1 Introduction
Nanoscience and nanotechnology is an amazing and growing research area that includes the preparation, characterization and application of nanostructures with various shapes and sizes in many chemical transformations. Nanoparticle properties are significantly different in their bulk analogues due to their high surface-to-volume ratio and coordination sites which provide a larger number of active sites per unit area [1]. Recently, magnetic Fe3O4 nanoparticles have gained extensive interests because of their wide use in several areas, including drug delivery, medicinal applications, remediation and industry [2]. In comparison with other nanocatalysts, Fe3O4 nanoparticles (nano-Fe3O4) in particular, are environmentally benign, economic and comparatively anti-toxic [3]. An important property of these nanoparticles is simple and convenient separation from the reaction media by a simple magnetic separation. In recent years, magnetic nanoparticles were used as an efficient catalyst in many organic transformations including regioselective synthesis of 3-[(2-chloroquinolin-3-yl)methyl]pyrimidin-4(3H)ones [4], synthesis of propargylic amines [5], coupling of phenols with aryl halides [6], synthesis of α-aminonitriles [7], Suzuki reaction [8], synthesis of quinoxalines [9], synthesis of sulfonamides [10], Sonogashira–Hagihara reaction [11], Paal-Knorr reaction, aza-Michael addition and pyrazole synthesis [12].
Research in multicomponent reactions (MCRs) is an encouraging and hot topic of organic chemistry, because of their advantageous in preparation of small molecule heterocyclic libraries and in drug discovery procedures [13]. MCRs are efficient, environmentally friendly, fast, atom economic and time saving. They supply an effective tool for the preparation of various compounds with pharmaceutical and biological properties [14], including heterocyclic compounds [15].
MCRs of aldehydes, dimedone and amines have received much attention in recent years. During the last decades, great consideration has been paid to the preparation of acridine derivatives due to their highly absolute biological and physiological activities such as anti-cancer [16], cytotoxic [17], anti-tumor [18], anti-multidrug-resistant [19], antimicrobial [20] and anti-fungal properties [21].
There are several methods reported in the literature for the synthesis of 1,8-dioxo-decahydroacridines via three-component coupling of aldehydes, dimedone and amines in the presence of diverse catalysts including ceric ammonium nitrate (CAN) [22], fluorotailed acidic imidazolium salts [23], 1-butyl-3-methylimidazolium bromide [bmim]Br [24], 1-methylimidazolium triflouroacetate [Hmim]TFA [25], proline [26], amberlyst-15 [27], carbon-based solid acid (SBSA) [28], silica-bonded N-propyl sulfamic acid (SBNPSA) [29] and 4-dodecylbenzenesulfonic acid (DBSA) [30]. However, many of these methods suffer from disadvantages such as toxic organic solvents, unsatisfactory yields, long reaction times, expensive catalysts, laborious work-up procedures, the requirement of special apparatus and harsh reaction conditions. Thus, the development of simple, efficient, high-yielding and eco-friendly methods using new catalysts for the synthesis of these compounds would be highly desirable.
In accordance with the above mentioned points related to the important growth of nanotechnology and MCRs in the synthesis of heterocyclic compounds and also in continuation of our research on the application of nanocatalysts in organic synthesis [31–34], herein we wish to report an efficient and novel method for the synthesis of 1,8-dioxo-decahydroacridines via one-pot MCRs of aldehydes, dimedone and aromatic amines in high yields and short reaction times by using nano-Fe3O4 with high specific surface area and average crystalline size of 40 nm as a green, robust and easily recoverable catalyst (Scheme 1).
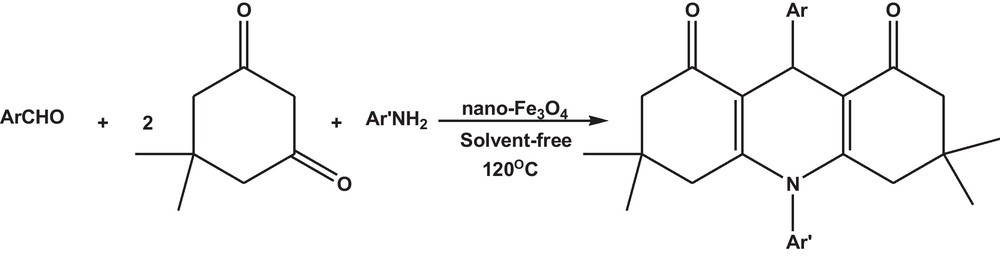
Three-component reaction of aldehydes, dimedone and amines catalyzed by Fe3O4 nanoparticles.
