1 Introduction
The influence of using NHC–Pd metal catalysis in the formation of CC bonds has been effectively demonstrated by numerous research groups worldwide. In the past few years, N-heterocyclic carbenes (NHCs) have become increasingly popular as suitable ligands for the synthesis of a large range of coordination compounds. The flexibility of NHCs continues to be fruitful in many interesting methods of preparing complexes efficiently. In coordination chemistry, NHC ligands are broadly equated with phosphines owing to their resemblance and wide-ranging application in catalysis. The excellent donating capability of NHCs related to phosphine ligands provides strong metal–ligand bonding, which leads to an electron-rich metal center and affords high strength and catalytic activity to the transition metal complexes. Subsequently, at higher temperatures, phosphines suffer from important PC bond degradation of the complexes compared with NHCs that show little tendency to dissociate from the metal center because of strong-donor but poor π-acceptor character [1].
The last two decades have witnessed many productive applications of NHC–Pd complexes in the field of organometallic chemistry and catalysis [2]. The newly formed compounds are provided with specific ligands so as to achieve an appropriate balance between the electronic and bulky environments around the metal and assist controlling their activity, stability, and chemoselectivity profiles. The new generation of palladium complexes bearing NHCs shows improved thermal and oxidative stability, making it a long-lived and active catalyst, a property which in future will be considered as a key assist of this type of ligands [3,4]. Besides the aforementioned benefits, this group of ligands is also easily accessible through well-established synthetic protocols while in synthesis, an additional ligand is not required to prevent aggregation of the catalyst. NHC ligand has very diverse structures but the most frequently encountered functionalities in the catalytic complexes are the following prototypes: triazo-5-ylidenes, imidazole-2-ylidenes, and thiazo-2-ylidenes.
The growth and efficiency of NHCs have enormously augmented both organic catalysis and organometallic chemistry as evident from the first report of NHCs by Wanzlick [5] in the early 1960s and the significant breakthrough of NHC-transition metal complexes [6]. Later this innovation was subsequently exploited in organic synthesis as well, with the successful isolation of first stable NHC, by Schmidt et al. [7].
Furthermore, in 2010 Akira Suzuki, Ei-ichi Negishi, and Richard F. Heck got the Nobel Prize in chemistry for palladium catalyzed cross coupling in organic synthesis. NHC ligands have become abundant in organometallic chemistry surpassing that of the phosphine ligands, which is also revealed by the recent viable accessibility of a wide range of NHCs and their precursors [8]. For this reason, metal–NHCs have been generally applied in organic chemistry for the most important Pd-catalyzed CC bond formations such as the Heck reaction [9], Suzuki reaction [10], Stille reaction [11], Hiyama coupling [10], Sonogashira coupling [12], Buchwald–Hartwig amination [13], Kumada coupling [14], and Negishi reaction [15]. Therefore, our prime intention is to provide an updated review on the development of NHC–palladium complexes: their structure, metal coordination chemistry, bonding theory, and applications for the Heck reaction, which could further accelerate the research on this topic.
2 Mizoroki–Heck reaction
The Mizoroki–Heck reaction is the most effective path for the vinylation of aryl/vinyl halides or triflates, catalyzed by palladium in the presence of a base. The reaction is also well known for the substitution of olefin-hydrogen with the aryl group, which accomplished vast synthetic importance [16]. The progress in metal-catalyzed alcohol activation, amidation, etherification, amination, hydroamination, and hydroacylation reactions, which have been elucidated in the past two decades, leads to metal catalyzed CC bond formation [17] as an interesting area with abundant scope for the investigator.
The palladium(II)-assisted vinylic substitution of an organometallic reagent was first reported by Heck [17,18] using stoichiometric amounts of palladium(II) salt, two decades back. A catalytic preference was described by Pucheault et al. using organoboronic acid involving the use of a base [19]. Since then, various protocols have been carried out for the palladium-catalyzed Heck reaction of organometallic reagents with electron-deficient olefins [20]. This is predominantly correct from the perception of catalysis, which intends to determine a consistent method for a wide range of metal-catalyzed bond-forming routes. The CC bond-forming reactions are achieved from aryl derivatives influenced by the catalytic precursors, bases, ligands, and the reactivity of intermediate palladium(0) or palladium(II) complexes. In such a crucial field, the modification of the Heck–Mizoroki reaction is essential for improving the efficiency and regioselectivity of the catalytic reactions [21,22].
The mechanism was proposed for the Heck reaction (Scheme 1) [23] that involves the oxidative addition of haloalkenes or haloarenes to palladium(0), followed by migratory syn-insertion of a complexed olefin. Internal rotation about a CC bond enables syn-β-hydride elimination to produce a coupled product and allows regeneration of the palladium(0) species. The catalytically active complex is assumed to be a coordinately unsaturated 14-electron palladium(0) species with weak donor ligands coordinated to it.
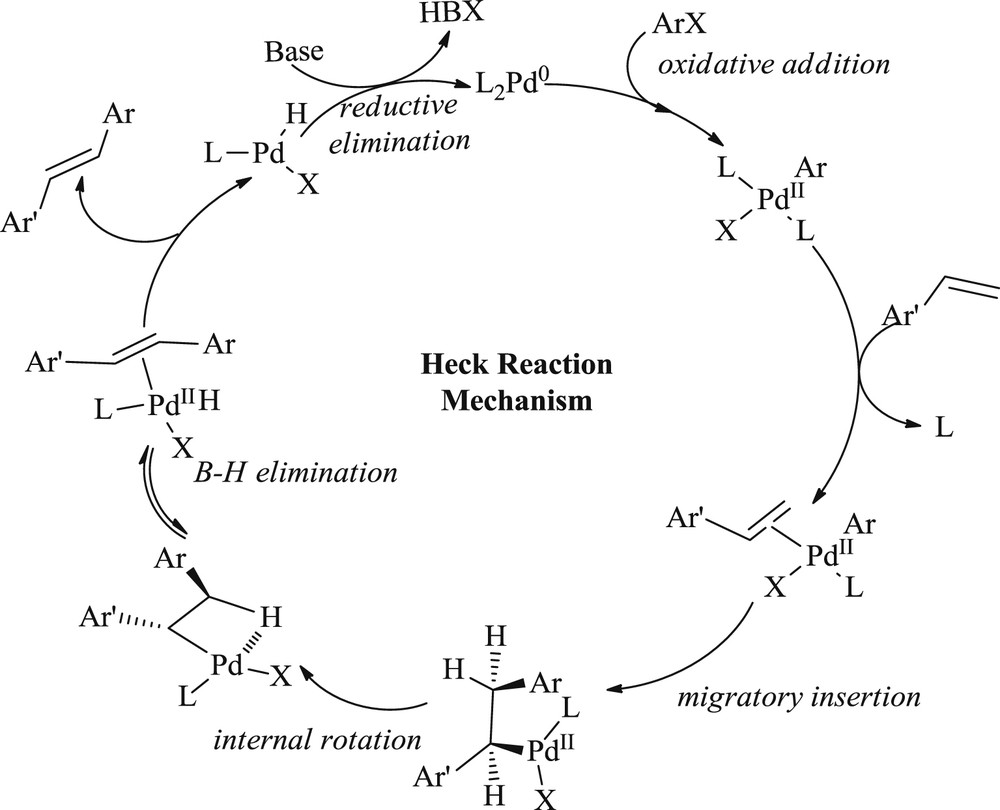
Mechanism of the Heck reaction.
Herrmann et al. [24] have described the mixed palladium(II) complexes of NHCs and phosphines (Fig. 1). This idea of mixing NHC and triaryl- or trialkylphosphines for catalysis is based on the intention to merge the strengths of both ligand systems. The high stability of the NHC–palladium bond is combined with the easy dissociation of the phosphine ligands. These NHC–phosphine complexes of palladium(II) provide admirable resources for triggering both the activity and stability of the catalytically active species in CC coupling reactions. The catalytic systems demonstrate the applicability of these catalysts in Mizoroki–Heck olefination, homocoupling of terminal alkynes (Scheme 2), Suzuki–Miyaura, and in Stille coupling for biaryl formations. The authors conclude that the vital feature of the catalysts is the necessity of bulky NHC ligands, whereas the phosphine ligand has to be optimized for each type of a reaction separately.
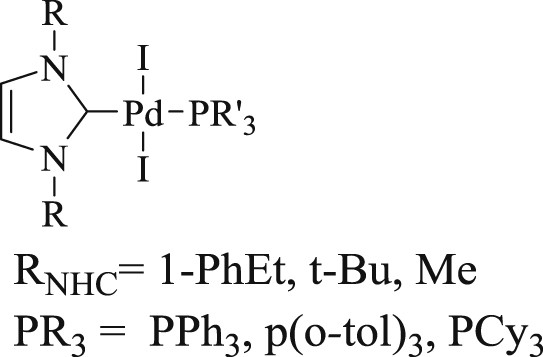
Mixed palladium NHC complexes.
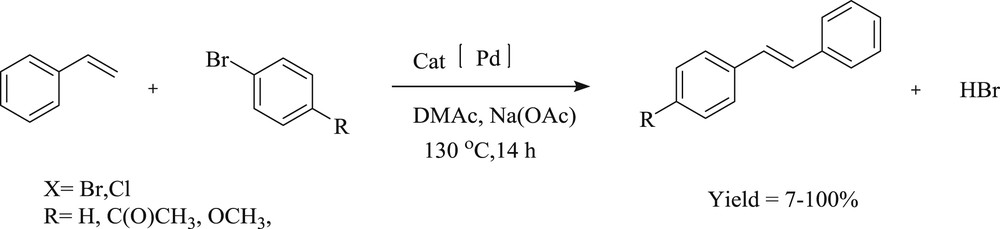
The Mizoroki–Heck reaction: coupling of different aryl halides and styrene using mixed palladium complexes.
Zhang et al. [25] developed a convenient, efficient, and high yielding route for the arylation of olefins (Scheme 3), facilitated by multidentate Pd–NHC salt (Fig. 2). The catalytic system is particularly stable toward air, moisture, and even several common oxidants. The CC coupling mediated by a imidazolylidene-based palladium complex can proceed in the presence of strong oxidizing agents, which was the most valuable contribution provided by the mechanism of the Heck reaction.
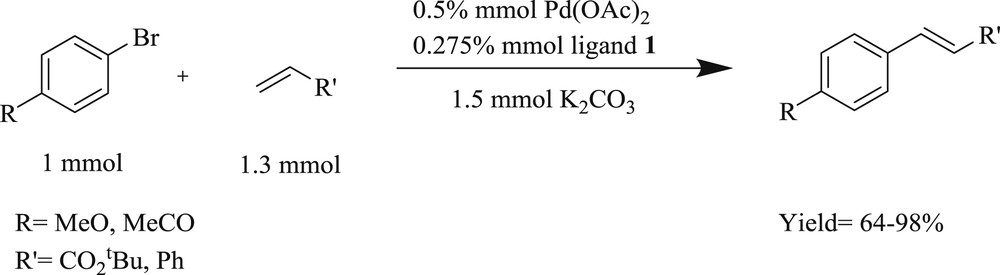
Heck reaction of aryl bromides with styrene/tert-butyl acrylate.
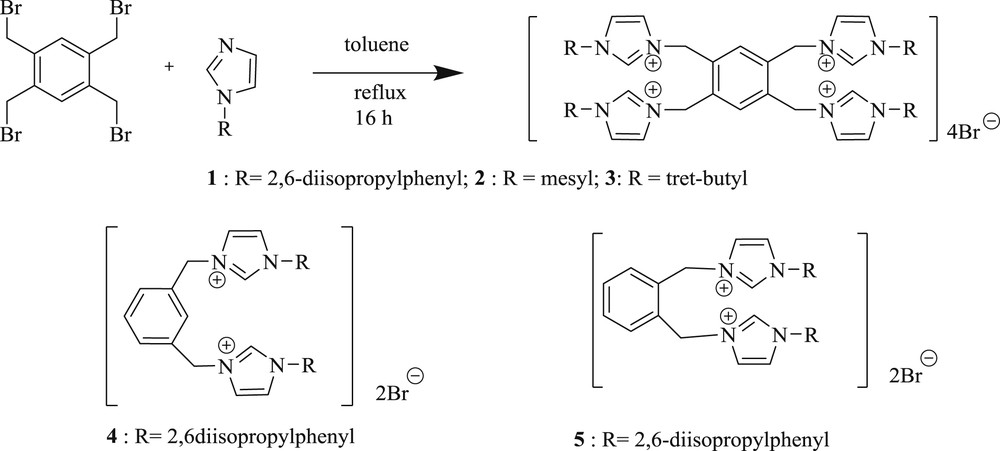
Synthesis of 1,3-disubstituted imidazolium salts.
Jutand et al. [26] have prepared very effective recyclable chiral diamino carbene Pd(II) salts with high air, moisture, and thermal stability, and these were tested in the Mizoroki–Heck reaction (Scheme 4). It is important to note that Pd(II) complexes bonded to carbene(s) are less easily reduced than those ligated by PPh3. The Pd(0) salts attached to the carbene(s) undergo facile oxidation than those bonded by PPh3 and thus are more reactive in oxidative additions with an aryl halide.
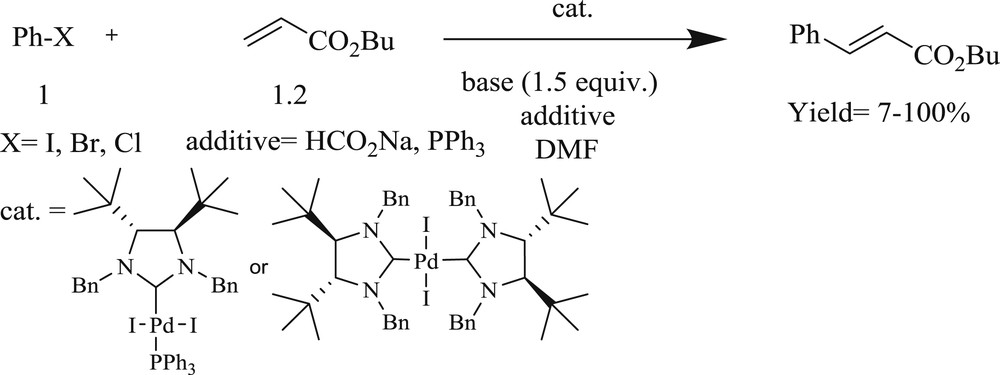
Heck reactions with NHC–Pd complexes.
Iyer et al. [27] have demonstrated N-ligands as an excellent alternative for the traditional P-ligands in the Pd-catalyzed Mizoroki–Heck reaction with appreciable yield. These metal complexes with N-ligands can be readily synthesized and they also possess the additional advantage of functional group modification compared with P-ligands. The degradation reaction associated with P-ligands is not observed in the N-ligands, with high thermal, moisture, and air stability. In addition, the absence of back bonding (available in the P-ligands) and the σ-donor ability of N-ligands cause activation of aryl and vinyl halide in the oxidative addition reaction by assisting in the labialization of the bromide and chloride.
An interesting result has also been reported [28] with a series of salts (Scheme 5), containing palladium 1,3-dialkylperhydrobenzimidazolin-2-ylidene (6–8) (Scheme 5) and palladium 1,3-dialkylimidazolium-2-ylidene (9–10) complexes. Styrene or phenyl boronic acid reacts with aryl halide derivatives in the presence of a catalytic amount of new palladium carbene complexes, PdCl2 (1,3-dialkylperhydrobenzolin-2-ylidene) or PdCl2(1,3-dialkylimidazolin-2-ylide), to give the corresponding CC coupling product in good yield (Scheme 6). It was concluded that 6 and 9 catalysts are more efficient for the Heck reaction of aryl bromides but their activity is much lower for the coupling of aryl chloride.
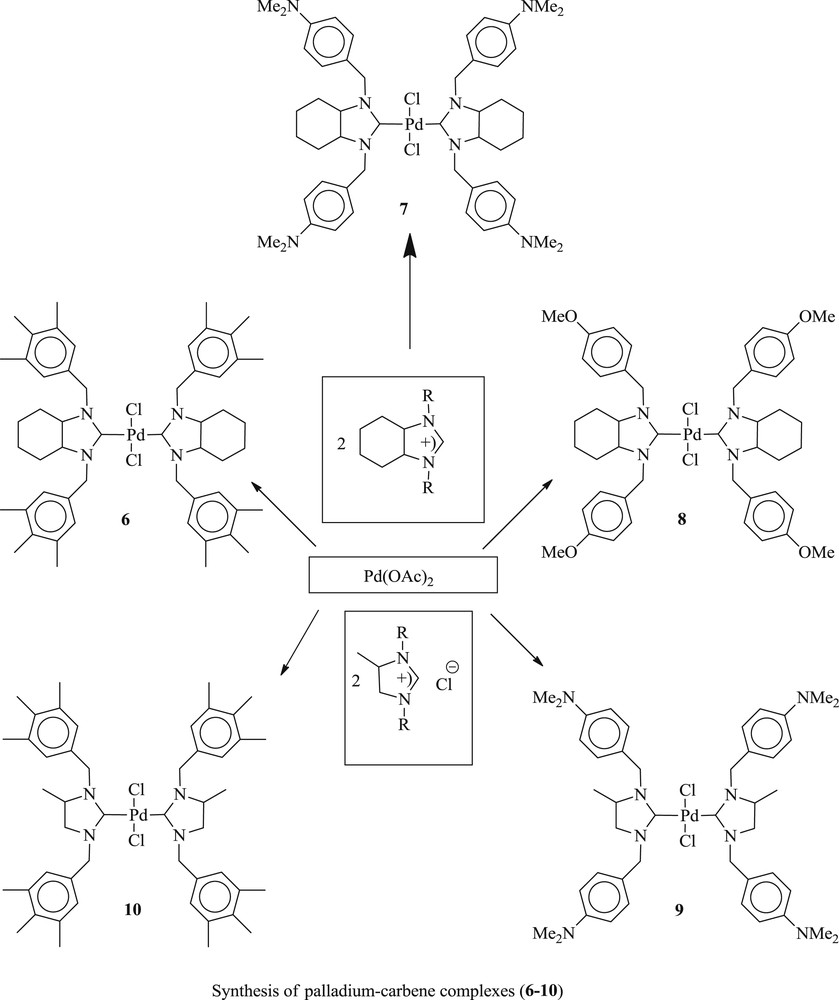
Palladium–carbene complexes 6–10.

