1 Introduction
Polyaniline (PANI) is a conducting polymer that has received immense attention in recent years owing to its physical, chemical and biological properties [1]. Therefore, the PANI-containing polymeric nanocomposites (PNCs) are widely being used for the fabrication and development of bioelectronics, optoelectronics, and sensor-based devices [2,3]. Among them, particularly, the PNCs of metal oxides such as cobalt iron oxide (CoFe2O4) nanoparticles (NPs) have been studied extensively to understand their unique physicochemical properties, not only for scientific research, but also for technological applications [4]. CoFe2O4 belongs to the family of spinel-type ferrites and is one of the most important ferrites, with high coercivity, moderate magnetization, high magnetocrystalline anisotropy, chemical stability, and mechanical hardness [5]. These CoFe2O4-based PNCs frequently exhibit unexpected hybrid properties derived from both components and have great potential applications in the areas of electronics, photonics, catalysis, etc. [6].
Magnetic NPs of iron oxides and their hybrid forms represent the most suitable candidates for the preparation of magnetic nanocomposites owing to their application-convenient magnetic (e.g., superparamagnetism) and biochemical (e.g., non-toxicity, biocompatibility, biodegradability) properties and low price. These oxides that can play the role of nano-antimicrobial agents are believed to be non-toxic, and some of them even contain mineral elements essential to the human body [7]. Moreover, biomedical applications of polyaniline/CoFe2O4 nanocomposite have also been reported in recent years owing to its suitable magnetic behaviour at room temperature for MRI contrast enhancement and hyperthermia treatments [8]. Although conventional antibacterial agents have several disadvantages, i.e., toxicity to the microbiota and sensitivity towards the temperatures and pressures [9], therefore, the interest in metal-oxide-based nano-antimicrobial agents are rising over the years [10,11]. These metal-oxide-based nano-antimicrobial agents exhibited strong antimicrobial activity at low concentrations, with high stability in extreme conditions [12,13]. The suggested mechanism for the antimicrobial activity of metal-oxide-based NPs is based mainly on the production of reactive oxygen species (ROS) from the catalysis of water [14]. Although many controversies have been raised on the mechanistic aspects of the antimicrobial activity of the metal oxide NPs, there is copious evidence that metal-oxide-based NPs increase oxidative stress through the generation of reactive oxygen species (ROS) that include predominantly hydroxyl radicals (•OH) and singlet oxygen (1O2). These ROS mainly contribute to the antimicrobial activity of metal-oxide-based NPs [14,15]. Other antimicrobial mechanisms involving metal-oxide-based NPs, like cytoplasmic membrane disruption and electrostatic binding of metal oxide NPs to the cell surface of the microbial pathogen, have been reported [16–18]. However, recently the production of ROS governed by the electronic band gap property of metal-oxide-based NPs has been considered as triggering the actual mechanism [14]. The energy band gap property of metal-oxide-based NPs are influenced by various physical and chemical parameters [19]. Therefore, a fundamental understanding of the energy band gap property of metal-oxide-based NPs becomes crucial to the tailoring of novel nano-antimicrobial agents in an economic way.
Recent preliminary studies on CoFe2O4 NPs have demonstrated that they may be appropriate antimicrobial agents to control and manage human microbial pathogens [20,21]. Ferrite NPs exhibit an intrinsic peroxidase-like activity by generating ROS via the Fenton reaction [22]. CoFe2O4 NPs interacts with the microbial cell surface and elevates the oxidative stress that disrupts the integrity of the cell wall/membrane and damage the biological macromolecules [23]. Although NPs coated with polymers exhibited stronger antimicrobial activity in comparison to the bare [24], extensive work has been done in recent years to develop promising nanocomposite antibacterial agents based on synthetic materials or biopolymers. These polymers possess an intrinsic antibacterial activity through (i) coating or adsorption of an antibacterial agent onto the polymer surface; (ii) grafting/fabrication of an antibacterial agent in the polymer via ionic or covalent bonding, or (iii) direct incorporation of an antibacterial agent into the polymer during in situ synthesis [25,26]. Among them, conducting polymer polyaniline (PANI) has inherently a broad spectrum of antibacterial and antifungal properties. Thus, the PANI has been the subject of considerable attention due to its potential in biomedical applications [27,28]. These findings motivated us to use PANI/CoFe2O4 composite as an anticandidal agent.
Over the past years, researches have been carried out on the assessment of the antimicrobial activity of NPs and nanomaterials. However, research focused on the antimicrobial properties of NPs and nanomaterials were confined to the prokaryotic pathogens in comparison to the eukaryotic pathogens. Therefore, in this study, we have chosen Candida albicans, an eukaryotic human pathogen, for assessment of the anticandidal properties of PANI/CoFe2O4 nanocomposite. C. albicans is amongst the most common fungal causative agent in superficial and deep-seated candidiasis, depending on the patient's immune system status [29]. The brutality of antifungal drugs in pharmacotherapy has led to the development of widespread multi-drug resistance (MDR) in C. albicans [30]. The failure of antifungal drugs to control infection makes it crucial to find alternatives to currently available drugs. Unfortunately, most of these antifungal drugs chemical in nature and bulk form that are being investigated are too reactive and are unsuitable for the treatment of C. albicans infections in humans [31]. With the continuous development of MDR in C. albicans, the search for new medical treatments beyond conventional antifungal drugs has become a key aim of public health research [32]. Possible innovative strategies encompass the inhibition of C. albicans growth with the use of NPs and nanomaterials [33]. There is thus a need for the identification of a novel class of PNCs that can be able to inhibit efficiently C. albicans growth. Identification of such metal-oxide-based or hybrid nano-antimicrobial agents could present us with new opportunities for the development of novel non-antibiotic drugs for treating C. albicans diseases [30,32,33]. The anticandidal activity of PANI/CoFe2O4 against C. albicans has not been studied yet. Therefore, in this study, we have synthesized a stable and surface-protected PANI/CoFe2O4 nanocomposite (denoted as “cfPNCs”) using a conducting polymer and investigated its anticandidal activity against C. albicans 077.