2 Results and discussion
Fe3O4 nanoparticles were prepared according to the procedure reported by Zhang et al. [9] from the reaction of FeCl2.4H2O and FeCl3.6H2O with solutions of hydrochloric acid and then sodium hydroxide.
The XRD pattern of the Fe3O4 nanoparticles is shown in Fig. 1. All reflection peaks can be readily indexed to pure cubic crystal phase of Fe3O4 with F-33 mspace group (JCDPS No. 75-0449). Also no specific peak due to any impurities was observed. The broad peaks indicate that the particles are in nanoscale size. The crystallite size diameter (D) of the magnetic nanoparticles has been calculated by Debye–Scherrer equation (D = Kλ/βcosθ), where β FWHM (full-width at half-maximum or half-width) is in radians and θ is the position of the maximum of diffraction peak, K is the so-called shape factor, which usually takes a value of about 0.9, and λ is the X-ray wavelength (λ = 1.5406 Å for Cu Kα). Average crystallite size of the prepared nano-Fe3O4 has been found to be 40 nm.
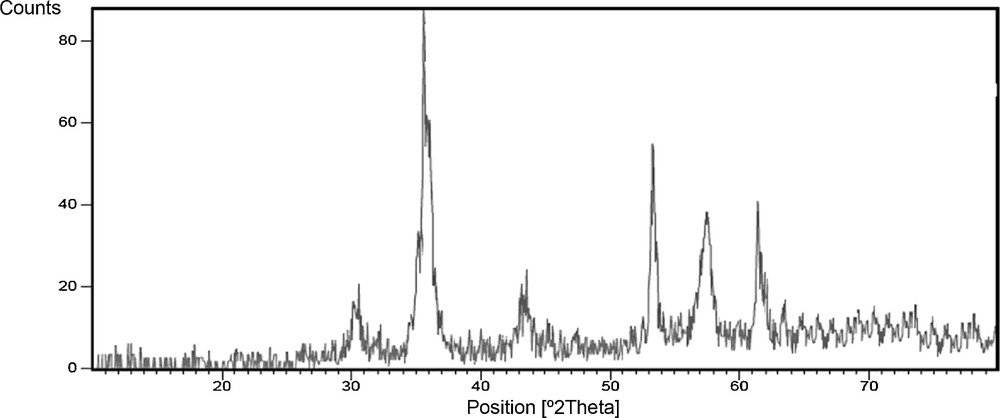
The XRD pattern of Fe3O4 nanoparticles.
The XRD patterns of magnetite and maghemite are very similar and the lattice parameter must be precisely determined. Magnetite is an inverse-spinel with the lattice parameter of 8.3941 Å. Cations are arranged with one Fe3+ per filled tetrahedral hole, and Fe2+ and the remaining Fe3+ randomly distributed in the octahedral holes. This places half of the smaller cations, Fe3+, in the smaller tetrahedral sites (as compared to the larger Fe2+ cations and the larger octahedral sites).
The lattice parameter of synthesized magnetite is 8.3876 Å, which is consistent with the expected lattice parameter for Fe3O4 particles. This value is closer to that of stoichiometric magnetite (8.3941 Å) than that of maghemite (8.346 Å) [35,36].
In addition, the specific surface area was measured by nitrogen physisorption (the BET method), the specific surface area was approximately 98 m2/g. Also, the theoretical particle size was calculated from the surface area and magnetite density (5.18 g/cm3) from the equation was 11.8 nm.
In order to investigate the morphology and particle size of Fe3O4 nanoparticles, SEM images of magnetic nanoparticles are presented in Fig. 2. These results show that spherical Fe3O4 nanoparticles were obtained with an average diameter of 45 nm as confirmed by XRD analysis.