Heck coupling reaction of aryl bromides with styrene.
Shi and Qian [29] have successfully prepared a dimeric bidentate NHC–Pd(II) system (Fig. 3) and used this as an effective catalyst for cross-coupling reactions under ambient conditions (Schemes 7 and 8). It was observed that the presence of electron donating groups in the substrate enhances the yield of the coupling products. It has also been illustrated that this system achieved the CC Suzuki–Miyaura coupling reaction with the corresponding products in appreciable yields.
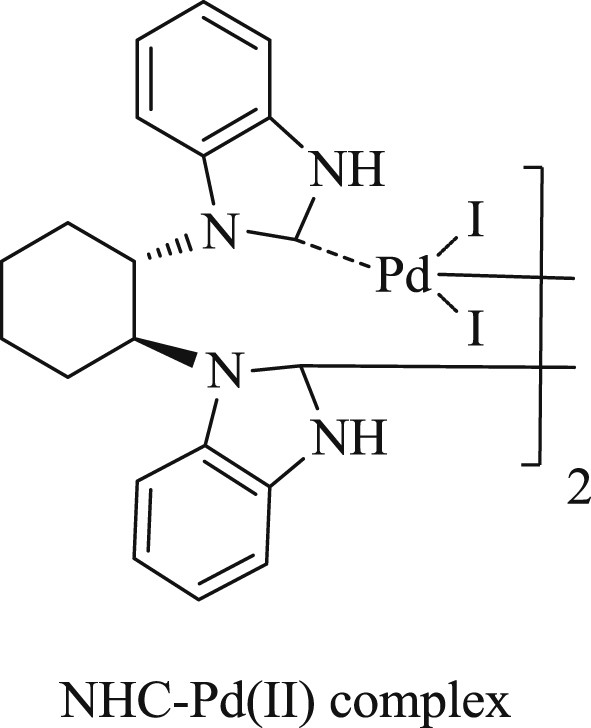
NHC–Pd(II) complex.

NHC–Pd(II) catalyzed Heck-cross coupling reaction of arylhalide with butyl acrylate.