2 Materials and methods
2.1 Materials
All reagents were of analytical grade. Cobalt nitrate, iron nitrate, ammonium peroxydisulfate, citric acid, N-methyl-2-pyrrolidone (NMP) and hydrochloric acid were purchased from SRL, India. The nutrient media, Sabouraud's dextrose (SD) broth and agar for the C. albicans 077 were obtained from the HiMedia Laboratories, Mumbai, India. Histidine and 2,7-dichlorofluorescin diacetate (DCFH-DA) was purchased from Sigma-Aldrich (St. Louis, Missouri, USA). All other chemicals used were of the highest purity available from commercial sources.
2.2 Nanocomposite materials synthesis
2.2.1 CoFe2O4 NPs synthesis
CoFe2O4 NPs were synthesized by the gel-combustion citrate–nitrate method reported previously [34]. The salts of Co(NO3)3·9H2O and Fe (NO3)3·9H2O (1:1 molar ratio) were dissolved completely in 100 mL of Milli Q water. In this salt mixture, an equal amount of citric acid (1:1 citric acid/nitrate molar ratio) was added, and the suspension was continuously stirred at a constant temperature at about 90 °C. The pH of the suspension was maintained at ∼7.4 to stabilize the nitrate–citrate solution until a high-viscosity wet sol gel was formed. The sol gel was subjected to a high temperature of ∼300 °C in an aerobic atmosphere for combustion. The combustion product was a brown, puffy, porous dry gel; calcination of the powder was carried out at 550 °C for 3 h.
2.2.2 PANI/CoFe2O4 nanocomposite (cfPNCs) synthesis
The cfPNCs was prepared via in situ polymerization of aniline in an aqueous solution [35] containing CoFe2O4 NPs as synthesized (see 2.2.1). Initially, 0.2 M of aniline was dissolved in 1 M HCl and 0.25 g (w/v) of an ultra-sonicated CoFe2O4 NPs aqueous solution was added. The solution was vigorously stirred to keep CoFe2O4 NPs suspended. In this solution, 0.625 M (NH4)2S2O8 was added dropwise to the suspension mixture under constant stirring and refrigerated conditions (∼4 °C) for ∼8 h. The color of the synthesized cfPNCs changed into dark green after polymerization. The resultant cfPNCs was harvested by centrifugation at 5000 rpm for 10 min. The slurry of the cfPNCs thoroughly was washed with 0.1 M HCl, then with MQ water. The final cfPNCs product was dried under vacuum at 60 °C for 24 h, and grounded into powder form. To see the effect of PANI (aniline; 0.1 M, 0.2 M, 0.3 M, 0.4 M, and 0.5 M) on the anticandidal activity, different samples of the cfPNCs were also synthesized.
2.3 PANI/CoFe2O4 nanocomposite (cfPNCs) characterizations
The synthesis of cfPNCs in solution was monitored by measuring the absorbance (A) using UV-vis spectrophotometer (PerkinElmer Life and Analytical Sciences, CT, USA) in the wavelength range of A200 to A800 nm. The CoFe2O4 NPs and cfPNCs dispersed in NMP were stored at 4 °C for 120 days and the A275 nm absorbance was measured regularly to ascertain their stability. The vacuum-dried cfPNCs powder was stored in amber color vials at room temperature under dry and dark conditions until used for further characterization. The X-ray diffraction (XRD) patterns of powdered samples were recorded on a MiniFlex™ II benchtop XRD system (Rigaku Corporation, Tokyo, Japan) operating at 40 kV and at a current of 30 mA with Cu Kα radiation (λ = 1.54 Å). The diffracted intensities were recorded from 20° to 80° 2θ angles. The crystalline size (D) [1] of the cfPNCs NPs in the composite was calculated following the Debye–Scherrer formula:
2.4 PANI/CoFe2O4 nanocomposite (cfPNCs) anticandidal activity
2.4.1 Growth conditions
Clinical isolate C. albicans 077 was obtained from the Department of Microbiology, JN Medical College, Aligarh, U.P., India. The stock culture was maintained on slants of SD agar at 4 °C. The primary culture of the C. albicans 077 was prepared from the stock slant into the SD broth medium and incubated for 48 h (stationary phase, 108 cfu/mL) at 37 °C. The primary culture (∼1 mL) was re-inoculated into the 50-mL fresh SD broth and grown for ∼12 h up to mid-log phase (∼105 cfu/mL) at 37 °C. All experiments were performed from the mid-log phase (∼105 cfu/mL) freshly grown C. albicans 077 culture in triplicates.
2.4.2 Anticandidal activity
2.4.2.1 Agar disc diffusion assay
To assess the zone of inhibition of C. albicans 077 grown by cfPNCs, an agar disc diffusion assay was performed. Briefly, 5 mL of mid-log phase grown culture of C. albicans 077 was centrifuged at 4000 rpm for 5 min at 4 °C. Then the pellet was washed with 1 × PBS and resuspended in 500 μL of a normal saline solution (NSS). Hundred μL of the suspended cells were spread uniformly on SD agar plates and the plates were incubated at 37 °C for 30 min. The seeded petri plates were used for the loading of the various concentrations of cfPNCs (0, 5, 10, and 15 μg/mL) onto filter paper discs. To see the effect of PANI (aniline; 0.1 M, 0.2 M, 0.3 M, 0.4 M, and 0.5 M) on the anticandidal activity, 5 μg/mL of the synthesized cfPNCs were loaded onto the filter paper discs mounted on C. albicans 077-seeded petri plates. The Petri plates were incubated at 37 °C for 40 h, then the zone of inhibition was determined by measuring the diameter of C. albicans 077 cell clearance.
