1 Introduction
Multicomponent reactions (MCRs) have drawn great interest enjoying an outstanding status in modern organic synthesis and medicinal chemistry because they are one-pot processes bringing together three or more components and show high atom economy and high selectivity [1]. Such processes are of great interest in diversity-oriented synthesis, especially to generate compound libraries for screening purposes. The Hantzsch reaction [2], and its products 1,4-dihydropyridines (DHP) have attracted immense attention of synthetic chemists due to their pharmacological properties [3,4].
In addition, the dihydropyridine unit has been widely employed as a hydride source for reductive amination [5]. Despite the potential importance of 1,4-dihydropyridyl compounds from a pharmaceutical, industrial, and synthetic point of view [6–8], polyhydroquinoline compounds not only attract the attention of chemists to synthesize but also represent an interesting research challenge. The classical methods involve the three-component condensation of an aldehyde with ethyl acetoacetate, and ammonia in acetic acid or in refluxing alcohol [9–11]. However, these methods suffer from drawbacks such as a long reaction time, use of large quantities of organic solvents, lower product yields or harsh refluxing conditions. In recent years, several new efficient methods for the synthesis of polyhydroquinoline derivatives, which include the use of microwaves, [12] autoclave, [13] ionic liquids, [14] iodine, [15] metal triflate, [16] cerric ammonium nitrate, [17] l-proline, [18] PTSA-SDS, [19] BINOL-phosphoric acid, [20] Hf(NPf2)4/C10F18, [21] and TFE [22], ionic liquids [23], the use of microwaves [24], refluxing at high temperature [25], grinding [26], Bu4NHSO4 [27], HY-zeolite [28], L-proline [29], silica-supported acids [30], TMSCl–NaI [31], boronic acids [32], metal triflates [16], cerric ammonium nitrate (CAN) [33], baker's yeast [34], and p-TSA [35], or ZnO-nanoparticles [36].
In continuation of our studies in developing cheap and environmentally benign methodologies for organic synthesis, we turned our attention towards the synthesis of hexahydroquinoline derivatives. The non-ionic surfactant Triton X-100 (TR) is one of the most commonly used detergents in biochemistry as solubilizer with a wide range of applications to biological systems [37]. Solubilization of lipid membranes triggered by Triton X-100 is a well-described phenomenon. It is also used as an emulsifier, and complexing agent in both aqueous and non-aqueous media. Non-ionic surfactants have the tendency to adsorb at interfaces and to form micelles beyond their critical micelle concentration (CMC) similar to ionic surfactants [38]. However, the advantage of non-ionic surfactants (Triton X-100) is the absence of the electrical double layer as formed by the ionic surfactants. Consequently, non-ionic surfactants are desirable model adsorbents for interfacial processes. Therefore, we decided to exploit these properties of non-ionic surfactant for organic reaction. We report herein a practical synthesis of hexahydroquinoline derivatives in Triton X-100 aqueous micellar media at room temperature.
2 Results and discussion
We carried out the four-component coupling reaction of dimedone, aldehyde, acetoacetic ester, and ammonium acetate using Triton X-100 in water solvent (Scheme 1).
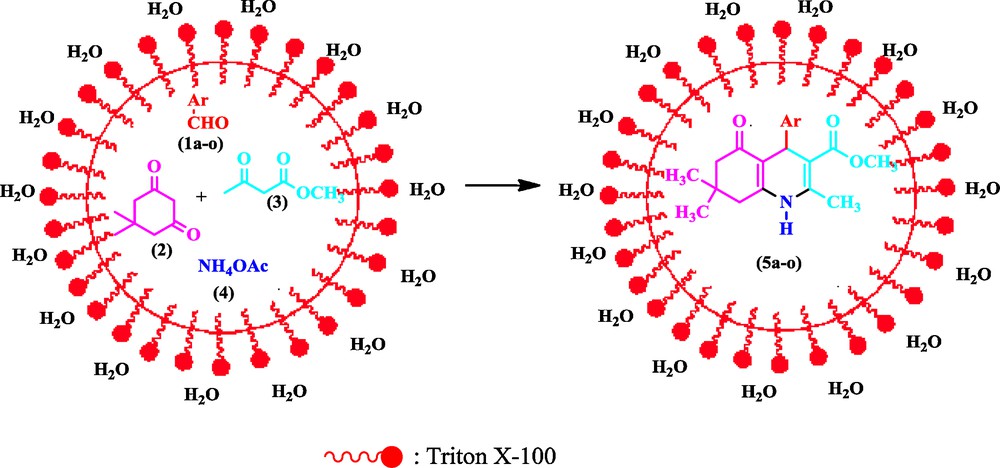
Triton X-100 catalyzed condensation of dimedone, aldehydes, methyl acetoacetate and ammonium acetate.