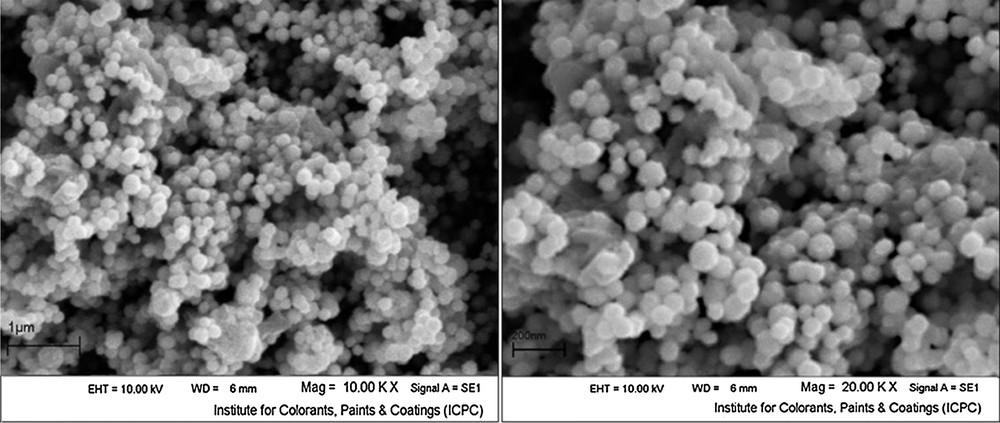
SEM image of Fe3O4 nanoparticles.
The IR spectra of prepared Fe3O4 nanoparticles is shown in Fig. 3. The results in Fig. 3 show that the data are the same as reported in literature. A strong peak at around 570 cm−1 is related to Fe-O stretching frequency. Whereas the IR spectra of Fe2O3 reported in literature is more complicated in comparison with the IR spectra of magnetite [37–40]. Difference of IR spectra of maghemite from magnetite is related to presence of vacancies within the OH sites, and the absence of Fe2+ cations.
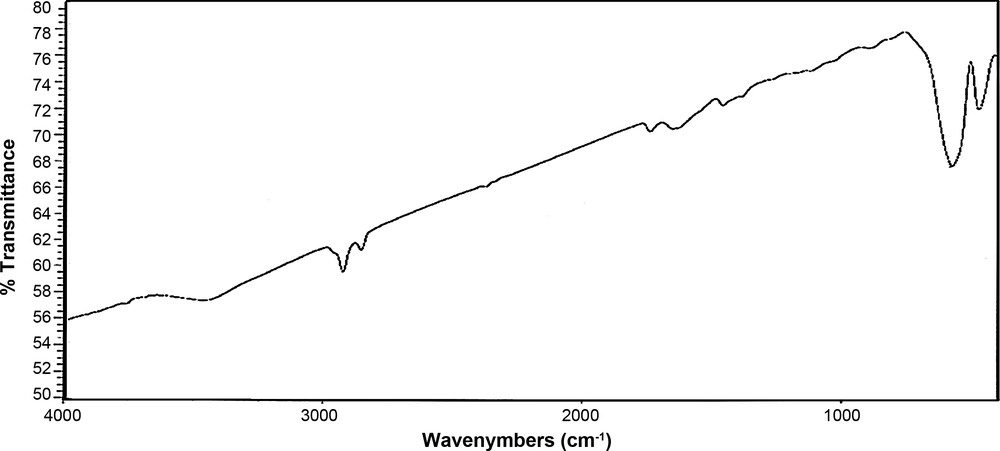
FT-IR spectrum of Fe3O4 nanoparticles.
In early studies, to optimize the reaction conditions, the reaction of benzaldehyde, dimedone and aniline was chosen as the model reaction for the one-pot synthesis of the corresponding acridine derivative.
The reaction conditions were optimized on the basis of the catalyst, solvent and different temperature for the synthesis of 1,8-dioxo-decahydroacridines.
Our initial studies were carried out by using several nanoparticles including Mn3O4, CuO, CaO, MgO and Fe3O4 under various reaction conditions. The optimized conditions were obtained when the reactions carried out in the presence of nano-Fe3O4 under solvent-free conditions at 120 °C (Table 1).