2.4.2.2 In vitro killing assay
To determine the in vitro killing activity of the cfPNCs, the C. albicans 077 cell suspension was obtained similarly as described for the agar disc diffusion assay (Section 2.4.2.1). The suspended cells (∼100 μL) were dispersed into the 96-well microtiter plate in triplicates. To this, various concentrations of cfPNCs (0, 5, 10, and 15 μg/mL) diluted in 100 μL of a sterile SD broth medium were added and incubated at 37 °C for 2 h. The whole suspension of the plate wells was spread on the SD agar plate and incubated at 37 °C for 40 h. Anticandidal activity was detected by dose-dependent reduction in a number of colony forming units (cfu) in terms of absorbance data.
2.4.2.3 Growth kinetics assay
To see the effect of the cfPNCs on the growth kinetics of C. albicans, 50 mL of SD broth in individual flasks were inoculated with 100 μL of the NSS suspended cells of Candida cells. The different concentrations of cfPNCs (0, 5, 10, and 15 μg/mL) to be tested were poured in the individual flasks. The flasks were incubated at 37 °C for 40 h and the time-dependent growth kinetics were recorded turbidometrically at A595 nm. The experiment was performed in triplicates and the turbidity background induced by cfPNCs was subtracted from the final reading.
2.5 Intracellular measurement of ROS generation
The production of intracellular reactive oxygen species (ROS) was measured using 2,7-dichlorofluorescin diacetate (DCFH-DA) [36]. The DCFH-DA passively enters the cell, where it reacts with ROS to form the highly fluorescent compound dichlorofluorescein (DCF). Briefly, a DCFH-DA (10 mM) stock solution in methanol (HPLC grade) was diluted in the culture medium to yield a working solution (100 μM). At the end of exposure, C. albicans 077 cells were washed twice with ice-cold 1X PBS and then incubated in 1 mL of a working solution of DCFH-DA at 37 °C for 30 min. The C. albicans 077 cells were treated with different concentrations of cfPNCs for 40 h, lysed in alkaline solution and centrifuged at 5000 rpm for 10 min. Then, 200 μL of the supernatant was transferred into the other fresh well of microtiter plate, and fluorescence was measured at an excitation of A485 nm and emission of A520 nm using a microplate reader (Bio-Rad laboratories Inc., Hercules, CA, USA).
3 Results and discussion
Developing biocompatible advanced functional materials with antimicrobial properties may be promising for environmentally benign applications. In this regard, polymer-based functionalized metal-oxide-based nanocomposite materials are of great interest due to their broad spectrum of antimicrobial potentials and applications in medicine. In this study, we have assessed the anticandidal activity of the polymer-functionalized CoFe2O4 NPs. Our results had shown that cfPNCs significantly kill C. albicans 077 cells at specific concentrations.
3.1 Synthesis and characterization of PANI/CoFe2O4 nanocomposite (cfPNCs)
The synthesis of cfPNCs was validated by the UV-visible spectrum recorded from A250 to 800 nm in NMP as a solvent. The typical UV-visible spectrum is shown in Fig. 1A; it showed two major distinctive peaks arisen through the presence of the PANI at positions ∼A330 nm and ∼A650 nm. The absorption band around A330 nm is attributed to the π–π* transition of the benzenoid ring, and the peak around ∼A650 nm corresponds to the charge transfer from the benzenoid rings to the quinoid rings [37]. Besides that, the presence of the absorption peak at ∼A275 nm reflected the presence of CoFe2O4NPs embedded in the PANI matrix. The absorption peak at ∼A330 nm and ∼A650 nm corresponding to PANI were red and blue shifts in cfPNCs, respectively, owing to the presence of CoFe2O4 NPs (Fig. 1A). Thus, this result suggests that the interaction takes place between CoFe2O4 NPs and PANI chains. The absorption band widening towards the visible light region in case of cfPNCs was observed, and this has been used for the visible-light-driven ROS-mediated photocatalysis of various dyes [38]. The electronic structure of cfPNCs is characterized by the energy band gap, which is essentially the energy interval between the valence band (Ev) and the conduction band (Ec). The generation of a specific type of ROS such as •OH, 1O2, or O2•− is governed by the metal-oxide-based NPs related to the energy band gap as well as by the redox potentials (EH) of the different ROS generation reactions [33,39]. These ROS species induced by metal-oxide-based NPs have been established as the main mechanism of their antimicrobial action. Therefore, we have calculated the electronic band gap energy of cfPNCs from the absorbance data. The electronic band gap of the cfPNCs was determined by employing the Tauc relationship given below:
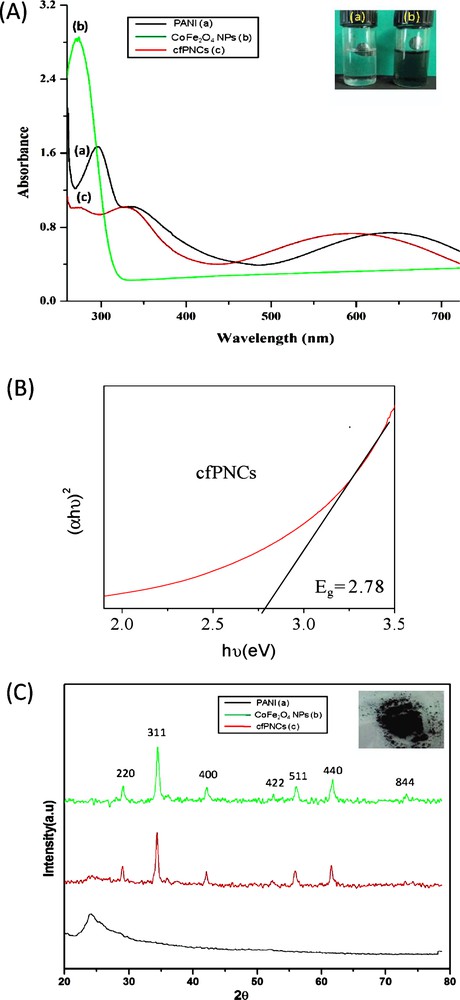
(A) UV-visible absorption spectra of (a) PANI, (b) CoFe2O4 NPs and (c) cfPNCs were recorded between A250 nm to A800 nm using N-methyl-2-pyrrolidone (NMP) as a solvent. The inset in the figure depicts the NMP solution of as-synthesized (a) CoFe2O4 NPs and (b) cfPNCs, respectively. (B) Tauc plot depicting the energy band gap (Eg) of cfPNCs. (C) XRD patterns of (a) PANI, (b) CoFe2O4 NPs, and (c) cfPNCs, recorded in the 20°–80° 2θ angular range. (a) The peak at ∼24.25° can be indexed as revealing the amorphous nature of PANI. (b) The XRD pattern of CoFe2O4 NPs evidenced well-resolved diffraction peaks, which can be assigned to reflection planes (220), (311), (400), (422), (511), (440), and (844). (c) As-synthesized cfPNCs XRD pattern, showing well-resolved diffraction peaks, which can be assigned to reflection planes (220), (311), (400), (422), (511), (440), and (844) of CoFe2O4 NPs embedded in amorphous PANI. The inset in the figure depicts the dried dark brown colour solid powder of synthesized CfPNCs.