Initially, we screened various conditions for the one-pot four-component reaction of benzaldehyde, dimedone, acetoacetic ester, and ammonium acetate as a model reaction in methanol at room temperature. In polar solvents like methanol, ethanol, DMF, tetrahydrfuran, acetonitrile and DMSO product 5a was obtained in higher yields. However, in a non-polar solvent such as dichloromethane, product 5a were obtained in poor yield. When the reaction was carried out under solvent free condition at room temperature and 60 °C, we found 21% and 53% product 5a. Also, when benzaldehyde, dimedone, acetoacetic ester, and ammonium acetate were stirred at room temperature without water, using water as solvent and Triton X-100 (20 mol %) as a surfactant the yield of 5a was improved (entry 11, 12, Table 1). However, it was found that the amount of Triton X-100 (C14H22(C2H4O)n) where n = 9–10, influenced the yield of 5a.
Effect of solvents on synthesis of methyl 2,7,7-trimethyl-5-oxo-4-phenyl-1,4,5,6,7,8-hexahydroquinoline-3-carboxylate 5aa.
Yield (%)b | Solvents | Entry |
33 | MeOH | 1 |
31 | EtOH | 2 |
25 | THF | 3 |
12 | DCM | 4 |
26 | ACN | 5 |
30 | DMSO | 6 |
27 | DMF | 7 |
9 | Waterc | 8 |
38 | MeOHc | 9 |
21 | Neat | 10 |
53d | Neat | 11 |
65 | Triton X-100e | 11 |
97 | Water/Triton X-100f | 12 |
a The reaction was conducted with benzaldehyde (2 mmol), dimedone (2 mmol), acetoacetic ester (2 mmol) and ammonium acetate (2 mmol) at room temperature.
b Isolated yield.
c Reaction was carried out with heating.
d Reaction carried out at 60 °C
e Triton X-100 (20 mol %).
f Triton X-100 (20 mol %) in water (5 mL).
We studied the role of catalyst concentration on the model reaction 5a. We have varied the catalyst concentration to 0, 1, 5, 10, 20 and 25 mol %. The result revealed that, when the reaction was carried out in the presence of 0, 1, 5 and 10 mol % of catalyst it gave lower yield of product even after prolonged reaction time. At the same time when the concentration of catalyst was 20 mol % we achieved an excellent yield of product in a short span. Even after increasing the catalyst concentration above 20 mol % the yield of the products did not improve. So, it is established that the 20 mol % of catalyst is sufficient to catalyst and bring it to completion. The results are listed in Table 2.
One-pot synthesis of methyl 2,7,7-trimethyl-5-oxo-4-phenyl-1,4,5,6,7,8-hexahydroquinoline-3-carboxylate 5a in presence of various Triton X-100 surfactant concentrationa.
Entry | Catalyst (mol %) | Time (min) | Yield (%)b |
1 | – | 60 | 5 |
2 | 1 | 60 | 10 |
3 | 5 | 60 | 38 |
4 | 10 | 60 | 55 |
5 | 20 | 60 | 97 |
6 | 25 | 60 | 88 |
a Condition: the reaction was performed by using 2 mmol of 1a, 2 mmol of 2, 2 mmol of 3 and 2 mmol of 4 in the presence of Triton X-100 surfactant in 5 mL water at rt.
b Isolated yields.
In order to determine the scope of this reaction, we have synthesized differently substituted hexahydroquinoline by varying differently substituted aldehydes (1a–o) including both electron-donating and electron-withdrawing groups. It is observed that the reaction gave good yields of products with faster reaction rate when the aldehyde bearing electron-withdrawing group is used compared to the aldehydes with electron-donating groups. The corresponding results are tabulated in Table 3.
Synthesis of hexahydroquinoline derivatives via Hantzsch reaction in Triton X-100 aqueous micellar media (20 mol %).
Entry | Ar | Product | Time (min) | Yield (%)a |
1 | C6H5 | 5a | 60 | 97 |
2 | 4-BrC6H4 | 5b | 90 | 94 |
3 | 2-BrC6H4 | 5c | 100 | 93 |
4 | 4-CH3C6H4 | 5d | 120 | 92 |
5 | 2-CH3C6H4 | 5e | 130 | 91 |
6 | 4-CH3OC6H4 | 5f | 120 | 93 |
7 | 2-CH3OC6H4 | 5g | 110 | 92 |
8 | 4-NO2C6H4 | 5h | 30 | 98 |
9 | 2-NO2C6H4 | 5i | 35 | 96 |
10 | 4-FC6H4 | 5j | 25 | 98 |
11 | 2-FC6H4 | 5k | 30 | 98 |
12 | 4-CF3C6H4 | 5l | 30 | 97 |
13 | 4-C6H5C6H4 | 5m | 30 | 90 |
14 | 1-Naphthyl | 5n | 65 | 89 |
15 | 2-Furyl | 5o | 85 | 87 |
a Isolated yields.