Optimization of model reaction by using various catalysts, solvents and amount of magnetic nanoparticles.a
Entry | Catalyst | Catalyst (mol %) | Solvent | Time (min) | Yields (%)b |
1 | Mn3O4 | 20 | EtOH | 120 | 55 |
2 | CuO | 20 | EtOH | 150 | 45 |
3 | CaO | 20 | EtOH | 200 | 30 |
4 | MgO | 20 | EtOH | 180 | 40 |
5 | Fe3O4 | 20 | EtOH | 60 | 75 |
6 | Fe3O4 | 20 | DMF | 140 | 45 |
7 | Fe3O4 | 20 | Toluene | 300 | 25 |
8 | Fe3O4 | 20 | Solvent-free | 25 | 85 |
9 | Fe3O4 | 15 | Solvent-free | 25 | 85 |
10 | Fe3O4 | 10 | Solvent-free | 25 | 85 |
11 | Fe3O4 | 5 | Solvent-free | 35 | 80 |
a Reaction conditions: benzaldehyde (1 mmol), dimedone (2 mmol) and aniline (1 mmol).
b Isolated yields.
In continuation of our research, the model reaction was carried out by using various amounts of Fe3O4 nanoparticles. The optimum amount of nano-Fe3O4 was 10 mol% as shown in Table 1. Increasing this amount did not show any change in yield and time of the reaction (Table 1, Entry 10).
The influence of solvent was studied when the model reaction was performed using Fe3O4 nanoparticles under various solvents and solvent-free conditions (Table 1, Entries 5–8). The best results were obtained under solvent-free conditions (Table 1, Entry 8). As shown in Table 1, the time of reaction was significantly decreased, but the yield of product formation increased in comparison with solvent conditions.
We next used several kinds of aldehydes and amines to investigate their three-component reactions under the optimal reaction conditions (Scheme 1, Table 2). As shown in Table 2, aldehydes containing either electron-withdrawing or electron- releasing groups gave the corresponding 1,8-dioxo-decahydroacridines in high yields. In addition, it was specifically considerable that aryl aldehydes bearing electron-withdrawing groups such as NO2 and Cl reacted very smoothly in short reaction times and higher yields (Table 2, Entries 8,9) while electron-releasing substitutions such as OMe and Me (Table 2, Entries 4, 5) showed less reactivity in the cyclization reaction. As shown in Table 2, these condensation reactions also proceed suitably when different aromatic amines were used in the synthesis of 1,8-dioxo-9-aryl-10-aryl-decahydroacridine derivatives. In this case, we found that aromatic amines containing electron-donating substituents such as OMe and Me (Table 2, Entries 11, 14) afforded the desired products in short reaction times and high yields.
One-pot synthesis of 1,8-dioxo-decahydroacridines catalyzed by nano-Fe3O4.a
Entry | Ar | Ar′ | Product | Time (min) | Yieldb | m.p (°C) | Lit. m.p (°C) |
1 | Ph | Ph | 4a | 25 | 85 | 254–255 | (254–256)[22] |
2 | o-MeC6H4 | Ph | 4b | 40 | 75 | 225–227 | – |
3 | m-MeC6H4 | Ph | 4c | 30 | 80 | 208–210 | – |
4 | p-MeC6H4 | Ph | 4d | 35 | 80 | 260–262 | (260–263)[30] |
5 | o-OMeC6H4 | Ph | 4e | 50 | 70 | 270–272 | (270–272)[22] |
6 | p-OMeC6H4 | Ph | 4f | 35 | 75 | 219–221 | (220–222)[22] |
7 | m-NO2C6H4 | Ph | 4g | 20 | 88 | 298–299 | (297–299)[22] |
8 | p-NO2C6H4 | Ph | 4h | 15 | 90 | 288–290 | (289–290)[30] |
9 | p-ClC6H4 | Ph | 4i | 15 | 92 | 244–246 | (244–246)[22] |
10 | p-BrC6H4 | Ph | 4j | 20 | 90 | 254–256 | (255–257)[30] |
11 | Ph | p-MeC6H4 | 4k | 20 | 85 | 260–262 | (260–263)[28] |
12 | p-NO2C6H4 | p-MeC6H4 | 4l | 10 | 90 | 272–274 | – |
13 | p-ClC6H4 | p-MeC6H4 | 4m | 12 | 95 | 269–270 | (270–271)[28] |
14 | Ph | p-OMeC6H4 | 4n | 18 | 88 | 215–216 | (215–217)[23] |
15 | p-MeC6H4 | p-OMeC6H4 | 4o | 30 | 80 | 239–241 | (238–240)[28] |
16 | p-CNC6H4 | p-OMeC6H4 | 4p | 15 | 90 | 233–235 | – |
a All the reactions were carried out under solvent-free conditions.