The XRD patterns of synthesized cfPNCs show well-resolved diffraction peaks, which can be assigned to the reflection planes (220), (311), (400), (422), (511), (440), and (844) of the CoFe2O4 NPs present in the PANI matrix (Fig. 1C). The reflection plane pattern was observed to be similar to the characteristics of the spinel cubic structure of CoFe2O4 {space group: Fd3 m (227), JCPDS card No. 22-1086} [40]. The mean crystalline size of CoFe2O4 NPs, calculated by the Debye–Scherrer equation, was found to be ∼19.58. The additional peaks at ∼24.25° depicted in Fig. 1C (a) can be indexed as reflecting the presence of amorphous PANI to the periodicity parallel and perpendicular to the polymer chains. The relative intensity of CoFe2O4 NPs diffraction peaks was reduced in cfPNCs compared with that of the bare CoFe2O4 NPs. Therefore, the results confirmed that the as-synthesized cfPNCs, PANI matrix encapsulated CoFe2O4 NPs.
The surface morphology and particle sizes of as-synthesized cfPNCs were determined by SEM, TEM, and AFM. The representative TEM micrograph of CoFe2O4 NPs is shown in Fig. 2A. The TEM micrographs shown in Fig. 2B exhibited a porous and well-interconnected irregular structure with irregular shapes and the porous nature of the cfPNCs is evidenced by TEM images taken at high magnification (Fig. 2C). The TEM micrographs indicate that the surface of cfPNCs was rough, with a more or less spherical morphology ranging from ∼18 to 22 nm. The micrographs exhibited dark areas, indicating the presence of the CoFe2O4 NPs, while the bright area represents the PANI matrix encapsulated in the CoFe2O4 NPs (Fig. 2C). The microscopic results also confirmed that the PANI abridged the aggregation of CoFe2O4 NPs through repulsive forces between CoFe2O4 NPs and the PANI matrix. The agglomeration showed in the TEM images could be due to the preparation technique, as particle deposition on a copper grid and the drying process promote agglomeration [36].
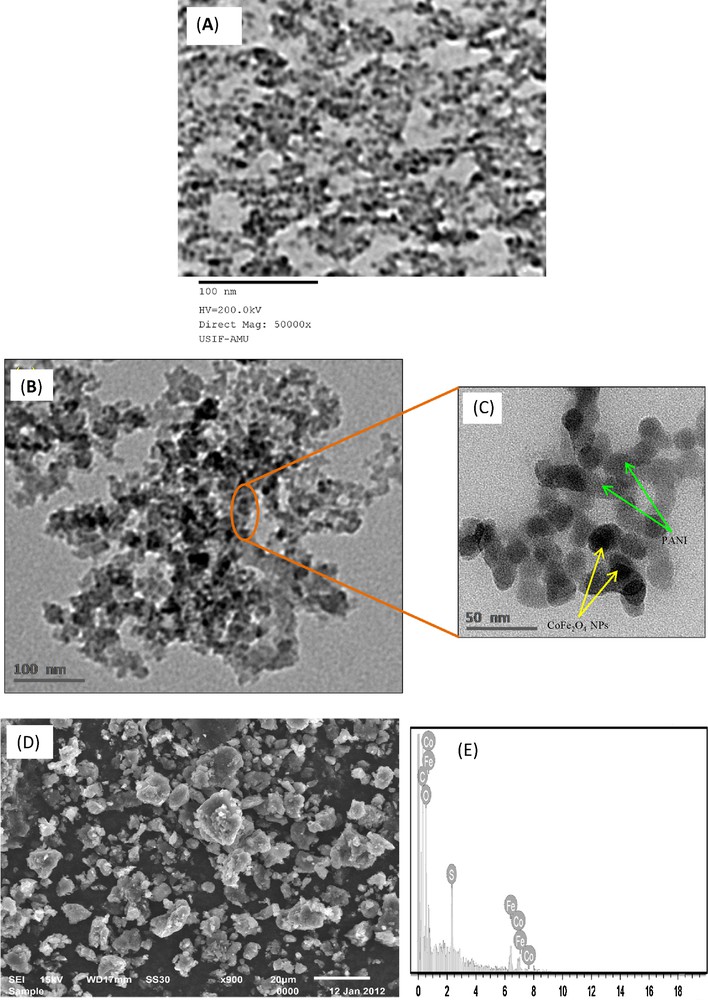
(A) Transmission electron micrograph of CoFe2O4 NPs. (B and C) Transmission electron micrographs of cfPNCs exhibiting a porous and well-interconnected irregular structure with irregular shapes, at low and high magnifications, respectively. (D) Scanning electron micrograph of a cfPNCs powder, depicting micrometric aggregates consisting of nanometric spherical CoFe2O4 NPs in the PANI matrix. (E) EDAX spectrum, showing the elemental composition of cfPNCs and evidencing the presence of Fe, Co, and O.