3 Conclusion
In summary, we have described herein an efficient methodology for Hantzsch reaction using various electronically and structurally divergent aldehydes to give the product in excellent isolated yields. In contrast to the existing methods using potentially hazardous catalysts/additives, this new method offers the following competitive advantages:
- • avoiding the use of any base, metal or Lewis acid catalyst;
- • short reaction time;
- • ease of product isolation/purification by non-aqueous work-up;
- • high chemo selectivity;
- • no side reaction;
- • low costs and simplicity in process and handling.
4 Experimental
4.1 Material and technique
Aldehydes were distilled before use. Melting points were determined using a Linkman HF591 heating stage, used in conjunction with a TC92 controller, and re-uncorrected. NMR spectra were recorded using either a Brucker DRX500 machine at room temperature. 1H and 13C NMR spectra were measured using deuterochloroform as solvent and chemical shifts were measured relative to residual solvent or CFCl3 as an internal standard for 19FNMR and are expressed in parts per million (δ). Mass spectra were obtained using a MicroMass LCT machine in ES or EI mode. Infrared spectra were measured on a Perkin Elmer Paragon 100 FT-IR spectrometer.
4.2 General procedure for the synthesis of hexahydroquinoline derivatives (5a–o)
A mixture of aromatic aldehyde (1a–o), dimedone (2) (0.01 mol), methyl acetoacetate (3) (0.01 mol) and ammonium acetate (4) (0.01 mol) were taken in a mixture of Triton X-100 (20 mol %) and water (2 mL) in a round-bottomed flask. The resulting mixture was vigorously stirred at room temperature until completion of the reaction as monitored by thin-layer chromatography (TLC). After completion, the reaction mixture was poured onto crushed ice (70 g) with vigorous stirring. The precipitate obtained was filtered, washed with water, dried, and purified by recrystallization from ethanol afforded pure products 5a–o. Structures of the all the products were confirmed by analytical and spectral data.
4.3 Spectral data of new compounds
4.3.1 Methyl 2,7,7-trimethyl-5-oxo-4-phenyl-1,4,5,6,7,8-hexahydroquinoline-3-carboxylate (5a)
0.315 g (97%); white crystal; mp 212–214 °C; IR (KBr) (υmax/cm−1): 3513, 3098, 2989, 2893, 1721, 1703, 1556, 1445, 798, 745; 1H NMR (CDCl3, 500 MHz) δ: 0.92 (s, 3H, CH3), 1.07 (s, 3H, CH3), 2.28–2.31 (m, 4H, 2 × CH2), 2.39 (s, 3H, CH3), 3.60 (s, 3H, OCH3), 5.06 (s, 1H, CH), 6.21 (s, 1H, NH), 7.10 (t, 1H, Ar-H), 7.20 (t, 2H, Ar-H), 7.29 (d, 2H, Ar-H). 13C NMR (CDCl3, 125 MHz) δ: 19.45, 26.30, 26.33, 26.60, 27.11, 29.33, 32.12, 36.25, 41.13, 50.44, 51.04, 106.06, 112.18, 117.09, 127.78, 127.99, 143.58, 146.63, 167.79, 195.38; MS (EI), m/z (%) = 326 (M+, 25), 327 (5), 248 (37); HRMS (EI) Found: M+, 325.1693, C20H23NO3 requires M+, 325.1702; Anal Calcd for C20H23NO3: C, 73.82; H, 7.12; N, 4.30. Found: C, 73.76; H, 7.28; N, 4.43.