b Isolated yields.
3 Catalyst recovery
After completion of the reaction, the reaction mixture was dissolved in chloroform and then the catalyst was separated magnetically. The magnetic Fe3O4 nanoparticles were washed three to four times with chloroform and methanol and dried at 100 °C for 5 h. The separated catalyst was used several times with a slightly decreased activity as shown in Fig. 4.
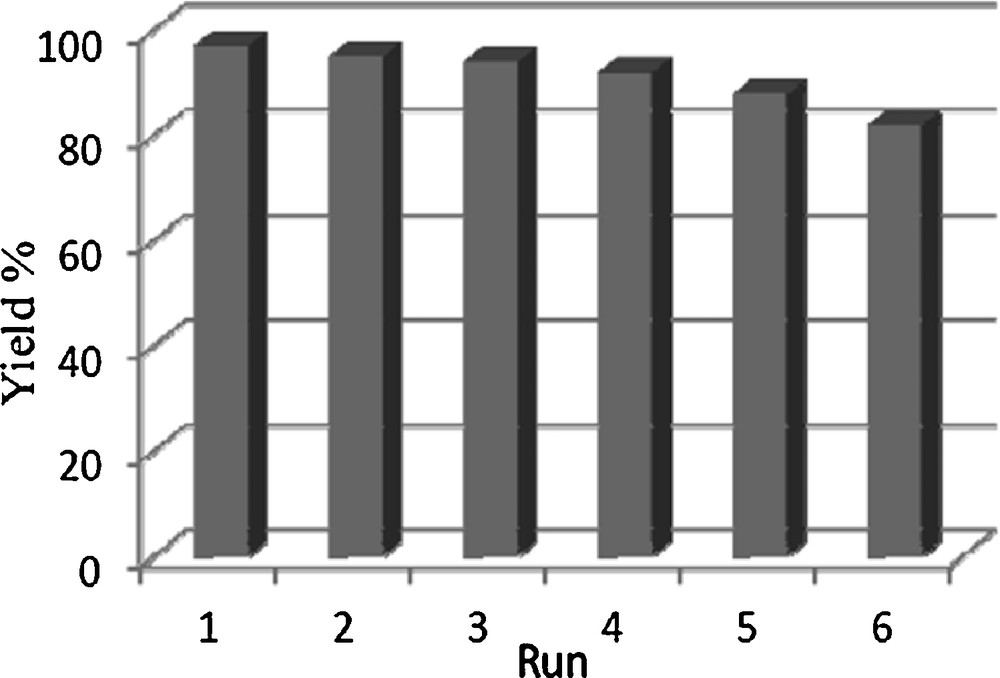
Recoverability of Fe3O4 nanoparticles in the model reaction.
4 Conclusions
In summary, an efficient, mild and green method for the synthesis of 1,8-dioxo-decahydroacridine derivatives has been developed in the presence of Fe3O4 nanoparticles under solvent-free conditions. The products were obtained in excellent yields and the reaction times were significantly short. The present approach demonstrates a simple and appropriate method for the three-component coupling of aldehydes, dimedone and amines in order to synthesis of some heterocyclic compounds via magnetic nanoparticles as novel, effective and simple reusable catalyst.