The morphology of the cfPNCs powder was also examined by SEM, as shown in Fig. 2D. The results revealed the formation of micrometric aggregates consisting of nanometric spherical particles. Moreover, elemental analysis using EDAX (Fig. 2E) indicates the presence of Fe, Co, and O in as-synthesized cfPNCs. The atomic ratio of Fe to Co has to be found very close to the stoichiometric Fe/Co ratio in CoFe2O4 NPs, which further validates the existence of CoFe2O4 NPs entrenched in the PANI matrix. The AFM images also show the spherical morphology of cfPNCs in the size range between 12 and 21 nm (Fig. 3A and B), which is consistent with cfPNCs results obtained by TEM and SEM. The overall microscopic data confirmed that as-synthesized cfPNCs contain the nano-scale CoFe2O4 NPs with a more or less spherical shape.
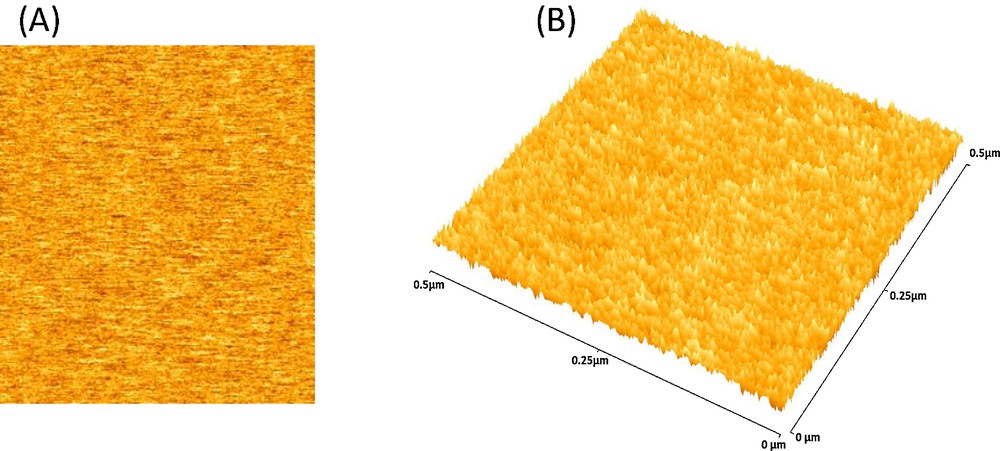
Atomic force 2D (A) and (B) 3D micrographs illustrating the nanostructure of as-synthesized cfPNCs, respectively.
The chemical structure of the as-synthesized cfPNCs was ascertained by FTIR spectroscopy. The FTIR spectra of the pure PANI and cfPNCs are shown in Fig. 4A. The spectrum of pure PANI revealed characteristic bands at positions 1580.54 cm−1, 1498.32 cm−1, 1303.12 cm−1, 1148.44 cm−1, and 818.93 cm−1. The band positions at 1580.54 cm−1 and 1498.32 cm−1 were attributed to the characteristic CC stretching of the quinoid and benzenoid rings, while the band position at 1303.12 cm−1 was assigned to the CN stretching of the secondary aromatic amine. The bands at position 1148.44 cm−1 and 818.93 cm−1 reflect the aromatic CH in-plane bending and the out-of-plane deformation of C–H in the 1,4-disubstituted benzene ring, respectively. The FTIR spectrum of cfPNCs exhibited the homology with pure PANI since, because of the higher mass of the participating atoms, vibrations of transition metal–oxygen bonds appear in the far-infrared region. These results indicate that there was some interaction between CoFe2O4 NPs and the PANI backbone. The FTIR spectrum of the cfPNCs exhibited that the deviations in the absorption bands at 1604.67 cm−1 and 1455.87 cm−1 can be assigned to the quinonoid ring and the benzenoid ring, respectively. It can be deduced that PANI in both pernigraniline form and emeraldine form are coexisting in the resultant composite. Furthermore, cfPNCs shows that the prominent stretching bands at 3440.54 cm−1 and 2923.12 cm−1 are assigned to the NH stretching vibrations for aromatic amine and –CH stretching, respectively. The change in the band's position clearly indicates that the NH group of the alanine ring interacts with the Fe2+ ions of the CoFe2O4 NPs. The presence of the additional band at position ∼557.43 cm−1 is the signature band of FeO stretching; thus the data revealed the presence of CoFe2O4 in the PANI matrix [37].