4.3.2 Methyl 4-(2-bromophenyl)-2,7,7-trimethyl-5-oxo-1,4,5,6,7,8-hexahydroquinoline-3-carboxylate (5c)
0.379 g (94%); yellow solid; mp 251–252 °C; IR (KBr) (υmax/cm−1): 3539, 3109, 2978, 2798, 1731, 1709, 1545, 1465, 787, 732. 1H NMR (CDCl3, 500 MHz), δ: ppm, 0.95 (s, 3H, CH3), 1.11 (s, 3H, CH3), 2.32–2.36 (m, 4H, 2 × CH2), 2.78 (s, 3H, CH3), 3.87 (s, 3H, OCH3), 5.10 (s, 1H, CH), 6.33 (s, 1H, NH), 7.34–7.73 (m, 3H, Ar-H), 7.89 (d, J = 7.5 Hz, 1H, Ar-H); 13C NMR (CDCl3, 125 MHz) δ: 20.21, 27.31, 27.78, 28.66, 29.01, 30.13, 33.14, 38.25, 44.63, 52.44, 55.04, 103.26, 110.88, 118.69, 129.08, 130.93, 141.78, 145.62, 165.99, 193.68; MS (EI), m/z (%) = 407 (M+, 2), 405 (20), 248 (35); HRMS (EI) Found: M+, 403.0812, C20H22BrNO3 requires M+, 403.0801; Anal Calcd for C20H22BrNO3 C, 59.42; H, 5.48; N, 3.46. Found: C, 59.76; H, 5.28; N, 3.65.
4.3.3 Methyl 2,7,7-trimethyl-5-oxo-4-p-tolyl-1,4,5,6,7,8-hexahydroquinoline-3-carboxylate (5d)
0.311 g (92%); pale yellow solid; mp 283–285 °C; IR (KBr) (υmax/cm−1): 3496, 3069, 2893, 2798, 1718, 1709, 1525, 1429, 767, 737. 1H NMR (CDCl3, 500 MHz) δ: 1.07 (s, 3H, CH3), 1.21 (s, 3H, CH3), 2.18–2.30 (m, 4H, 2 × CH2), 2.36 (s, 3H, CH3), 2.46 (s, 3H, CH3), 3.79 (s, 3H, OCH3), 5.21 (s, 1H, CH), 6.11 (s, 1H, NH), 7.33 (d, J = 7.5 Hz, 2H, Ar-H), 7.57 (d, J = 7.5 Hz, 2H, Ar-H). 13C NMR (CDCl3, 125 MHz) δ: 21.15, 27.33, 26.33, 28.60, 28.91, 31.03, 33.42, 34.75, 40.83, 45.48, 50.54, 108.66, 114.98, 131.29, 134.58, 138.79, 143.68, 144.63, 166.89, 192.48. MS (EI), m/z (%) = 339 (M+, 15), 324 (5), 248 (57); HRMS (EI) Found: M+, 340.1008, C21H25NO3 requires M+, 339.1809; Anal Calcd for C21H25NO3: C, 74.31; H, 7.42; N, 4.13. Found: C, 74.47; H, 7.38; N, 4.09.
4.3.4 Methyl 4-(4-fluorophenyl)-2,7,7-trimethyl-5-oxo-1,4,5,6,7,8-hexahydroquinoline-3-carboxylate (5j)
0.336 g (98%); white solid; mp 182–184 °C; IR (KBr) (υmax/cm−1): 3534, 3075, 2968, 2859, 1730, 1711, 1543, 1438, 778, 735; 1H NMR (CDCl3, 500 MHz) δ: 0.92 (s, 3H, CH3), 1.07 (s, 3H, CH3), 2.28–2.31 (m, 4H, 2 × CH2), 2.39 (s, 3H, CH3), 3.60 (s, 3H, OCH3), 5.06 (s, 1H, CH), 6.21 (s, 1H, NH), 7.20 (t, J = 8.0 Hz, 2H, Ar-H), 7.29 (d, J = 8.0 Hz, 2H, Ar-H); 13C NMR (CDCl3, 125 MHz) δ: 21.46, 23.78, 25.34, 27.80, 28.81, 30.47, 31.14, 38.55, 43.14, 48.94, 50.54, 115.66, 120.68, 125.49, 129.10 (d, 1JCF = 250.3 Hz), 132.39, 140.53, 144.67, 163.59, 192.35; 19F NMR (CDCl3, 470 MHz) δ: −60.1. MS (EI), m/z (%) = 343 (M+, 5), 324 (5), 248 (25); HRMS (EI) Found: M+, 343.1653; C20H22FNO3: requires M+, 343.1602; Anal Calcd for C20H22FNO3: C, 69.95; H, 6.46; N, 4.08. Found: C, 70.16; H, 6.58; N, 4.03.
