 1.4 Hz, 6-H 2-chlorophenyl), 7.23 (2H, dd, J = 8.8 Hz, J = 2.6 Hz, 4-H anisyl), 7.2 (1H, dd, J = 5.9 Hz, J = 1.9 Hz, 4-H 2-chlorophenyl), 7.17 (1H, dd, J = 7.4 Hz, J = 1.4 Hz, 5-H 2-chlorophenyl), 6.87 (1H, dd, J = 7.6 Hz, J = 1.7 Hz, 3-H 2-chlorophenyl), 6.76 (2H, d, J = 8.7 Hz, 5-H anisyl), 6.69 (2H, d, J = 2.6 Hz, 2-H anisyl), 6.41 (1H, s, Ar3CH), 3.72 (6 H, s, OCH3). 13C–NMR (125 MHz, CDCl3): δ 156.33, 140.52, 134.95, 132.87, 130.42, 130.18, 129.81, 128.28, 128.07, 126.92, 125.75, 112.48, 56.45, 41.31. MS (EI, 70 eV) (m/z, %): 410.06 ([M + 4]+, 12.11), 408.07 ([M + 2]+, 34.54), 406.08 (M+, 35.57), 155.13 (71.65), 125.08 (100), 91.12 (37.63). Anal. Calcd for C21H17Cl3O2: C, 61.86; H, 4.20. Found: C, 61.68; H, 4.17.
2-((4-Bromophenyl)(2,4,6-trimethoxyphenyl)-1,3,5-trimethoxybenzene (Table 3, compound 6 g). Mp 149–151 °C; IR (KBr): ν (cm−1) 3005, 2939, 1591, 1485, 1332, 1228, 1058, 950, 873, 810, 596. 1H–NMR (500 MHz, CDCl3): δ (ppm) 7.30 (2H, d, J = 8.4 Hz, 2-H 4-bromophenyl), 6.97 (2H, d, J = 8.3 Hz, 3-H 4-bromophenyl), 6.20 (1H, s, Ar3CH), 6.15 (4H, s, 3,5-H trimethoxyphenyl), 3.83 (6 H, s, OCH3), 3.56 (12H, s, OCH3). 13C–NMR (125 MHz, CDCl3): δ 160.20, 159.79, 145.40, 130.41, 130.13, 118.16, 113.95, 92.18, 56.50, 55.59, 37.07. MS (EI, 70 eV) (m/z, %): 504.21 ([M + 2]+, 2.57), 502.13 (M+, 2.64), 179.11 (14.18), 69.07 (64.25), 71.13 (21.77), 55.07 (100.00). Anal. Calcd for C25H27BrO6: C, 59.65; H, 5.41. Found: C, 59.48; H, 5.43.
2-((4-Chlorophenyl)(2,4,6-trimethoxyphenyl)-1,3,5-trimethoxybenzene (Table 3, compound 6 h). Mp 164–165 °C; IR (KBr): ν (cm−1) 2999, 2937, 1587, 1489, 1332, 1228, 1060, 950, 875, 813, 677. 1H–NMR (500 MHz, CDCl3): δ (ppm) 7.11 (2H, d, J = 8.5 Hz, 2-H 4-chlorophenyl), 6.98 (2H, d, J = 8.5 Hz, 3-H 4-chlorophenyl), 6.17 (1H, s, Ar3CH), 6.11 (4H, s, 3,5-H trimethoxyphenyl), 3.79 (6 H, s, OCH3), 3.53 (12H, s, OCH3). 13C–NMR (125 MHz, CDCl3): δ 159.80, 159.36, 144.41, 129.64, 129.25, 127.05, 113.75, 91.84, 56.10. 55.17, 36.63. MS (EI, 70 eV) (m/z, %): 460.11 ([M + 2]+, 25.56), 458.09 (M+, 67.78), 290.14 (100.00), 179.19 (60.56), 125.17 (49.72), 77.13 (18.06). Anal. Calcd for C25H27ClO6: C, 65.43; H, 5.93. Found: C, 64.98; H, 5.94.