NHC–Pd(II) complex catalyzed Heck-cross coupling reaction of phenylbromide with butyl acrylate.
Almost simultaneously, NHC phospha-palladacycles have also been successfully prepared [30] for the Heck coupling reaction (Scheme 9). This complex displayed high stability of phospha-palladacycles with the steric demand and σ-donor strength of NHCs. Also, it has been found that a less basic cyclometalated phosphine has a positive effect that increases the efficiency with very high tons of 10,800.
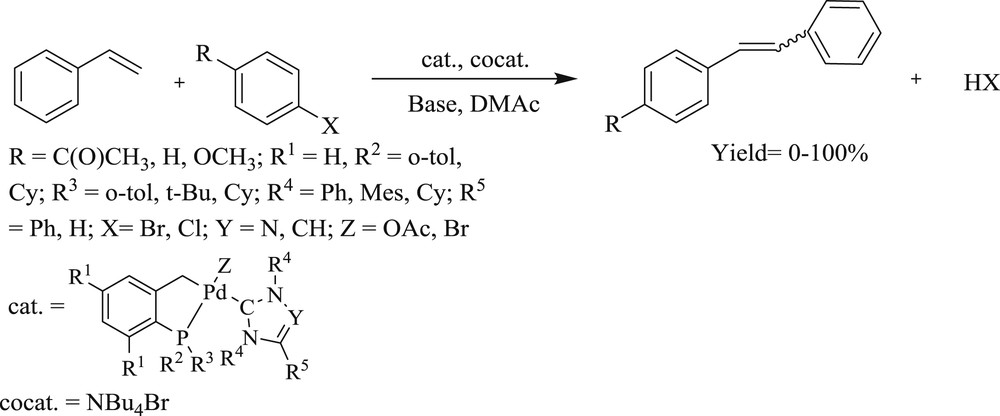
Heck olefination of aryl halides with styrene and NHC-palladacycles as catalysts.
A simple skill has been applied to the synthesis of the mono- and dinuclear palladium(II) benzothiazolin-2-ylidene salts [31] (Fig. 4), and good to excellent reactivity has been witnessed in the Heck reaction (Scheme 10).
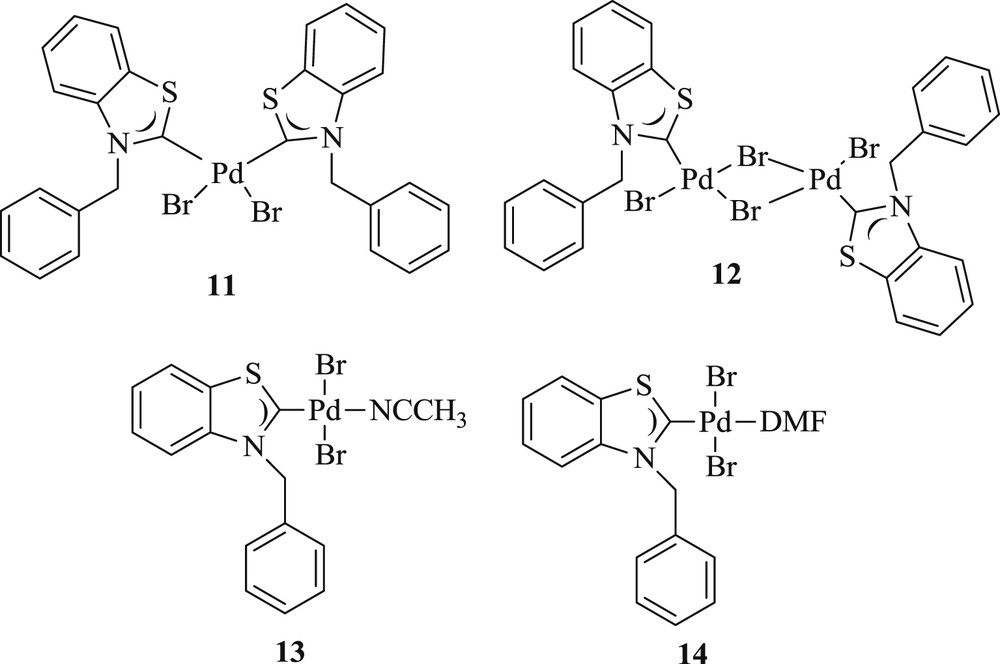
Palladium(II) carbene complexes 11–14.
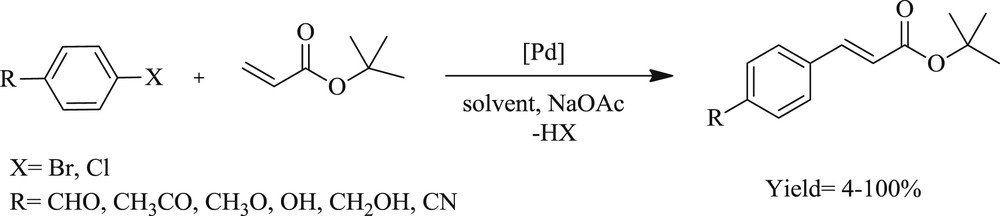
Mizoroki–Heck coupling reactions catalyzed by complexes 11–14.
Albrecht et al. [32] have performed an efficient Heck catalysis with the oxidative addition of iodo-functionalized diazolium salt (Fig. 5) to zerovalent palladium precursors. This provides a milder and more rational activity of the C(4)-bound carbene palladium complex compared with classical C(2)-bound carbene complexes.
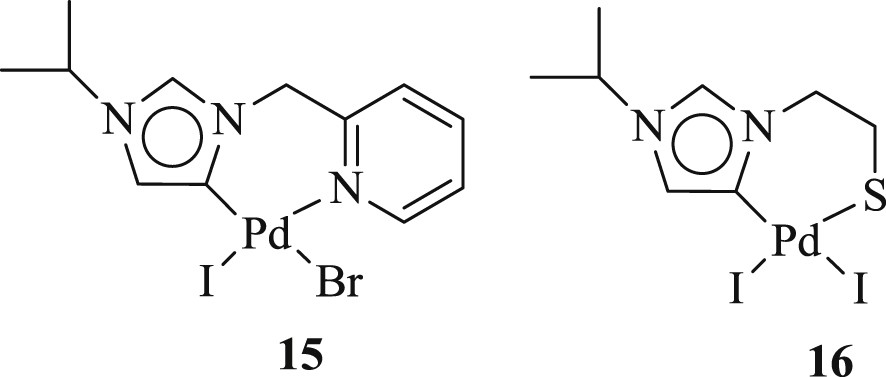
C(4)-bound Pd–NHC complexes 15,16.
A different pyrazolyl functionalized NHC–Pd(II) salt has been explored as well [33] that exhibited good performance toward the CC coupling reaction in ionic liquids (Scheme 11). Moreover, the Pd(II) complex could be reused three times with appreciable yield of the products. This work revealed that organic reactions catalyzed by hemilabile Pd(II) systems proceed competently in ionic liquids.
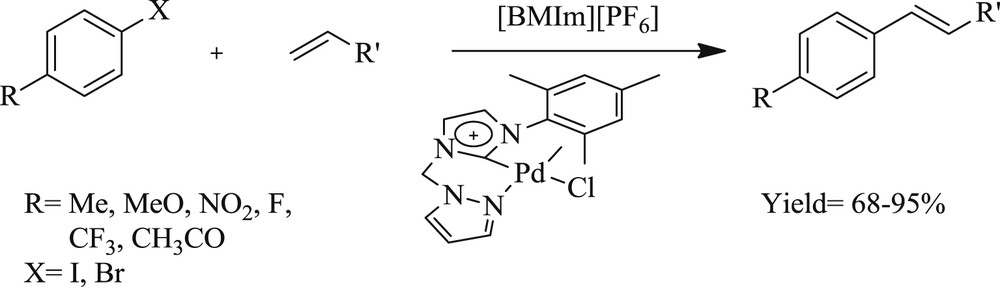
Pyrazolyl-functionalized NHC–Pd(II) catalyzed Heck reaction in an ionic liquid.
The coupling (Scheme 12) of aryl bromide and chloride with alkenes has been performed with an in situ palladium supported benzimidazolylidenes catalyst from N,N-dialkylbenzimidazolium salts in molten tetrabutylammonium bromide (TBAB) [34]. The reaction of 4-chloroacetophenone with butyl acrylate gave 4-acetyl-trans-cinnamic acid ester in 6 h, and the reaction was effected by 1 mol % of the benzimidazolium–palladium-containing 5,6-dibutoxy-N,N′-dibutylbenzimidazolium bromide. The authors also studied the electronic effect from aryl bromide that displayed a negligible impact on diarylation under catalysis of the bim-PdNaOAc system in TBAB. In this study, the reactivity of aryl halides with the electron-poor group was found to be more than the electron-rich group in the monoarylation. Similarly, mesityl bromide could be converted to butyl mesityl-trans-cinnamate using the same concentration of palladium.
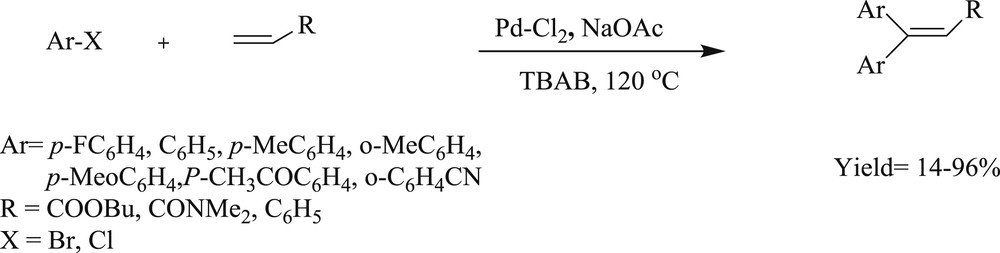
Monoarylation of olefins catalyzed by PdCl2–NaOAc in TBAB.
A variety of highly chelating N-aryl–substituted palladium(II)–bis(carbene) complexes [35] (Scheme 13) have been prepared in good yield, like 1,1′-bis(4-bromophenyl)-3,3′-methylene-diimidazoline-2,2′-diylidine-palladium(II) dibromide, 1,1′-bis(4-methoxyphenyl)-3,3′-methylene diimidazoline-2,2′-diylidene-palladium(II) dibromide, and 1,1′-bis(4-n-butoxyphenyl)-3,3′-methylenediimidazoline-2,2′-diylidine-palladium(II) dibromide (17–19) (Fig. 6). The catalytic activities of these Pd–NHC salts were tested for coupling of aryl chloride with olefins (Scheme 13). These complexes (19) were found to be highly selective in the CC coupling reaction.
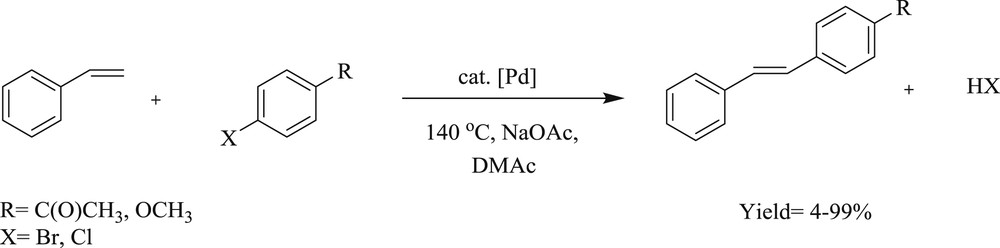
Mizoroki–Heck reaction: coupling of different arylhalides with styrene catalyzed by palladium–biscarbene complexes.
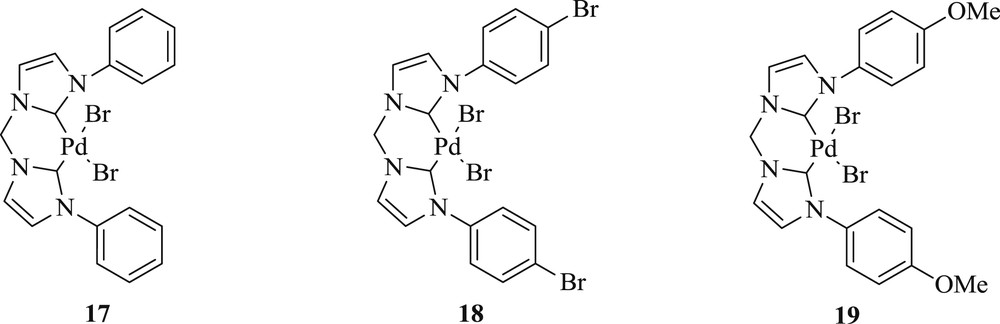
Palladium–biscarbene complexes 17–19.
Huynh et al. [36] testified bulky, benzannulated NHC–Pd(II) complex (Scheme 14) and established their ability in the CC bond formation (Scheme 15). However, the bulky ligand is believed to hinder a fast reductive formation of active Pd(0) species and subsequently lead to lower conversion as compared with the catalyst with less bulky benzimidazolin-2-ylidens.
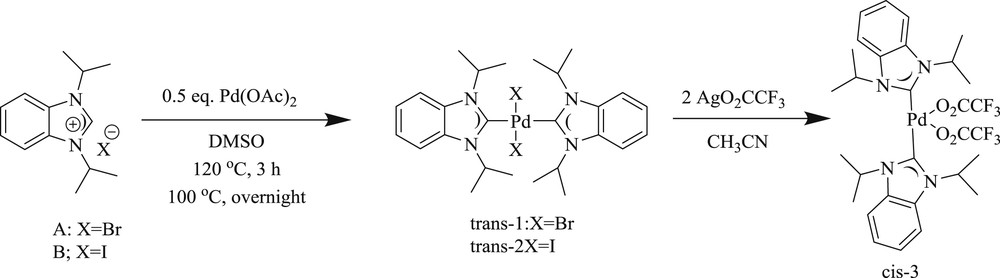
Synthesis of bis(carbene) Pd(II) complexes.

The Mizoroki–Heck reactions catalyzed by bis(carbene) Pd(II) complexes.
On the other hand, the task for coupling of three challenging substrates (Fig. 7) (Scheme 16) viz. steric (20), electronic (21), and catalyst poison (22) with tert-butyl acrylate has been effectively accomplished using an NHC palladacycle precatalyst [37]. As noted by the author, the complex can be synthesized on a large scale (Scheme 17) by a novel, one-pot, three-component reaction, which is stable to air, moisture, and long-term storage. The most selective bulky bromide (20) and the imidazole-derived bromide (22) displayed almost identical yields. A wide range of challenging substrates has been fruitfully coupled under a simple and user-friendly reaction protocol [37].
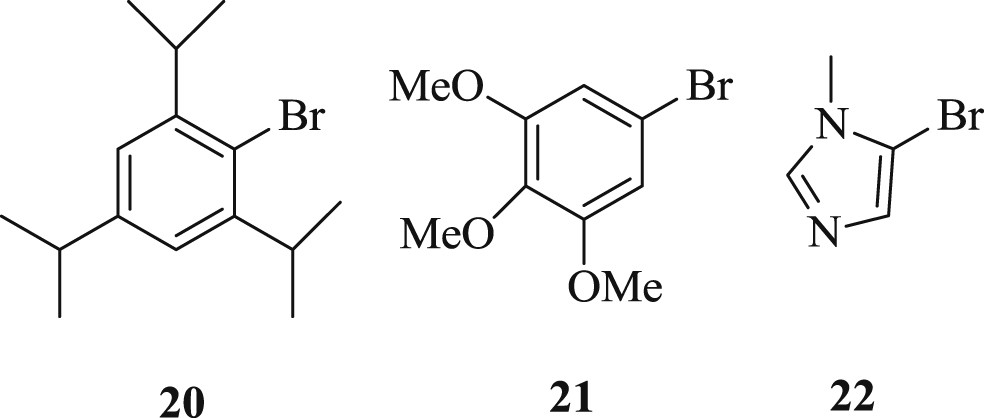
Sterically hindered substrates.