5 Experimental method
5.1 Materials and techniques
Chemicals were purchased from the Sigma-Aldrich and Merck in high purity. All of the materials were of commercial reagent grade and were used without further purification. All melting points are uncorrected and were determined in capillary tube on Boetius melting point microscope. 1H NMR and 13C NMR spectra were obtained on Bruker 400 MHZ spectrometer with CDCl3 as solvent using tetramethylsilane (TMS) as an internal standard, the chemical shift values are in δ. FT-IR spectrum was recorded on Magna-IR, spectrometer 550 Nicolet in KBr pellets in the range of 400–4000 cm−1. The elemental analyses (C, H, N) were obtained from a Carlo ERBA Model EA 1108 analyzer. The N2 adsorption/desorption analysis (BET) was performed at –196 °C using an automated gas adsorption analyzer (Tristar 3000, Micromeritics). Powder X-ray diffraction (XRD) was carried out on a Philips diffractometer of X’pert Company with monochromatized Ag Kα radiation (λ = 1.5406 Å). Microscopic morphology of products was visualized by SEM (LEO 1455VP). The mass spectra were recorded on a Joel D-30 instrument at an ionization potential of 70 eV.
5.2 Preparation of Fe3O4 nanoparticles
To a solution of FeCl2.4H2O (2.5 g) and FeCl3.6H2O (6 g) in 30 mL deionized water was added dropwise 1.0 mL of concentrated hydrochloric acid at room temperature. The solution was added in to 300 mL of 1.5 mol L−1 NaOH and then the solution was stirred vigorously at 70 °C until precipitation. Afterwards, the prepared magnetic nanoparticles were separated magnetically, washed with deionized water and then dried.
5.3 General procedure for the synthesis of 1,8-dioxo-decahydroacridine (4a-p)
A mixture of aldehyde (1 mmol), dimedone (2 mmol), aromatic amine (1 mmol) and Fe3O4 nanoparticles (0.02 g, 0.1 mmol, 10 mol%) in a round bottom flask was heated with stirring in the oil bath at 120 °C for appropriate times. During the procedure, the reaction was monitored by Thin Layer Chromatography (TLC). Upon completion, the reaction mixture was cooled to room temperature and the reaction mixture was dissolved in chloroform. The catalyst was insoluble in CHCl3 and separated magnetically. The solvent was evaporated and the solid was obtained recrystallized from ethanol to afford the pure acridines.
5.4 Spectral data of new compounds
3,3,6,6-Tetramethyl-9-(2-methylphenyl)-10-phenyl-1,2,3,4,5,6,7,8,9,10-decahydroacridine-1,8-dione (4b). Yellow powder; IR (KBr) (vmax/cm−1): 2952, 2875, 1644, 1572, 1366, 1224, 845; 1H NMR (400 MHz, CDCl3): δ 0.81 (s, 6H, 2 × CH3), 0.95 (s, 6H, 2 × CH3), 1.79–1.84 (d, J = 16 Hz, 2H, 2 × CH), 2.05–2.09 (d, J = 16.5 Hz, 2H, 2 × CH), 2.12–2.16 (d, J = 16.5 Hz, 2H, 2 × CH), 2.18–2.22 (d, J = 16 Hz, 2H, 2 × CH), 2.32 (s, 3H, CH3), 5.25 (s, 1H, CH), 7.13–7.31 (m, 5H, ArH), 7.55–7.57 (m, 4H, ArH); 13C NMR (100 MHz, CDCl3): δ 22.2, 26.9, 29.7, 32.3, 32.4, 41.5, 51.3, 112.1, 114.8, 127.4, 128.8, 129.4, 130.1, 131.6, 135.2, 139.1, 144.8, 150.1, 195.7. Anal. Calcd. For C30H33NO2: C 81.97, H 7.57, N 3.19. Found C 81.82, H 7.65, N 3.28. MS (EI) (m/z): 439 (M+).