3 Results and discussion
Initially, the effect of different parameters such as solvent, the amount of catalyst, temperature and reactant ratio were examined in the reaction of veratrole (1) with 3-nitrobenzaldehyde (2b) in the presence of SSA as a model reaction (Table 1). The model reaction was performed in solvents such as EtOH, CH3CN, CHCl3 and acetone and also under solvent-free conditions (entries 1–5). The reaction under solvent-free conditions gave the best result (entry 5). To determine the appropriate molar ratio of veratrole to aldehyde, the model reaction was investigated with different molar ratios of reactants and the best result was obtained with 3:1 molar ratio of veratrole to aldehyde (entry 5). Using lower amount of veratrole resulted in lower yields (entry 6), while higher amount of veratrole did not improve the reaction time and yield (entry 7). To find the optimized amount of silica sulfuric acid, the reaction was carried out in the presence of different amounts of the catalyst. Maximum yield was obtained with 150 mg of the catalyst (entry 5). Further increase in amount of silica sulfuric acid did not affect the product yield (entry 8), but a lower amount of the catalyst led to lower yield (entry 9). Indeed in the absence of the catalyst, the desired product was obtained in only 5% yield after 1 h (entry 10). Furthermore, the effect of reaction temperature was examined (entries 11–13) and we found that 65 °C is the optimal temperature (entry 5) and the reaction was incomplete at lower temperatures even after 2 h (entries 12 and 13). The model reaction was also carried out in the presence of sulfuric acid under similar experimental conditions (molar ratio and temperature); the corresponding triarylmethane 3b was obtained in only 26% yield. Further, SSA as a solid acid, in comparison with sulfuric acid, offers the advantages of easy separation, non-corrosiveness and low pollution effects.
Optimization of the reaction conditions for the SSA catalyzed reaction of veratrole 1 with 3-nitrobenzaldehyde 2b.
Entry | SSA (mg) | Veratrole (mmol) | Aldehyde (mmol) | Solvent | T (°C) | Time (min) | Yield (%)a |
1 | 150 | 3 | 1 | EtOH | Reflux | 60 | 13 |
2 | 150 | 3 | 1 | CH3CN | Reflux | 60 | 15 |
3 | 150 | 3 | 1 | Acetone | Reflux | 60 | 23 |
4 | 150 | 3 | 1 | CHCl3 | Reflux | 60 | 45 |
5 | 150 | 3 | 1 | Solvent-free | 65 | 15 | 94 |
6 | 150 | 2.5 | 1 | Solvent-free | 65 | 20 | 71 |
7 | 150 | 3.5 | 1 | Solvent-free | 65 | 15 | 94 |
8 | 200 | 3 | 1 | Solvent-free | 65 | 15 | 94 |
9 | 100 | 3 | 1 | Solvent-free | 65 | 20 | 80 |
10 | – | 3 | 1 | Solvent-free | 65 | 60 | 5 |
11 | 150 | 3 | 1 | Solvent-free | 75 | 15 | 94 |
12 | 150 | 3 | 1 | Solvent-free | 50 | 20 | 82b |
13 | 150 | 3 | 1 | Solvent-free | RT | 25 | 46b |
a Isolated yield.
b No further progress was observed even after 2 h.
Encouraged by our initial studies, we then investigated the generality and versatility of this method. A wide range of aromatic aldehydes were investigated for their reaction with veratrole in the presence of SSA under the optimized reaction conditions and the results are summarized in Table 2. The type of substituents on the aromatic aldehydes has significant influence on the yield of the product and reaction time. The aldehydes with electron-withdrawing groups (entries 2–9) afforded the desired products in high yields within short reaction times, while an aromatic ring with electrondonating groups, such as hydroxy and methoxy groups failed to produce the products and the starting materials were recovered from the reaction mixture (entries 11 and 12). It is important to note that 2-naphthaldehyde did not yield the corresponding TAM in the reaction with veratrole in the presence of sulfuric acid and only starting materials were recovered from the reaction mixture [10]. In contrast, the reaction of 2-naphthaldehyde with veratrole was performed efficiently in the presence of SSA within 25 min to afford the desired TAM in 87% yield (entry 10). More gratifyingly, this method is suitable not only for aromatic aldehydes, but also for aliphatic aldehydes, such as butanal with 93% yield and 2-phenylpropionaldehyde with 94% yield (entries 13 and 14). Another noteworthy feature of our protocol lies in the reaction of dialdehyde such as terephthalaldehyde (2g) with veratrole. Using a 2.5:1 molar ratio of veratrole to terephthalaldehyde, only one aldehyde moiety reacted selectively and the corresponding TAM was obtained in 86% yield. When veratrole was used in large excess (7 equiv. with respect to terephthalaldehyde), both aldehyde groups reacted and the desired product was obtained in 77% yield (Scheme 2). Such selectivity can be considered as an important practical achievement in the synthesis of TAMs.