IMes–Pd(dmba)Cl-catalyzed Heck–Mizoroki coupling reaction.
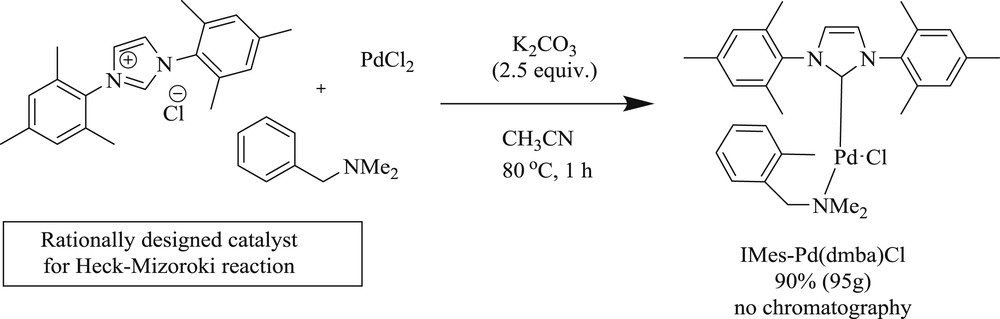
Synthesis of the IMes-Pd(dmba)Cl catalyst.
In the same year, another study demonstrated [38] the efficiency of bimacrocyclic NHC–Pd allyl salt (Fig. 8) obtained by transmetalation with allylpalladium chloride dimer from the NHC–Ag complex (23).
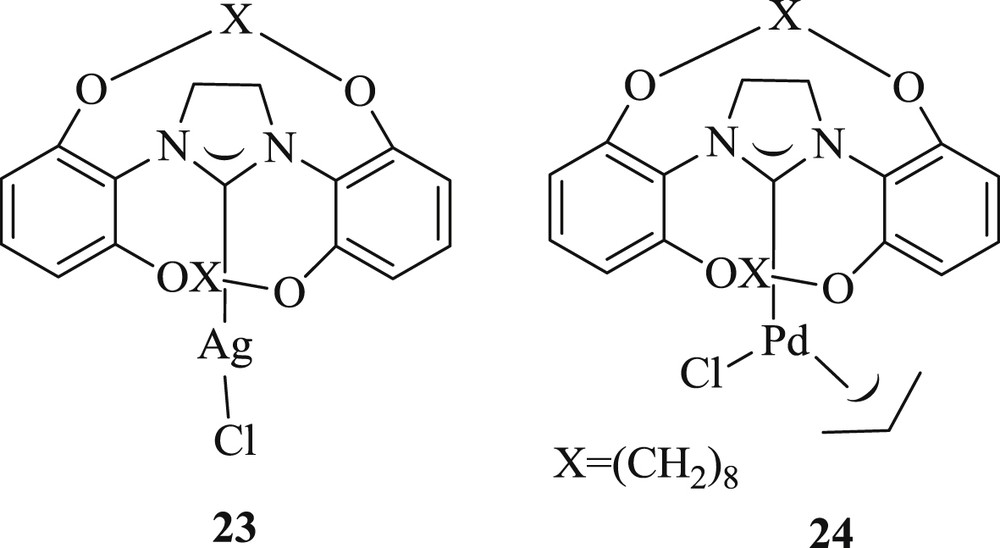
NHC–Ag 23 and NHC–Pd complex 24.
Analyzing the outcomes, complex (24) indicated better result in the Heck–Mizoroki reaction of aryl bromide with styrene, but a decrease in yield was observed when aryl chloride was used [38]. The bimacrocyclic complex (24) was also identified active at minute loading toward Suzuki–Miyaura cross-coupling reaction of inactivated aryl chlorides and bromides with 1-naphthalene–boronic acid. But the authors could not confirm anonymity in the formation of a tri-ortho-substituted biaryl with the same catalyst.
In another study, chiral palladium(II) salt (Fig. 9) developed [39] possessing a tridented carbene amidate alkoxide ligand assisted the formation of carbon–carbon and carbon–heteroatom bonds. This unique observation illustrated an asymmetric oxidative Heck-type reaction between aryl boronic acid and acyclic alkenes, which offered good to excellent yields (Scheme 18).
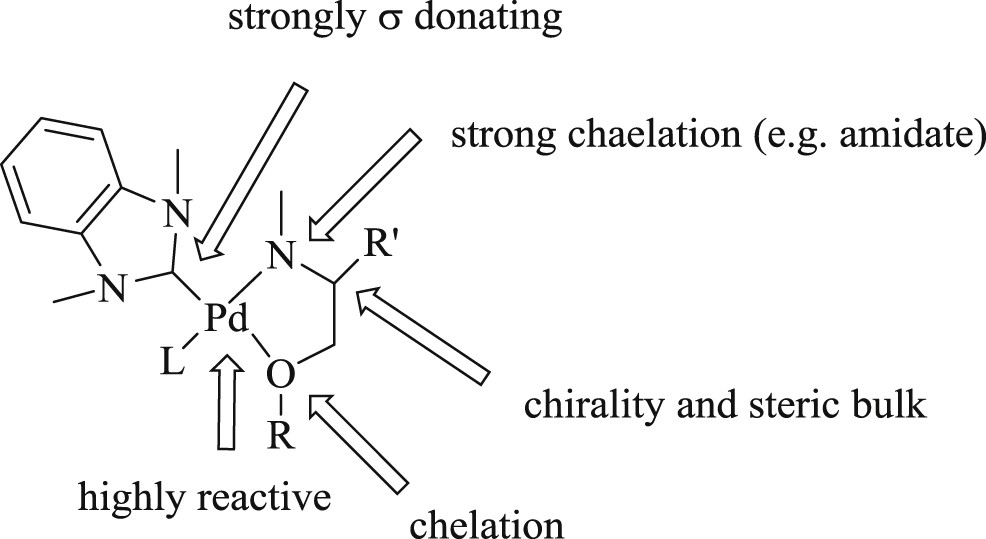
Chiral {Pd(OAc)2} complex.
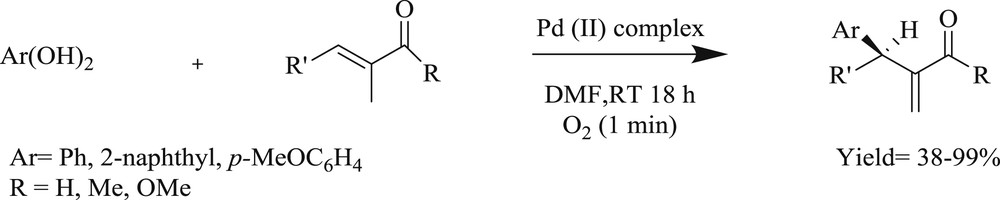
The catalytic oxidative Heck-type reaction of aryl boronic acids with acyclic alkenes.
Subsequently, methodology to synthesize [40] palladium(II) complexes containing pyrimidine-functionalized NHC-[Pd(L1)2(CH3CN)](PF6)2 (L1 = 1-n-butyl-3-(2-pyrimidyl) imidazolylidene) (Scheme 19) (26) and [Pd(L2)2](PF6)2 (L2 = 1-(2-picolyl)-3-(2-pyrimidyl) imidazolylidene) (Scheme 20) (28), have also been developed. The Pd(II)–NHC complexes were prepared via a carbene transfer reaction of [Ag(L1)2]PF6 (Scheme 19) (25) and [Ag2(L2)2](PF6)2 (Scheme 20) (27) with palladium salt, respectively. The complex (26) exhibited an unusual square-pyramidal structure, which represented the first example of the pentacoordinated Pd–NHC system. This palladium catalyst showed high performance for the Heck olefination of aryl bromides without additional ligands under mild condition. This approach is applicable to a wide variety of electron deficient and electron-rich substrates (Scheme 21).
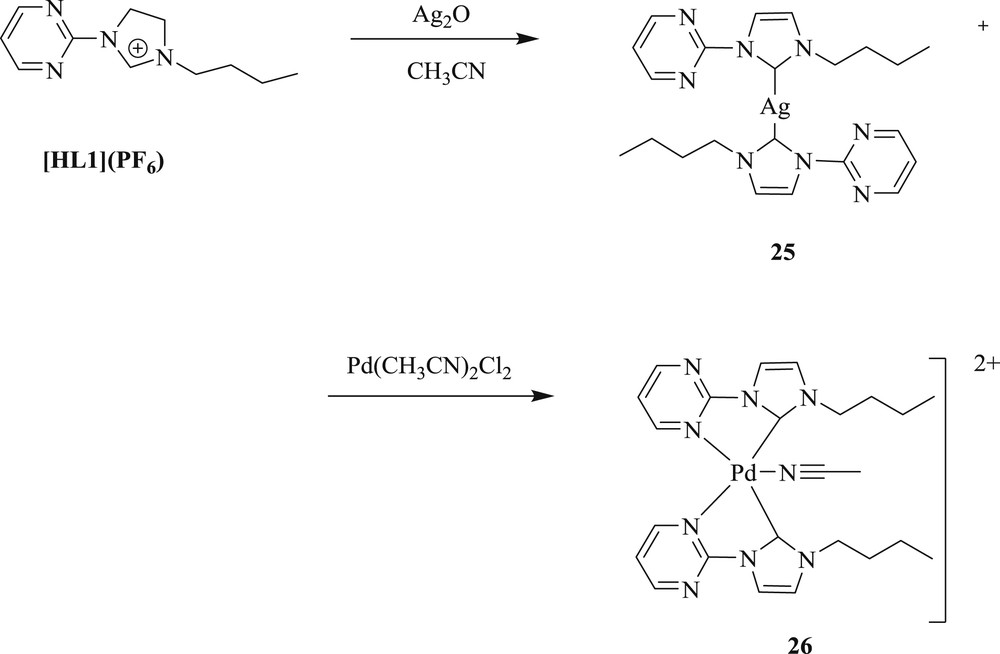
Synthesis of [Ag(L1)2]PF6 25 and NHC-[Pd(L1)2(CH3CN)](PF6)2 26.
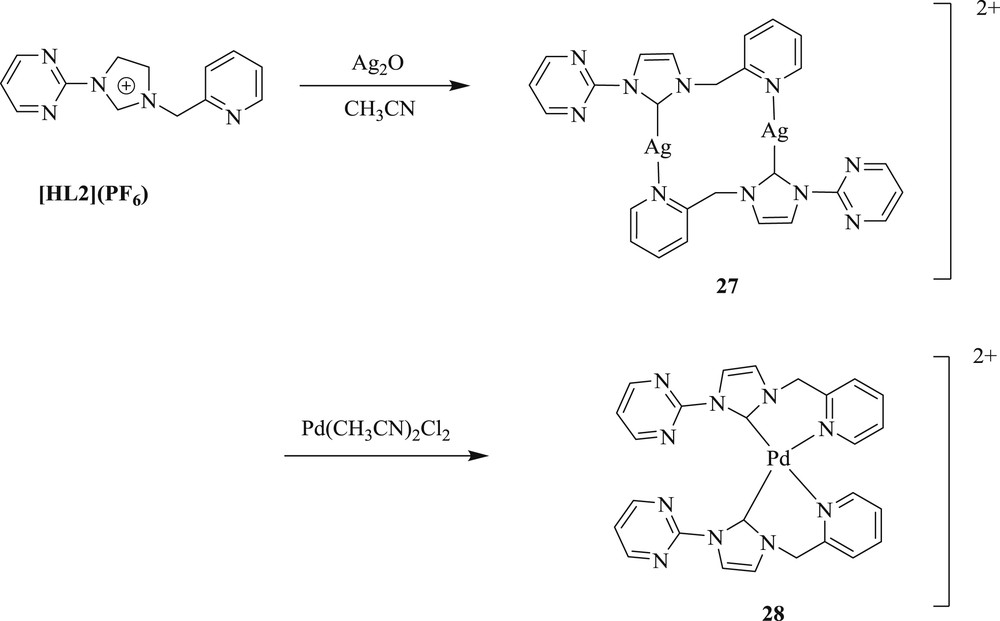
Synthesis of [Ag2(L2)2](PF6)2 27, [Pd(L2)2](PF6)2 28.
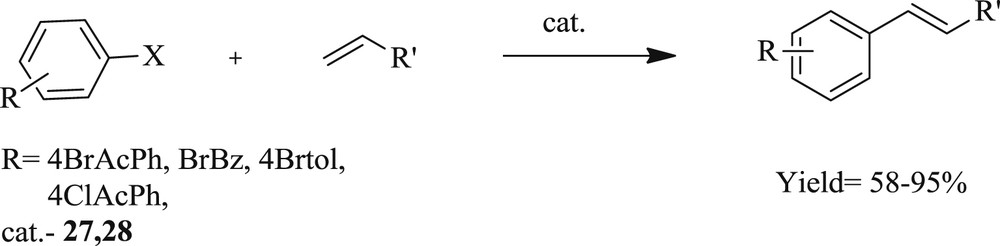
Heck coupling of aryl halides with olefins.
Jung et al. [41] developed a protocol using chiral dimeric tridented NHC-amidate-alkoxide-Pd(II) complexes (Fig. 10) (29–30). These complexes afforded an oxidative boron Heck-type reaction of aryl boronic acid with both acyclic (Scheme 22) and cyclic (Scheme 23) alkenes to the corresponding coupling product with remarkable enantioselectivities at room temperature. The study expanded the scope of a high degree of enantioselection because of the facial selection of alkenes, which was caused by counter axial groups (isopropyl and borate groups), surrounded by the possible transition states.
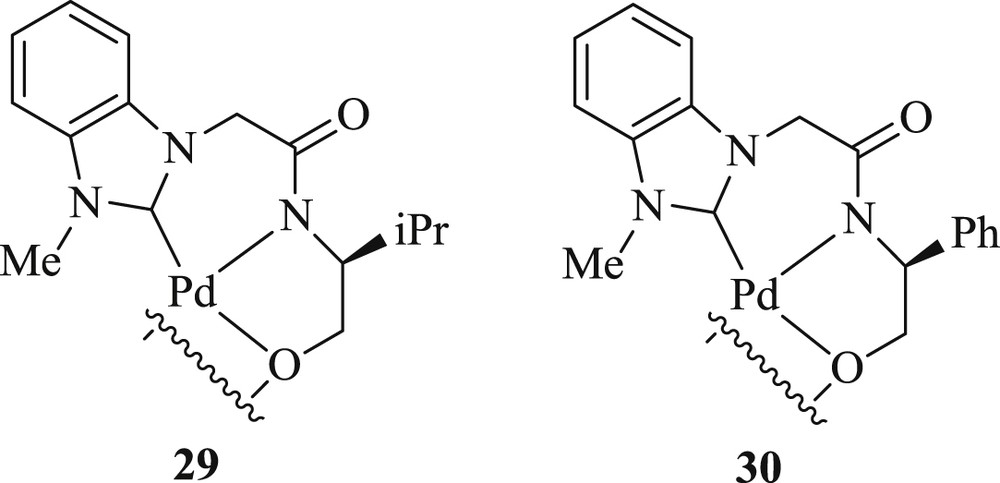
NHC–Pd 29 and 30.
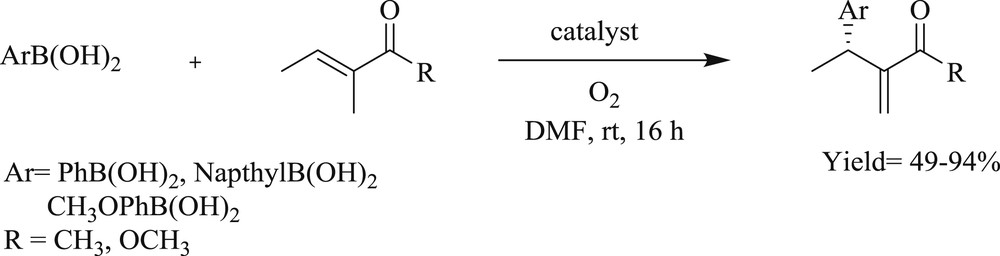
Asymmetric oxidative Heck reaction catalyzed by 29 and 30.
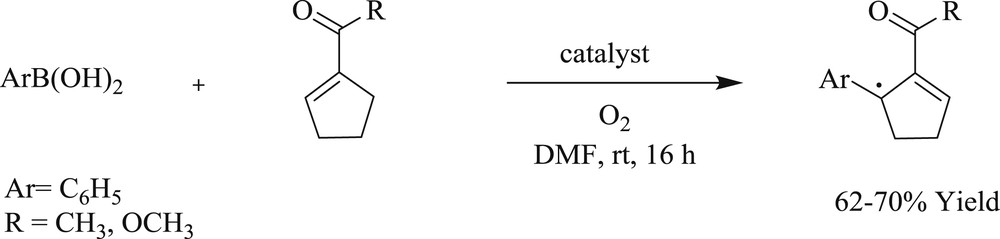
Asymmetric oxidative Heck reaction of aryl boronic acid with cyclic alkenes catalyzed by 29 and 30.
Another technique for the coupling reaction has also been investigated [42], which comprises mixed palladium(II) complexes (Fig. 11) with asymmetrically N,N′-substituted benzimidazoline-2-ylidene ligands. This procedure exhibited a better performance in the coupling reaction than those with symmetrically substituted NHC ligands. The highest reactivity was observed for cationic palladium(II) complexes (31a–d) (Fig. 11) bearing asymmetrically substituted NHC ligands in addition to diphosphane ligands (Scheme 24).
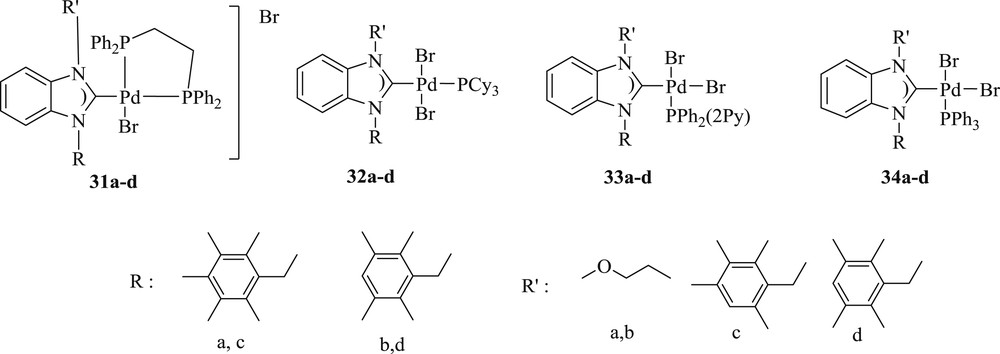
Palladium(II) NHC complexes 31(a–d)–34(1–d).