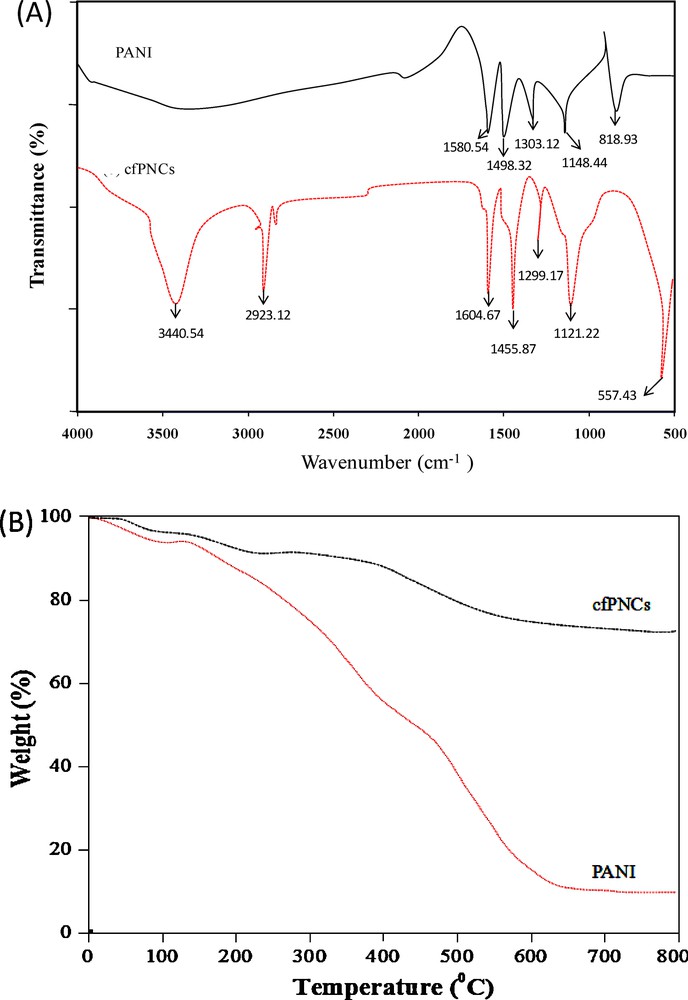
(A) FTIR spectra, showing the interaction between the PANI and CoFe2O4 NPs in cfPNCs. (B) TGA graph, showing the thermal behaviour of PANI and cfPNCs.
The thermogravimetric analysis (TGA) analysis revealed the composition of cfPNCs (Fig. 4B). The TGA curve depicted the two weight loss steps of the pure PANI polymer; in the first step, it indicates a weight loss at a temperature up to ∼110 °C, which may be ascribed to the eviction of a water molecule and of the dopant (HCl) from the PANI chains. The other weight loss step was observed in the ∼200–600 °C temperature range, where the higher temperature causes volatilization and thermal degradation of the lower-weight PANI chains. TGA analysis of as-synthesized cfPNCs exhibited the highest thermal stability in contrast to the PANI. The TGA curve of the cfPNCs reveals a weight loss of ∼22% at 500 °C. In contrast, pure PANI shows the same weight loss at 280 °C. The high thermal stability of cfPNCs is due to a strong interaction between PANI and CoFe2O4 NPs, which restricts the thermal motion of PANI in the composite. It also revealed that PANI itself was decomposed thermally within this temperature range, and that pure PANI has the great weight loss (∼80%) at temperatures between 200 and 600 °C. The overall TGA data reveal that, in this study, the as-synthesized cfPNCs have the higher thermal stability, although the thermal stability of the cfPNCs depends on the amount of CoFe2O4 NPs that are present in the PANI matrix [34].
3.2 Anticandidal activity of PANI/CoFe2O4 nanocomposite (cfPNCs)
In light of the evidence of the rapid global spread of resistant clinical isolates of C. albicans, the need to find new anticandidal agents is of supreme importance. However, the past records of rapid, widespread emergence of resistance to newly introduced antifungal agents indicate that even new families of antimicrobial agents will have a short life assurance. Therefore, researchers are increasingly turning their attention to nanomaterials, looking for new leads to develop better nano-antimicrobial drugs against MDR C. albicans strains [41]. Therefore, in the current study, we have assessed the anticandidal activity of cfPNCs against MDR C. albicans 077. The disc diffusion and in vitro killing assays revealed that cfPNCs efficiently inhibits the growth of C. albicans 077 in a dose-dependent manner (Fig. 5A and B). Furthermore, SEM analysis of treated and untreated C. albicans 077 cells confirmed that cfPNCs have promising anticandidal activity (Fig. 5C). Growth-inhibition results based on the growth curve analysis of C. albicans in the presence of different concentrations of cfPNCs are shown in Fig. 5D. The untreated sample cells showed a normal pattern of growth with a lag phase of ∼4 h, an active exponential phase of 8 to ∼20 h before attaining the stationary phase. The anticandidal activity of cfPNCs leads to the significant concentration-dependent manner suppressed growth and delayed exponential phases of C. albicans 077 (Fig. 5D). The disc diffusion assay data revealed that the addition of different concentrations of PANI marginally enhanced the anticandidal activity of cfPNCs (Fig. 5E). PANI has inherent antimicrobial activity and shows growth inhibition of the bacteria and fungi under in vitro conditions [27,28].