3,3,6,6-Tetramethyl-9-(3-methylphenyl)-10-phenyl-1,2,3,4,5,6,7,8,9,10-decahydroacridine-1,8-dione (4c). Yellow powder; IR (KBr) (vmax/cm−1): 2954, 2873, 1642, 1574, 1362, 1221, 842; 1H NMR (400 MHz, CDCl3): δ 0.79 (s, 6H, 2 × CH3), 0.94 (s, 6H, 2 × CH3), 1.78–1.85 (d, J = 16.2 Hz, 2H, 2 × CH), 2.05–2.08 (d, J = 16.6 Hz, 2H, 2 × CH), 2.13–2.17 (d, J = 16.6 Hz, 2H, 2 × CH), 2.20–2.46 (d, J = 16.2 Hz, 2H, 2 × CH), 2.31 (s, 3H, CH3), 5.24 (s, 1H, CH), 6.91(s, 1H, ArH), 7.13–7.26 (m, 3H, ArH), 7.44–7.61 (m, 5H, ArH); 13C NMR (100 MHz, CDCl3): δ 22.1, 26.8, 29.8, 32.3, 32.3, 41.5, 51.4, 112.1, 115.1, 127.4, 128.7, 129.8, 131.1, 132.1, 135.7, 138.9, 145.1, 150.1, 195.7. Anal. Calcd. For C30H33NO2: C 81.97, H 7.57, N 3.19. Found C 82.08, H 7.48, N 3.11. MS (EI) (m/z): 439 (M+).
3,3,6,6-Tetramethyl-9-(4-nitrophenyl)-10-(p-tolylphenyl)-1,2,3,4,5,6,7,8,9,10 decahydroacridine-1,8-dione (4l). Yellow powder; IR (KBr) (vmax/cm−1): 2956, 2873, 1639, 1576, 1514, 1359, 1222, 863; 1H NMR (400 MHz, CDCl3): δ 0.92 (s, 6H, 2 × CH3), 1.12 (s, 6H, 2 × CH3), 1.84–1.88 (d, J = 17.6 Hz, 2H, 2 × CH), 2.07–2.13 (m, 4H, 4 × CH), 2.23–2.28 (d, J = 17.6 Hz, 2H, 2 × CH), 2.50 (s, 3H, CH3), 5.34 (s, 1H, CH), 7.09-7.11 (d, J = 7.2 Hz, 2H, ArH), 7.37–7.39 (d, J = 7.2 Hz, 2H, ArH), 7.59–7.61 (d, J = 8.1 Hz, 2H, ArH), 8.12-8.14 (d, J = 8.1 Hz, 2H, ArH); 13C NMR (100 MHz, CDCl3): δ 23.4, 26.7, 29.7, 32.4, 32.9, 41.7, 50.1, 113.5, 116.5, 123.5, 128.8, 129.7, 138.2, 146.2, 148.1, 150.4, 152.9, 195.7. Anal. Calcd. For C30H32N2O4: C 74.36, H 6.66, N 5.78. Found C 74.22, H 6.78, N 5.89. MS (EI) (m/z): 484 (M+).
3,3,6,6-Tetramethyl-9-(4-cyanophenyl)-10-(4-methoxyphenyl)-1,2,3,4,5,6,7,8,9,10-decahydroacridine-1,8-dione (4p). Yellow powder; IR (KBr) (vmax/cm−1): 2957, 2875, 2224, 1640, 1574, 1510, 1364, 1221, 849; 1H NMR (400 MHz, CDCl3): δ 0.79 (s, 6H, 2 × CH3), 0.96 (s, 6H, 2 × CH3), 1.84–1.89 (d, J = 17.6 Hz, 2H, 2 × CH), 2.06–2.10 (d, J = 17.6 Hz, 2H, 2 × CH), 2.13–2.22 (m, 4H, 4 × CH), 3.93 (s, 3H, OCH3), 5.28 (s, 1H, CH), 7.06–7.08(d, J = 8.4 Hz, 2H, ArH), 7.11–7.13 (d, J = 8.4 Hz, 2H, ArH), 7.54 (m, 4H, ArH); 13C NMR (100 MHz, CDCl3): δ 26.7, 29.7, 32.3, 33.7, 41.8, 50.1, 55.7, 109.5, 113.5, 115.1, 119.3, 128.8, 131.1, 132.0, 150.9, 151.6, 160.1, 195.7. Anal. Calcd. For C31H32N2O3: C 77.47, H 6.71, N 5.83. Found C 77.32, H 6.65, N 5.99. MS (EI) (m/z): 480 (M+).
Acknowledgements
The authors are grateful to University of Kashan for supporting this work by Grant NO: 65384.