Palladium-catalyzed C–C coupling reaction of n-butyl acrylate with aryl halides.
Further investigation in this area reported [43] a double Mizoroki–Heck coupling reaction with aryl dibromide yielding diacrylate (Scheme 25) using palladium(II) mono and bis(carbene) complexes (Scheme 26) (34–35). All bis(carbene) complexes (31–36) were obtained as an inseparable mixture of square planar, trans–anti, and trans–syn rotamers.
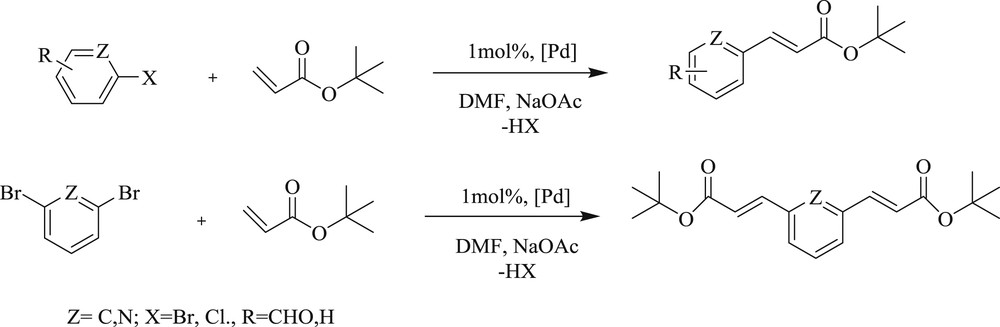
Mizoroki–Heck coupling reactions catalyzed by complexes 34 and 35.
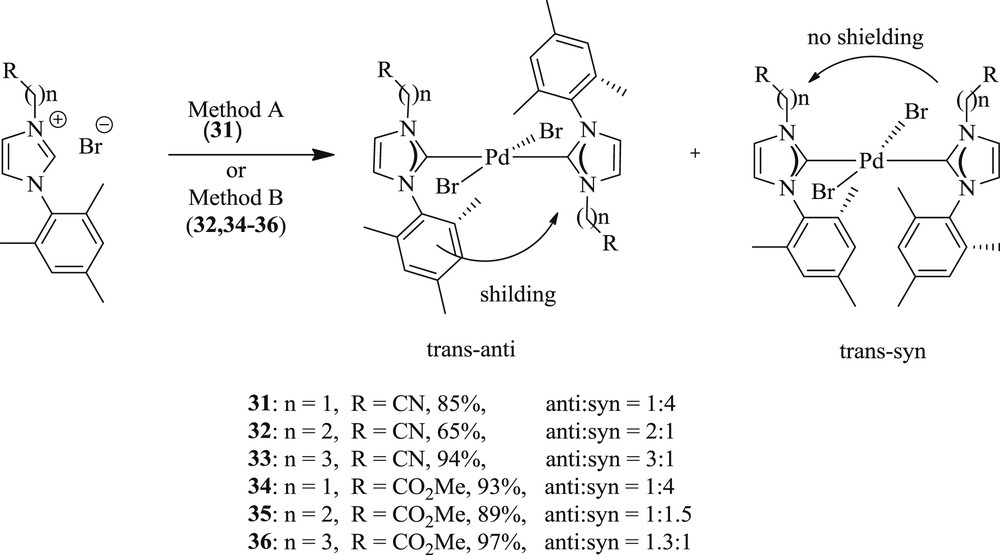
NHC–Pd(II) complexes 31–36.
Catalytic activity (Scheme 28) of bis, dimeric, and mixed Pd–NHC complexes (Scheme 27) has been further confirmed (37–44) by Gulcemal et al. [44]. It was found that both the longer oligoether and bulky benzyl substituent on the N atom and the methyl group at the 5- and 6-position of a benzimidazole ring gave an enhanced reaction rate. On the basis of these results, it is reasonable to assume that the interaction between the oxygen atoms of the oligoether palladium center is playing an important role in coupling reactions.

The Heck coupling reaction of styrene with aryl bromide catalyzed by Pd–NHC.
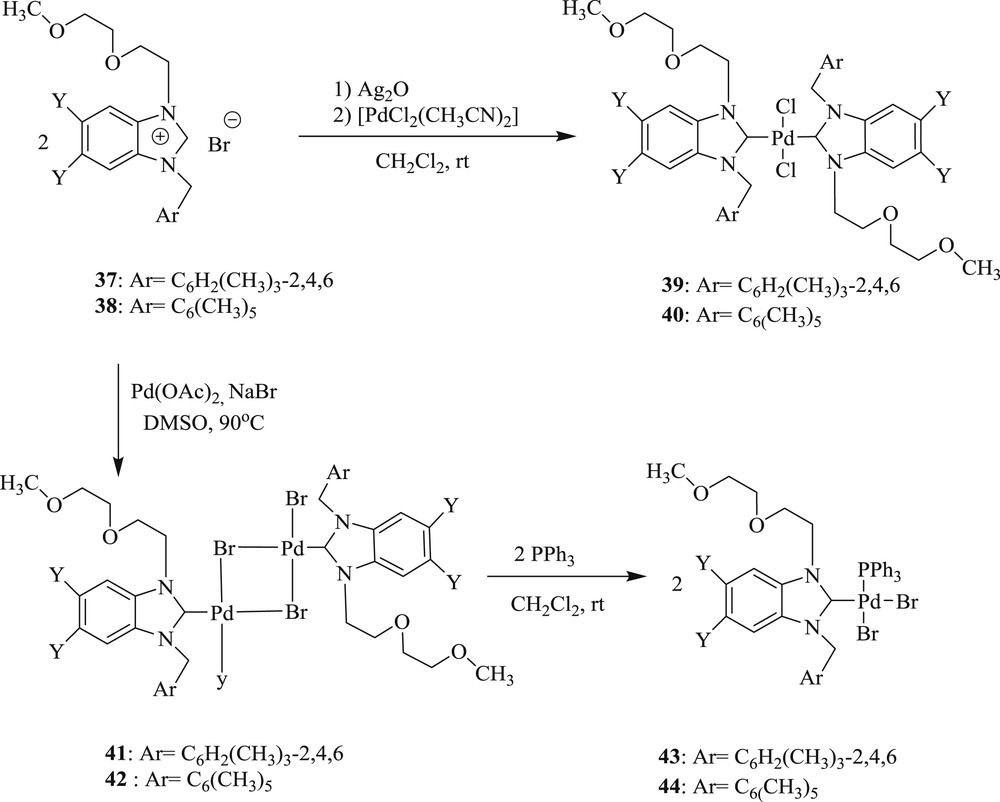
Synthesis of NHC–Pd(II) complexes 39–44.
Wang and Liu [45] described the synthesis of an imidazole-coordinated dinuclear monodente NHC–Pd complex (Fig. 12) (45) and cis-chelated bidentate NHC–metal complex (46) from the novel bis(imidazolium) salt. These catalysts were found competent in the Heck reaction (Scheme 29), under very mild conditions for bromoaryl and iodoaryl substrates. However, they were inactive toward chloroaryl substrates.
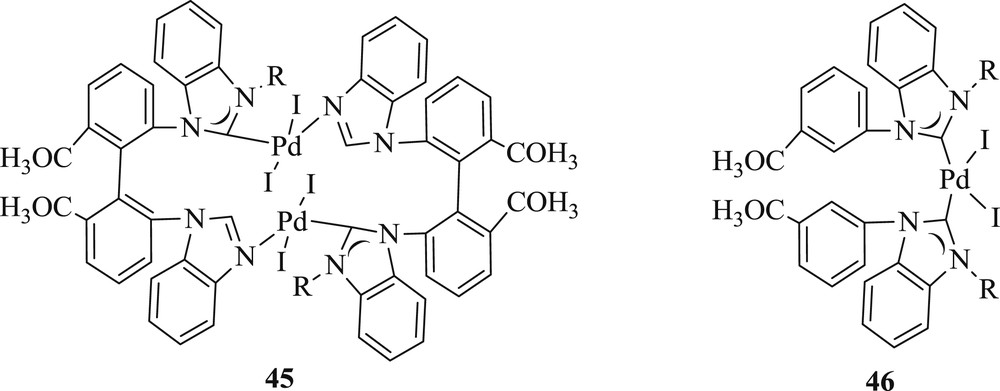
Monodentate and bidentate NHC–Pd complexes.
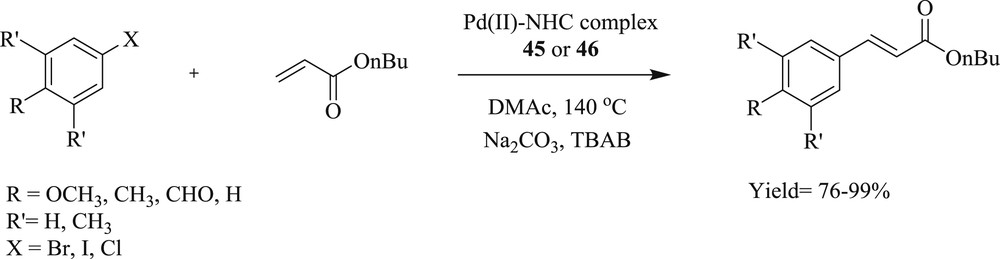
Heck reaction catalyzed by Pd(II)–NHC complexes.
Another notable study developed [46] the Heck–Mizoroki reaction (Scheme 30) mediated by cyclopalladated N,N′-dimethylbenzylamine ligated with NHC-1,3-bis(mesityl) imidazole-2-ylidine (IMes) (Fig. 13). It tolerated a variety of aryl- and heteroaryl bromide with good to excellent yields in low catalyst loading at ambient conditions. Electron rich m- and p-substituted aryl bromide produced diarylated products entirely, whereas electron-poor aryl bromides resulted predominately in monoarylated products.

Heck–Mizoroki couplings of aryl bromides with tert-butyl acrylate mediated by 47.
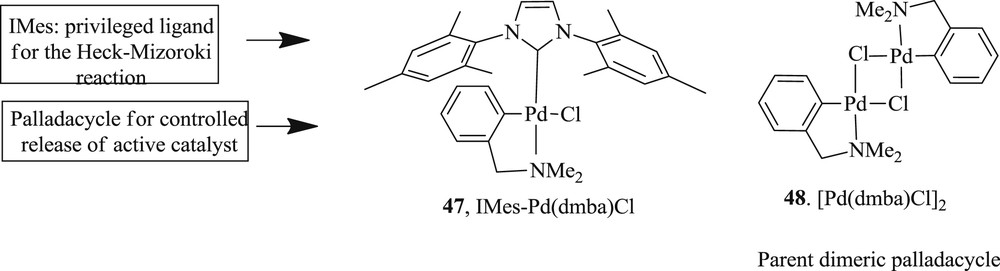
IMes–Pd(dmba)Cl 47, [Pd(dmba)Cl]2 48.
Significantly, another group acquired [47] novel pseudopincer and Carbon–Sulfur–Carbon (CSC) pincer-type Pd(II) complexes (Fig. 14) derived from benzannulated NHC. These systems were proficient for the CC coupling of activated and deactivated aryl bromides in considerable yield (Scheme 31) without poisoning the active catalyst.
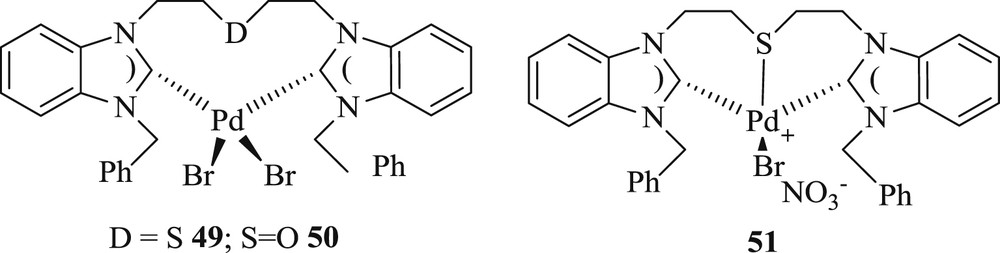
Benzimidazole-based CSC pincer-type Pd(II) complexes.