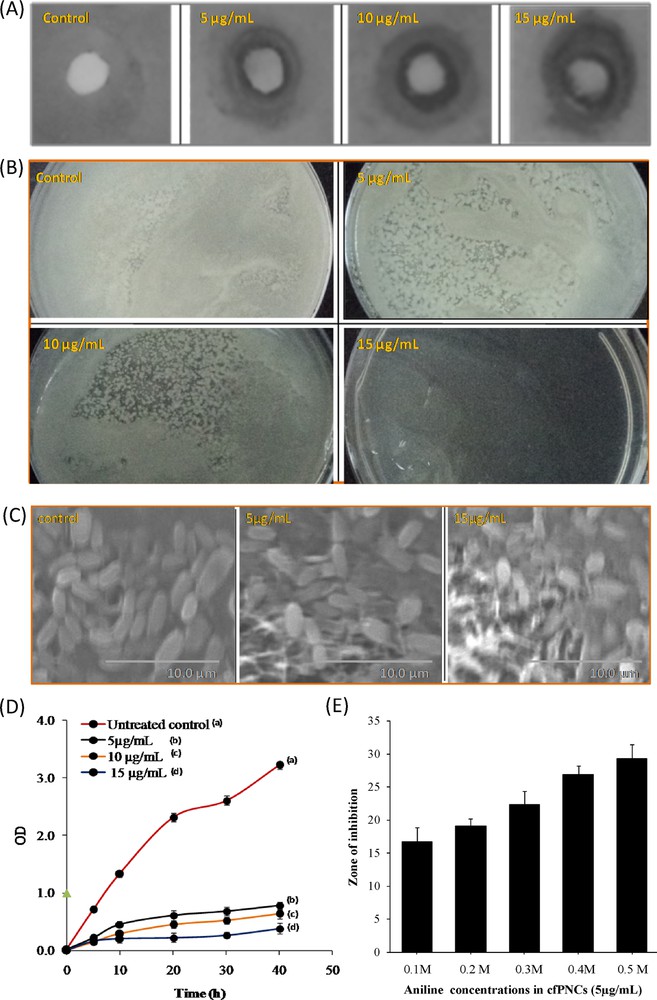
(A) Inhibition zone and (B) in vitro killing assays showing the anticandidal activity of cfPNCs against C. albicans 077, respectively. (C) SEM-based observation of changes in the cell morphology of C. albicans 077 treated with cfPNCs (15 μg/mL). (D) Growth curve analysis giving the growth inhibition of C. albicans 077 in the presence of different concentrations of CfPNCs. (E) Effect of PANI on the antimicrobial activity of cfPNCs (5 μg/mL).
The result shows that MDR C. albicans 077 was sensitive to cfPNCs with minimum inhibitory concentration (MIC) ∼13.5 μg/mL of cfPNCs. The MIC is the lowest concentration of the compound at which the microorganism tested does not demonstrate visible growth. The almost complete cessation of growth was observed at a concentration of 15 μg/mL. However, a vast divergence {0.4–0.8 μg/mL silver NPs [42] to 2000 μg/mL selenium NPs [43]} in the MIC values of various kinds of NPs against C. albicans strains has been reported in the literature. These differences in the MIC values were probably attributed due to the strain type, the synthesis method, the NPs type, and their surface modifications, etc. The obtained anticandidal results of the current study corroborate our findings that have been published recently on the NP- and nanomaterial-mediated inhibition of the growth of Candida spp. [42,43].
3.3 Stability of PANI/CoFe2O4 nanocomposite (cfPNCs) in SD broth medium and during storage
We also determined the stability of cfPNCs (15 μg/mL) in a SD broth culture medium up to 72 h at 37 °C through the change in their UV-visible absorbance characteristics [33,36]. No significant change such as agglomeration and absorbance of cfPNCs has been noticed in the course of cell culture medium incubation (Fig. 6A), suggesting that the SD broth culture medium does not affect cfPNCs’ stability, size and integrity. However, the incubation of the bare and surface modified NPs in the culture medium does not affect stability, size and integrity, respectively [36,44]. Also, it was observed that the colloidal solution of cfPNCs remained stable for 120 days and that there was no significant change in the absorbance (Fig. 6B).
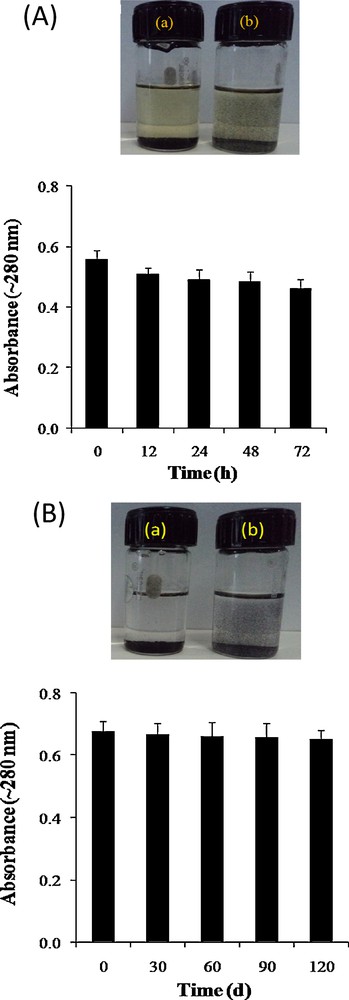
(A) Stability of the (a) CoFe2O4 NPs and (b) cfPNCs in the SD broth medium. The stability of cfPNCs in the SD broth culture medium was monitored up to 72 h at 37 °C through the change in UV-visible absorbance characteristics. (B) Stability of the (a) CoFe2O4 NPs and (b) cfPNCs in an NMP solution during storage. The stability of cfPNCs in NMP was monitored up to 72 h at 37 °C through the changes in UV-visible absorbance characteristics. The bar graph illustrates the non-significant change in the absorbance of cfPNCs (15 μg/mL) after 120-day storage at 25 °C.