The probable reaction pathway for SSA catalyzed synthesis of TAMs using veratrole and aldehydes is shown in Scheme 3. The reaction proceeds via a series of prototropic shift. The aldehyde is first activated by protonation with SSA to give A. Nucleophilic attack of veratrole on A affords B and then C which in turn is activated by the catalyst to afford D. Nucleophilic attack of second molecule of veratrole to D gives E which is subsequently converted to the desired product and releases the catalyst for the next catalytic cycle.
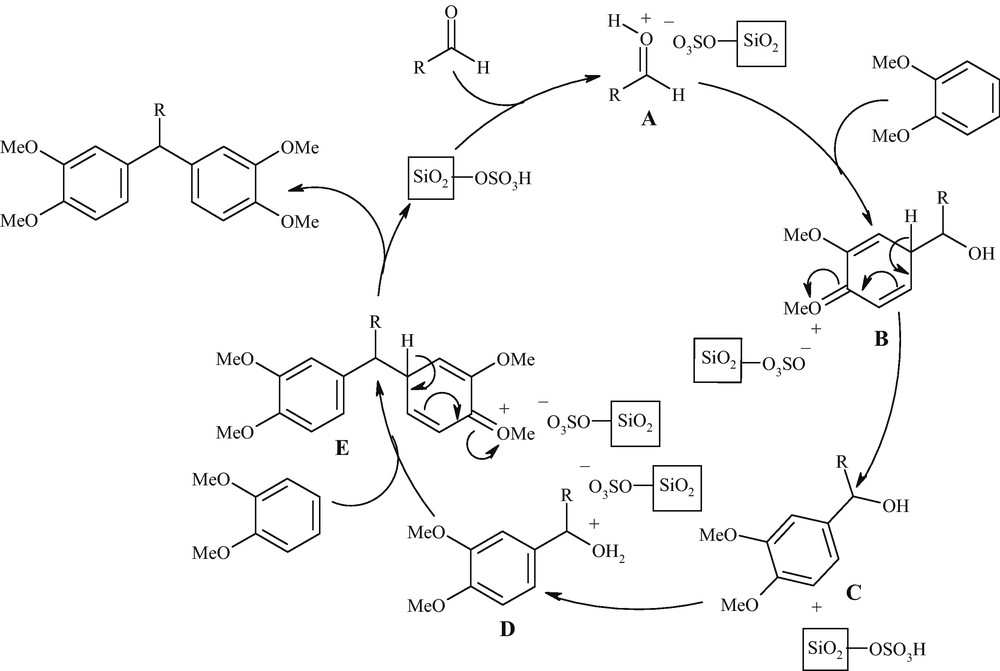
In order to show the efficiency and applicability of this method further, the reaction of other arenes such as anisole and its derivatives with aldehydes catalyzed by SSA under solvent-free conditions was also investigated. As shown in Table 3, treatment of a series of aldehydes with anisole and its derivatives in the presence of catalytic amount of SSA provided the corresponding TAM in high yields.
In view of green chemistry, we then turned our attention towards the reusability of the catalyst. The reusability of SSA was examined through a series of sequential reaction of veratrole with 3-nitrobenzaldehyde. After completion of the reaction, EtOH was added and SSA catalyst was separated by simple filtration and dried at 70 °C. The recovered catalyst was reused in subsequent reactions without significant decrease in its activity even after five runs (Table 4).
Reusability of SSA in synthesis of DVMs.
Run | Time (min) | Yield (%)a |
1 | 15 | 94 |
2 | 15 | 94 |
3 | 15 | 92 |
4 | 15 | 90 |
5 | 20 | 88 |
6 | 20 | 87 |
a Isolated yield.
In conclusion, we have developed a novel and facile method for the synthesis of TAMs in the presence of SSA as a cheap, safe, easy to handle and heterogeneous catalyst under solvent-free conditions. In addition, operational simplicity, mild reaction conditions, high yields, fast reaction, reusability of the catalyst and green synthesis avoiding toxic solvents are noteworthy advantages and make this method economic and environmentally-benign for the synthesis of TAMs.
Acknowledgements
Financial support of this work by Center of Excellence of Chemistry and Research Council of University of Isfahan is gratefully acknowledged.