Mizoroki–Heck coupling reactions catalyzed by complexes 49–51.
Interestingly, a series of di-NHC ligands with alkyl bridge of different chain lengths (n = 2–4) and their bipalladium complexes (52–54) (Fig. 15) have been prepared as well [48]. The influence of the different bridges on the structure and reactivity of the complexes was successfully tested in reactions (Scheme 32) of styrene and bromobenzene. The complex with two- and four-carbon linker gave higher yield whereas the one with three-carbon linker revealed better regioselectivity.

Bi-palladium di-NHC complexes 52–54.
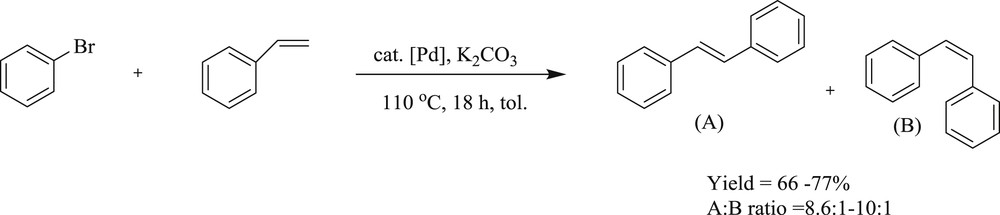
The Heck reaction catalyzed by bi-palladium di-NHC complexes.
At the same time, a series of amino-salicylaldimine–Pd(II) complex (Scheme 33) bearing 5-methyl-3-(R-1-ylmethyl)-salicylaldimine ligands (R = morpholine, piperidine, pyrolidine, 4-methylpiperrazin, diisopropylamine) were also obtained (25a–e) [49]. These palladium complexes provided a unique combination of activity in Heck and Suzuki reactions using DMF as a solvent (Scheme 34).
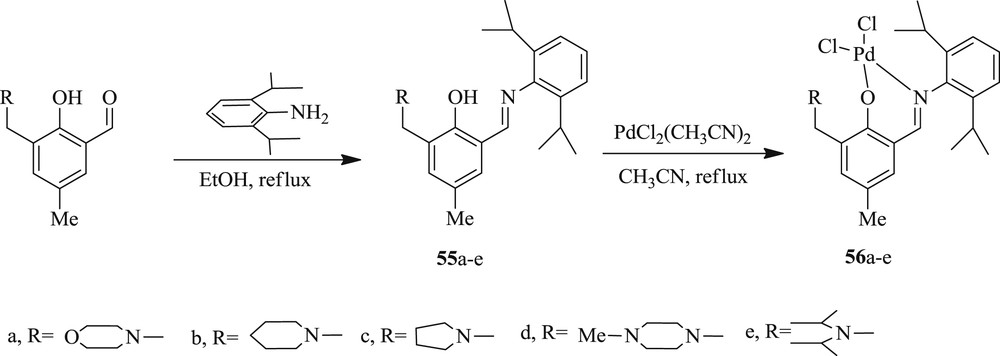
Synthesis of amino-salicylaldimine ligands 55a–e and of their Pd(II) complexes 56a–e.
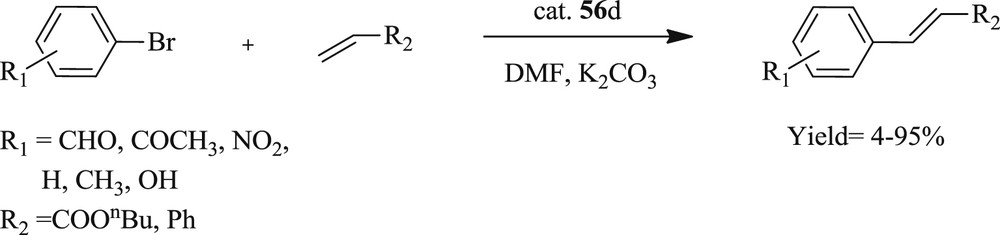
Heck cross-coupling reactions of aryl bromide with styrene or n-butyl acrylate.
A heteroleptic palladium(II) complex containing bidentate carbene/amido ligands and 3-(trifluoromethyl)-5-(2-pyridyl) pyrazolate (Fig. 16) was developed [50]. The complex tolerated challenging aryl halide including deactivated aryl chloride in coupling reactions with a minimum loading of 0.5 mol % in the presence of TBAB under conventional and microwave conditions (Scheme 35).
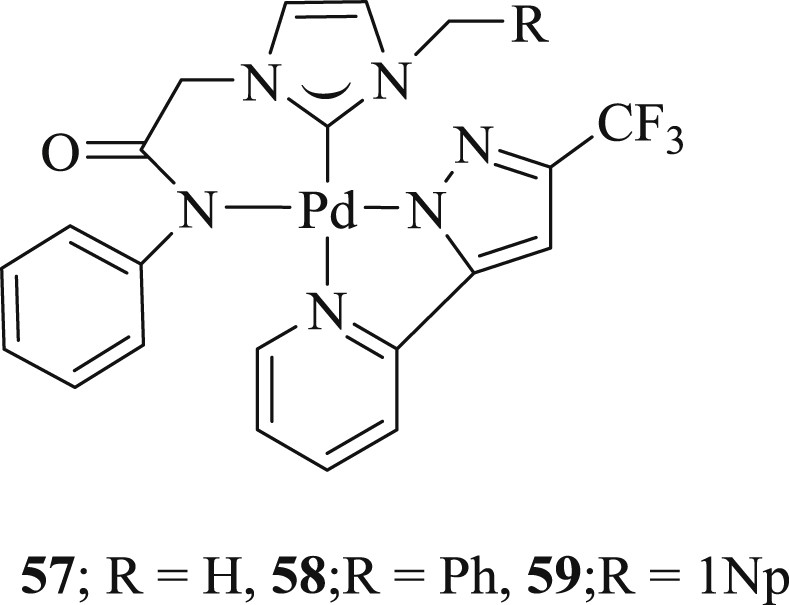
Bidentate palladium complexes 57–59.
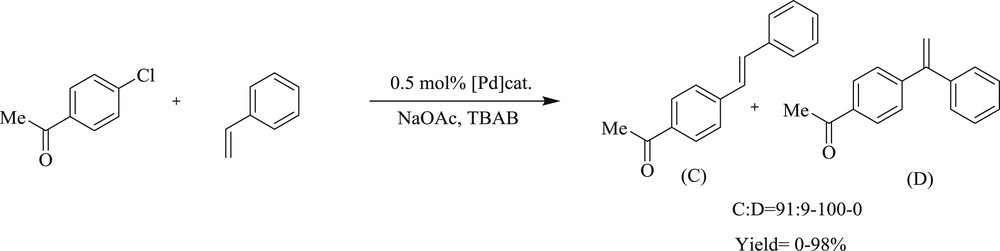
Mizoroki–Heck reaction catalyzed by bidentate palladium complexes.
It was specified by Shao et al. [51] that NHC–Pd(II) salt (Fig. 17) derived from proline is a highly stable and capable catalyst in the Mizoroki–Heck reaction of aryl bromides and iodides performed in water. The reaction tolerates various functional groups in the substrate and gave corresponding alkenes in appreciable yield (Schemes 36 and 37).
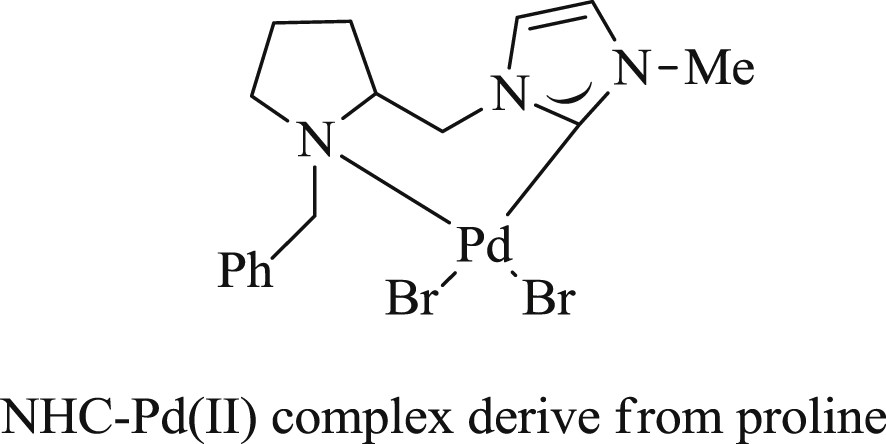
NHC–Pd(II) complex derived from proline.
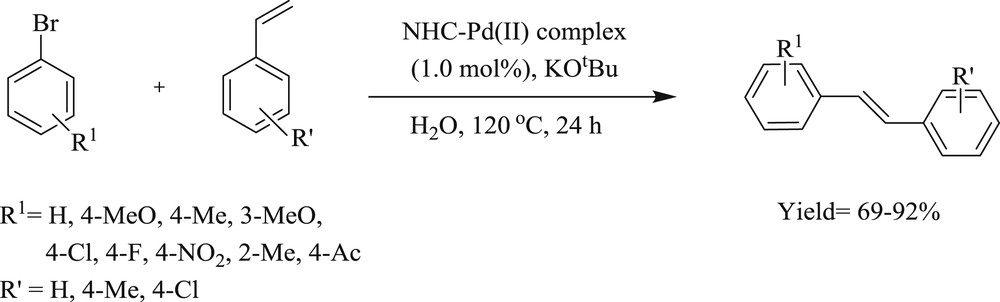
Reactions of aryl bromides with styrenes catalyzed by NHC–Pd(II) complexes.
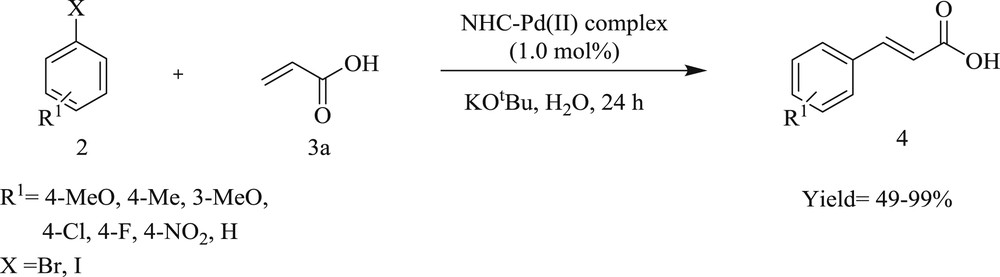
Reaction of bromo/iodobenzene with acrylic acid catalyzed by NHC–Pd(II) complexes.
The reaction of aryl bromide with tert-butyl acrylate was carried out using 1,2,3-triazol-5-ylidine–palladium complex (Fig. 18) [52], with the good conversion of alkenes (Schemes 38 and 39). These systems (60–62) were proficiently applied for electron rich, electron poor, and functionalized aryl bromides and alkenes as well as acrylates.
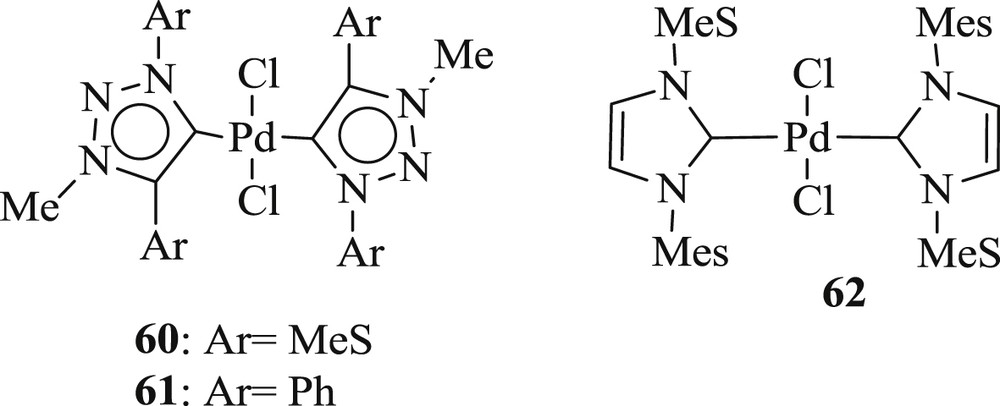
trans-Bis(1,2,3-triazol-5-ylidene)palladium (60, 61) and trans-bis(imidazol-2-ylidene)palladium (62).
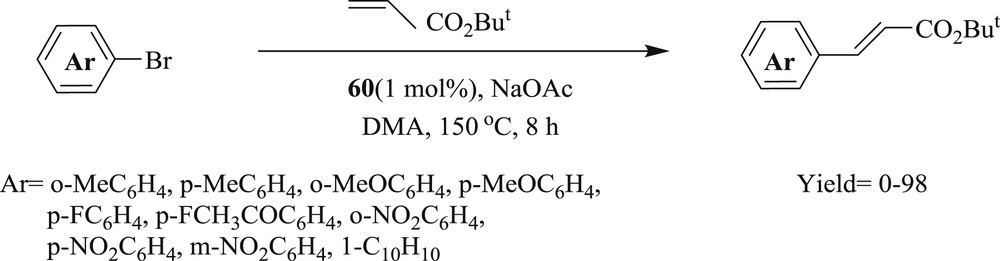
Mizoroki–Heck reaction with aryl bromides.