3.4 Role of ROS produced by PANI/CoFe2O4 nanocomposite (cfPNCs) in anticandidal activity
In our recent study [33], ZnO NPs were shown to induce cell death of C. albicans 077 cells by ROS production. In light of these results, we cogitate that the anticandidal effect of cfPNCs on C. albicans 077 may be mediated through ROS, even though oxidative stress induced by reactive oxygen species (ROS) is a well-known inducer of cytotoxicity and cell death in C. albicans [45]. We therefore tested whether the ROS produced by the cfPNCs are responsible for its inhibition of C. albicans 077 growth. The protective effect of histidine, a known scavenger of hydroxyl radicals and singlet oxygen, on the anticandidal activity of cfPNCs was tested by adding histidine (5 mM) to a C. albicans 077 culture containing cfPNCs at 15 μg/mL. Fig. 7A shows a protective effect of histidine on growth enhancement of C. albicans 077 in the presence of cfPNCs. Histidine (5 mM) almost completely protects C. albicans 077 from the anticandidal activity of cfPNCs. The fact that histidine reduced the effect of cfPNCs on C. albicans 077 viability (Fig. 7A) is supported by the ROS results shown in Fig. 7B, exhibiting a reduction in the amount of ROS produced within the C. albicans 077 cells treated with cfPNCs, after the addition of histidine. However, various researches have been done on the generation of ROS by various metal-oxide-based NPs. Recently, an electronic band gap structure of the metal oxides NPs with the redox potentials (EH) has been correlated with different ROS generation reactions [14,19,39]. Thus, when cfPNCs gained a higher energy than Eg, the electrons (e−) of CoFe2O4 NPs were promoted across the band gap to the conduction band (Ec), which creates a hole (h+) in the valence band (Ec). These e− in the EC and holes in the Ev possibly have high reducing and oxidizing powers, respectively. The electrons react with molecular oxygen to produce the superoxide anion (O2•−) through reductive reactions. The hole h+ can abstract an e− from water and/or hydroxyl ions to generate •OH (Fig. 7C and D). The promising anticandidal activity demonstrated in this study was attributed to the enhancement of the rapid separation efficiency of e− and h+ through an electronic interaction between CoFe2O4 NPs and PANI [37,38]. Taken together, the results of this study confirmed that the ROS principally contribute to the anticandidal activity of the cfPNCs (Fig. 7E).
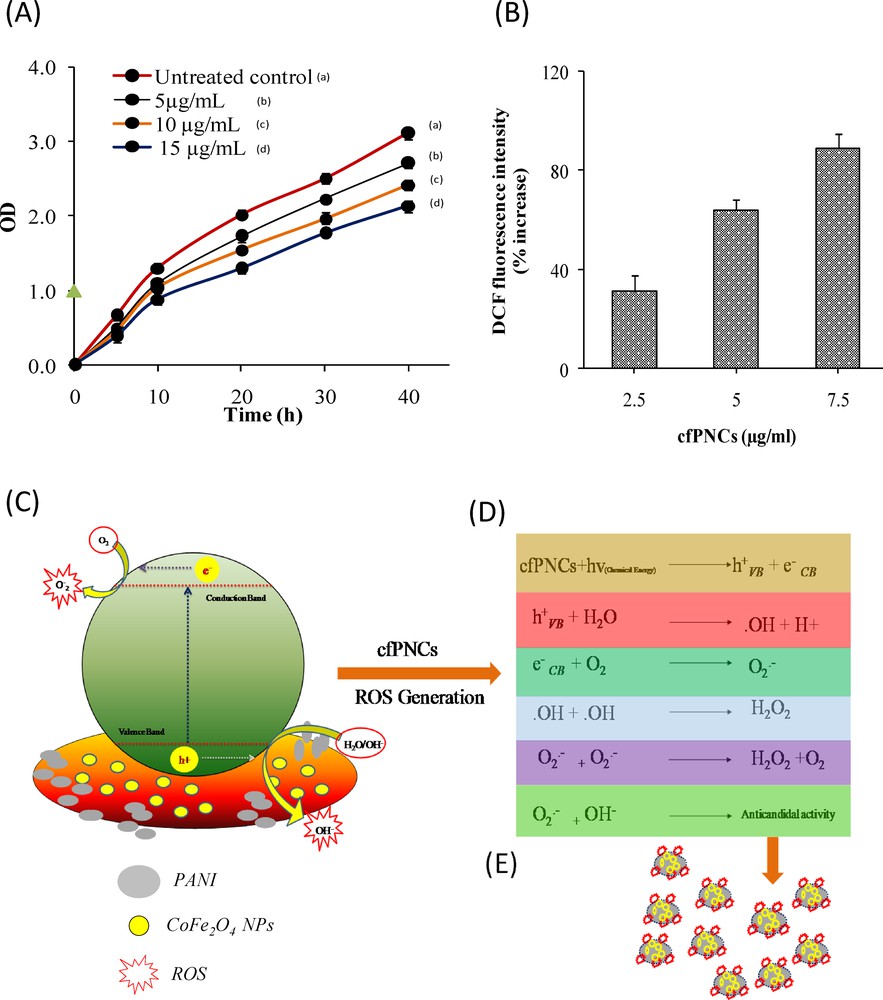
Role of ROS produced by cfPNCs in anticandidal activity. (A) Analysis of the growth curve shows the protective effect of histidine (5 mM) on C. albicans 077 growth inhibition in the presence of different concentrations of cfPNCs. (B) Determination of the ROS production within the C. albicans 077 cells treated by different concentrations of cfPNCs. (C and D) Plausible mechanistic aspects of ROS generation by cfPNCs and their role in anticandidal activity. (E) cfPNCs inside the cytoplasm; they can interfere with energy production in mitochondria and promote the generation of ROS. ROS may cause cell-lysis and DNA damage in C. albicans 077.
4 Conclusion
We have demonstrated here cfPNCs’ anticandidal activity against C. albicans 077 by alleviating ROS-mediated oxidative stress and revealed possible underlying mechanisms. In future studies, we will suggest in-depth in vitro and in vivo studies that will help to identify cfPNCs’ anticandidal potential for the management of diseases caused by MDR C. albicans isolates.
Acknowledgments
Financial support for this work through the Centre of Excellence in Materials Science (Nanomaterials), Department of Applied Physics, Z.H. College of Engineering & Technology, Aligarh Muslim University, Aligarh-202002, Uttar Pradesh, India is greatly acknowledged. B.R. Singh is greatly thankful to CSIR Govt. India for award of Pool Scientist.