4.3.5 Methyl 2,7,7-trimethyl-5-oxo-4-(4-(trifluoromethyl)phenyl)-1,4,5,6,7,8-hexahydroquinoline-3-carboxylate (5l)
0.381 g (97%); yellow solid; mp 248–250 °C; IR (KBr) (υmax/cm−1): 3485, 3089, 2968, 2865, 1723, 1711, 1556, 1476, 779, 738; 1H NMR (CDCl3, 500 MHz), δ: 1.19 (s, 3H, CH3), 1.36 (s, 3H, CH3), 2.47–2.65 (m, 4H, 2 × CH2), 2.85 (s, 3H, CH3), 3.84 (s, 3H, OCH3), 5.06 (s, 1H, CH), 6.30 (s, 1H, NH), 7.54 (t, J = 8.0 Hz, 2H, Ar-H), 7.79 (d, J = 8.0 Hz, 2H, Ar-H); 13C NMR (CDCl3, 125 MHz) δ: 22.54, 25.36, 26.26, 27.12, 28.16, 31.32, 32.56, 35.23, 40.18, 45.44, 53.54, 107.66, 111.28, 121.32, 131.09, 132.56, 134.89, 134.33 (q, 1JCF = 252.3 Hz, CF3), 143.64, 164.73, 194.33; 19F NMR (CDCl3, 470 MHz) δ: −110.5. MS (EI), m/z (%) = 393 (M+, 7), 324 (5), 248 (54); HRMS (EI) Found: M+, 393.1601. C21H22F3NO3 requires M+, 393.1612; Anal Calcd for C21H22F3NO3: C, 64.11; H, 5.64; N, 3.56. Found: C, 63.96; H, 5.48; N, 3.43.
4.3.6 Methyl 4-(biphenyl-4-yl)-2,7,7-trimethyl-5-oxo-1,4,5,6,7,8-hexahydroquinoline-3-carboxylate (5m)
0.360 g (90%); pale yellow solid; mp 267–269 °C; IR (KBr) (υmax/cm−1): 3531, 3079, 2976, 2881, 1731, 1710, 1536, 1443, 776, 734; 1H NMR (CDCl3, 500 MHz) δ: 1.07 (s, 3H, CH3), 1.12 (s, 3H, CH3), 2.34–2.56 (m, 4H, 2 × CH2), 2.67 (s, 3H, CH3), 3.87 (s, 3H, OCH3), 5.23 (s, 1H, CH), 6.35 (s, 1H, NH), 7.17 (d, J = 7.5 Hz, 2H, Ar-H), 7.24 (d, J = 8.0 Hz, 2H, Ar-H), 7.14 (d, J = 7.5 Hz, 2H, Ar-H), 7.54 (d, J = 8.0 Hz, 2H, Ar-H), 8.02 (m, 1H, Ar-H); 13C NMR (CDCl3, 125 MHz) δ: 19.45, 26.30, 26.33, 26.60, 27.11, 29.33, 32.12, 36.25, 41.13, 50.44, 51.04, 106.06, 112.18, 114.23, 116.65, 116.98, 118.76, 119.98, 121.65, 124.65, 129.09, 127.78, 127.99, 143.58, 146.63, 167.79, 195.38; MS (EI), m/z (%) = 401 (M+, 12), 274 (5), 215 (32); HRMS (EI) Found: M+, 401.2102, C26H27NO3 requires M+, 401.2014; Anal Calcd for C26H27NO3: C, 77.78; H, 6.78; N, 3.49. Found: C, 77.85; H, 6.84; N, 3.56.
4.3.7 Methyl 2,7,7-trimethyl-4-(naphthalen-1-yl)-5-oxo-1,4,5,6,7,8-hexahydroquinoline-3-carboxylate (5n)
0.337 g (89%); pale yellow solid; mp 263–265 °C; IR (KBr) (υmax/cm−1): 3523, 3080, 2979, 2879, 1736, 1712, 1539, 1438, 789, 747; 1H NMR (CDCl3, 500 MHz) δ: 0.93 (s, 3H, CH3), 1.12 (s, 3H, CH3), 2.31–2.45 (m, 4H, 2 × CH2), 2.54 (s, 3H, CH3), 3.72 (s, 3H, OCH3), 5.12 (s, 1H, CH), 6.17 (s, 1H, NH), 7.38–7.67 (m, 7H, Ar-H); 13C NMR (CDCl3, 125 MHz) δ: 19.06, 23.32, 25.34, 25.66, 27.67, 28.93, 33.14, 38.28, 47.13, 55.44, 58.98, 108.76, 119.98, 120.76, 121.67, 123.65, 126.89, 128.09, 129.78, 129.99, 139.57, 143.33, 162.72, 192.28; MS (EI), m/z (%) = 326 (M+, 25), 327 (5), 248 (37); HRMS (EI) Found: M+, 375.1903, C24H25NO3 requires M+, 375.1812; Anal Calcd for C24H25NO3: C, 76.77; H, 6.71; N, 3.73. Found: C, 77.04; H, 6.68; N, 3.80.
Acknowledgment
The authors are grateful to Payame Noor University for supporting this work.