Mizoroki–Heck reaction with aryl chlorides.
Furthermore, in another study, a simple way to synthesize bis(NHC)–Pd catalyst (Fig. 19) derived from caffeine has been demonstrated [53]. The scope of this method has been extensively applied in the aqueous medium for cross-coupling of Mizoroki–Heck, Sonogashira, and Suzuki reactions (Scheme 40).
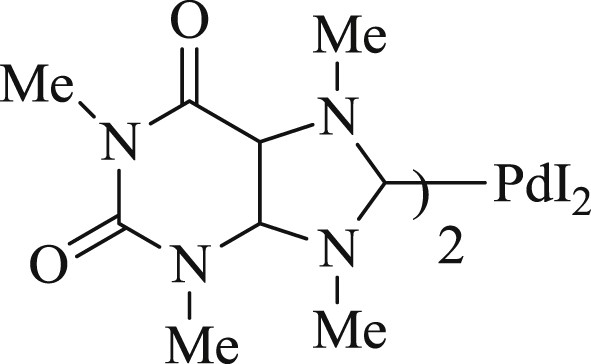
Bis-NHC–Pd complex.

Mizoroki–Heck reaction using the bis-NHC–PdI2 catalyst.
Notably, in the same issue, synthesis of six homo-dicarbene–Pd(II) salts (Fig. 20) bearing imidazole (63–65) and benzimidazole-derived carbene (66–68) with C1–C3 alkyl bridges have been described [54]. Among these, benzimidazole-2-ylidine showed the highest conversion in CC coupling compared with their imidazoline-2-ylidines because of the absence of acidic protons on the heterocycle. On the basis of these results, two Pd(II) complexes (69 and 70) of new propylene-bridged heterodicarbenes, containing both imidazole- and benzimidazole-derived NHCs, have been synthesized. Their capability (Scheme 41) was superior to that of homo-dicarbene complexes, which may be traced back to an electronic symmetry induced by the unusual heterodicarbene ligands.
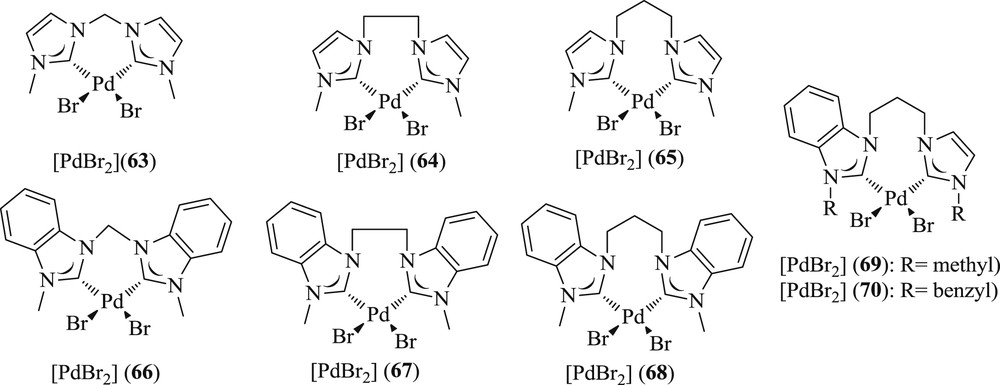
Pd(II)–diNHC complexes 63–70.

Mizoroki–Heck coupling reactions catalyzed by Pd(II)–diNHC complexes 63–70.
Another range of new six- and seven-membered expanded ring NHC complexes of zerovalent palladium dvtms (divinyltetramethyldisiloxane) (71–77) (Scheme 42) have been reported [55]. These spectacular precatalysts [Pd(NHC)(dvtms)] are evaluated for the coupling of 4-bromoacetophenone and n-butyl acrylate (Scheme 43). It was found that ring size and steric hindrance display the greatest performance of six-membered analogues compared with the five-membered NHC.
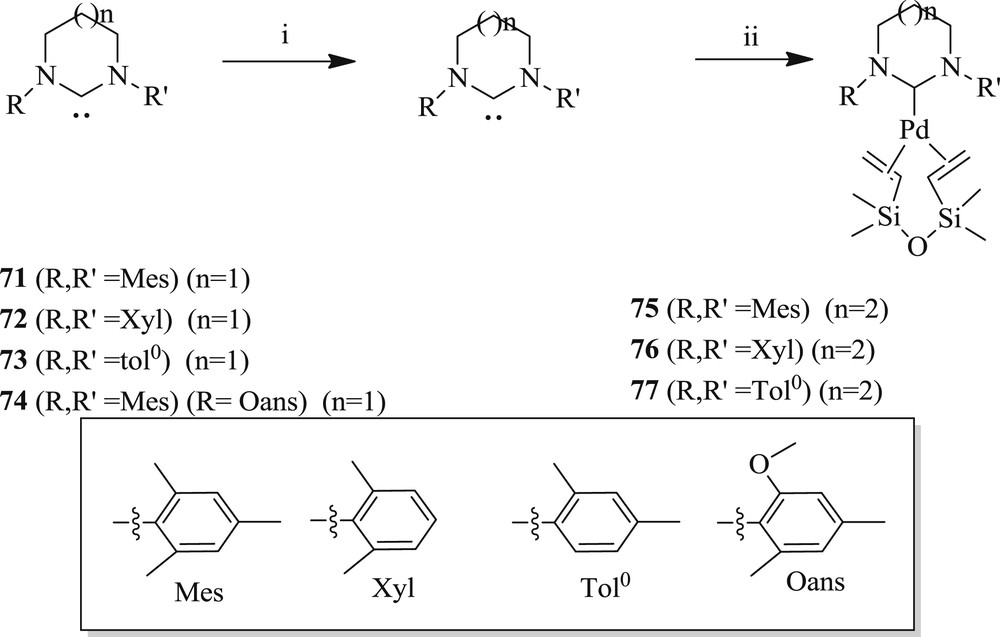
Synthesis of NHC–palladium(0) dvtms complexes 71–77.

Mizoroki–Heck coupling of 4-bromoacetophenone with n-butyl acrylate.
The synthesis of new dinuclear-heterocarbene–Pd(II) systems (Fig. 21) bearing triazolidine diylidine bridge and terminal benzimidazoline-2-ylidene have been illustrated as well [56]. The best results were achieved in the Mizoroki–Heck reaction (Scheme 44), which exposes the superiority of these systems compared with (Fig. 21) the previously examined mononuclear Pd(II)–bis(carbene) complex.
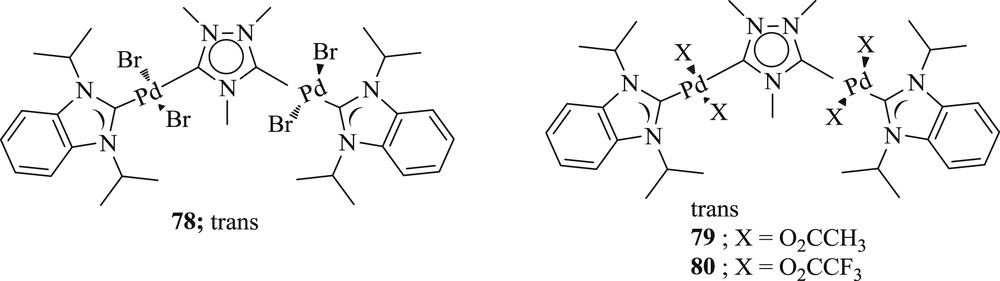
Tetracarboxylato-heterocarbene Pd(II) complexes 78–80.

Mizoroki–Heck coupling reactions catalyzed by complexes 78–80.
Cao et al. [57] have developed a series of dicarbene dipalladium complex with different N-substituted imidazolylidene for the coupling of styrene with bromobenzene. In this protocol, the complex with aryl substituents attached to the nitrogen atoms of the NHC ring has better activity than alkyl substituents. The various substituents on the phenyl group in complexes (83–85) (Scheme 45) have a little effect on both the regioselectivity and yield of the products.
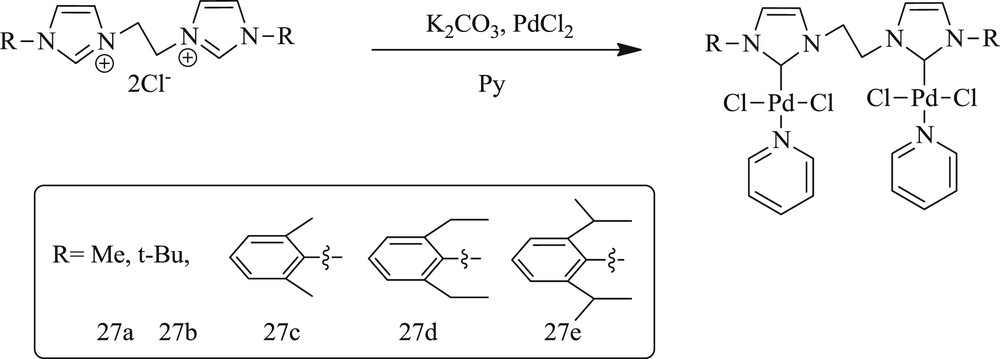
Synthesis of the dipalladium bis-NHC complexes 27a–27c.
Notably, the same author identified [58] a series of di-NHC dipalladium complexes Pd2LPy2Cl4 (L = LC3, LC5, LC6, and LC10) with alkyl bridges of different chain lengths. The activity of this method was successfully tested for the coupling of styrene with aryl bromides. The length of the bridged ligands (Fig. 22) had a considerable effect on the yield and regioselectivity (Scheme 46).
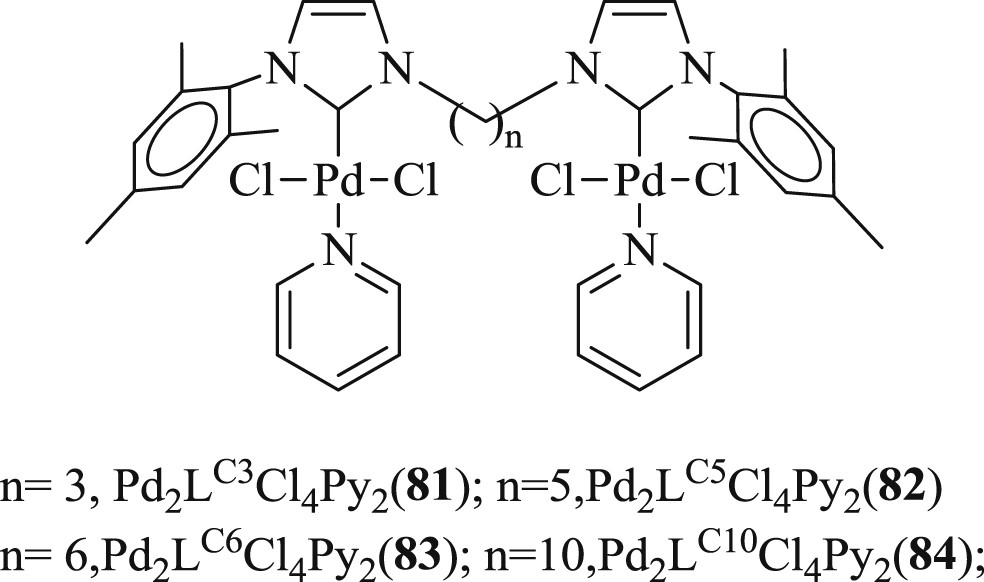
di-NHC dipalladium complexes 81–84 with different alkyl bridge lengths.
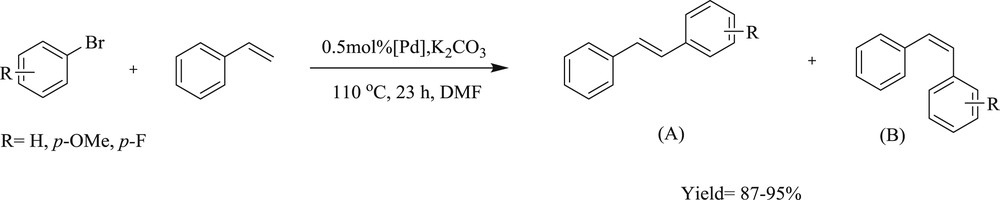
Mizoroki–Heck reaction catalyzed by 81–84.
A series of differently substituted Chugaev-type palladium bis(acyclic diamino carbene) systems have also been scrutinized (Scheme 47) [59]. All these systems were productively used in Heck reactions at 120 °C for both activated and deactivated aryl bromides in the presence of the mild base NaOAc with excellent yields (Scheme 48). Conversely, other bases like anhydrous K3PO4, Cs2CO3, and NEt3 were found less effective as base additives toward the formation of stilbene.
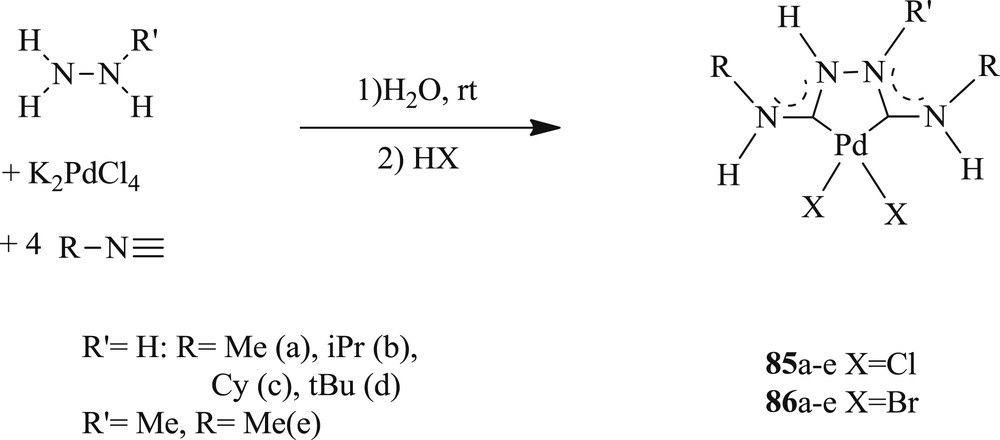
Modular synthesis of Chugaev-type palladium bis(ADC) complexes 85a–e and 86a–e.

Mizoroki–Heck coupling reactions with 86b.
Furthermore, a new approach [60] as single NHC ligands, 1,3-bis (2,4,6-trimethylphenyl) imidazolium chloride (87) (Fig. 23) has been identified for one-pot hydroacylation–Sonogashira, Heck (Scheme 49), Suzuki coupling under mild conditions. The reaction involves the use of 2-,3-,4-iodobenzaldehyde as an acyl donor that allows the subsequent reaction in the same pot facilitated by NHC ligands. With its multiple bond-formation abilities, the present concept represents an attractive option for the rapid construction of compound libraries based on small molecules.
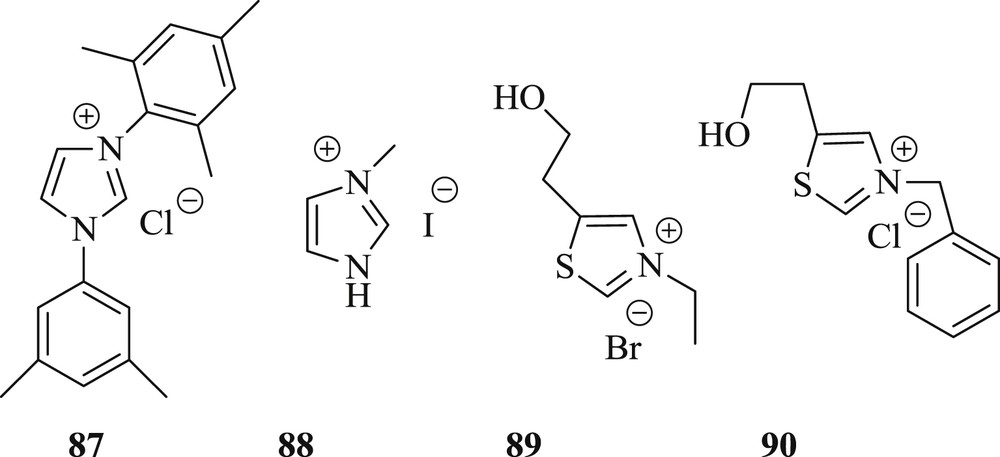
N-heterocyclic salts as a source of NHC 87–90.
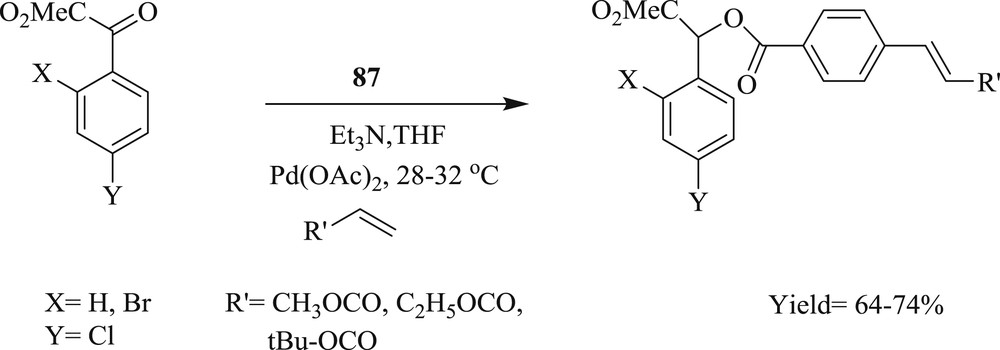
NHC-mediated hydroacylation–Heck coupling.
A series of 1-(cyclobutylmethyl)-substituted imidazolidinium/benzimidazolium salts (Fig. 24) have been synthesized [61] and studied in situ with Pd(OAc)2 for the reaction of an aryl halide with styrene in the presence of aqueous potassium hydroxide (Scheme 50). The advantages offered by this method are simple procedure and mild condition.
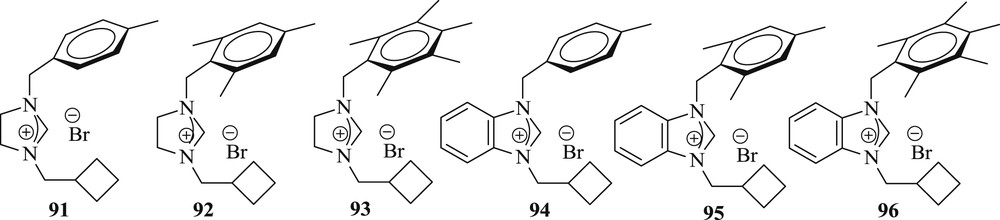
NHC ligands.

Heck coupling of 4-bromoacetophenone and styrene in water.
Simultaneously, the synthesis of 1-(pyridine-4-ylmethyl) NHC and their Pd(II) and Ag(I) complexes [62], have been effectively achieved (101–103) (Fig. 25). The prepared catalysts have been investigated in the Mizoroki–Heck reaction with low loading and the catalysts also tolerated a broad range of substrate with electron donating groups (Scheme 51).
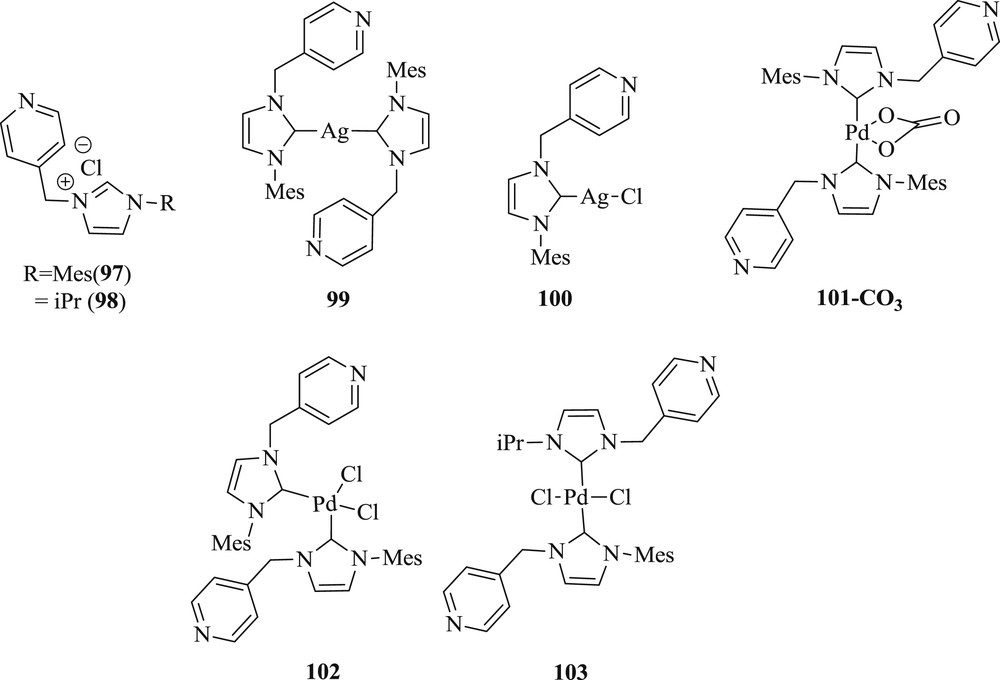
Ag–NHC and Pd–NHC complexes 97–103.
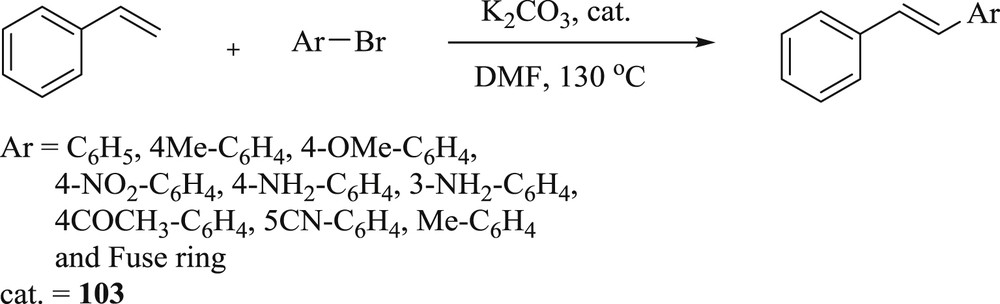
Heck–Mizoroki catalytic reaction with different aryl bromides.
Liu et al. [63] have introduced a new functionalized NHC–Pd(II) compound by the reaction of NHC–Ag transmetalation reagents with PdCl2(CH3CN)2 (Fig. 26). The catalyst provided significant yields of alkenes by the reaction of various aryl bromides with styrene in water at 110 °C (Scheme 52). However, the method also showed effective performance in the Suzuki–Miyaura reaction except for the challenging chloro derivatives.
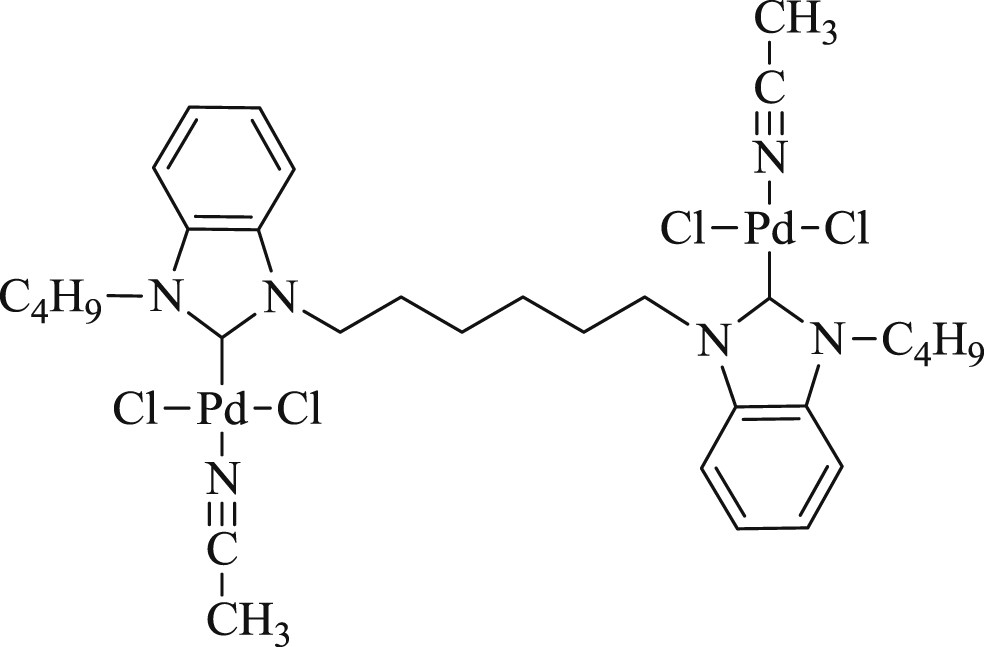
Pd–NHC complex.
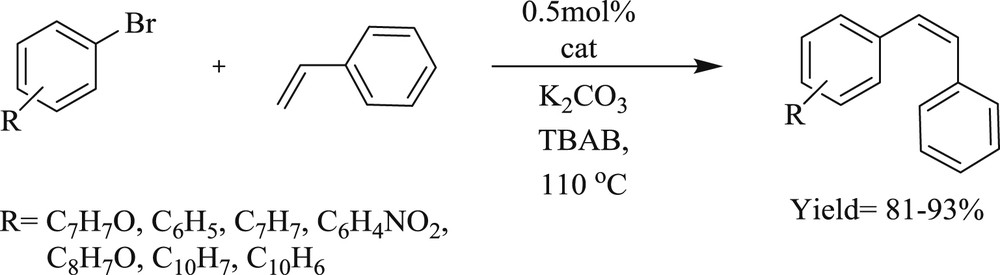
Mizoroki–Heck coupling reactions catalyzed by Pd–NHC complexes.
The approach of the dicarbene dipalladium complex (Fig. 27) mediated the mono- and the double-Heck reaction of terminal olefins with aryl halide has been proved to be a powerful method to form monoaryl- and diaryl-substituted alkene derivatives [64]. The phosphine-free diarylation reaction of terminal alkenes can be performed in the presence of TBAB as additives and NaOAc as a base with higher catalyst loading (1 mol %) in DMA at 120 °C (Scheme 55). The protocol is applicable to aryl iodides and bromides leading to symmetrical and unsymmetrical β,β-diarylated alkenes in appreciable yields (Schemes 53 and 54).
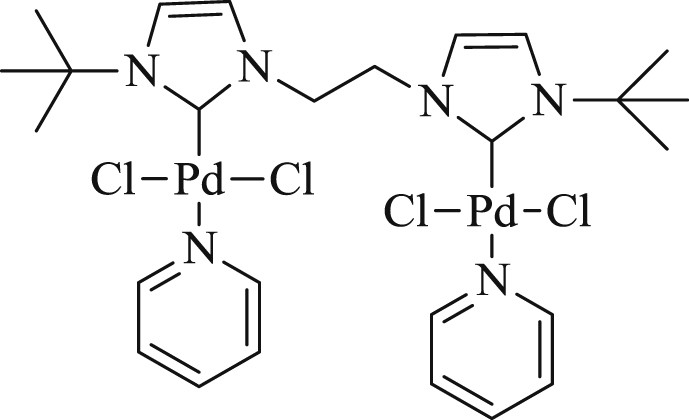
PEPPSI–Pd–NHC catalyst.
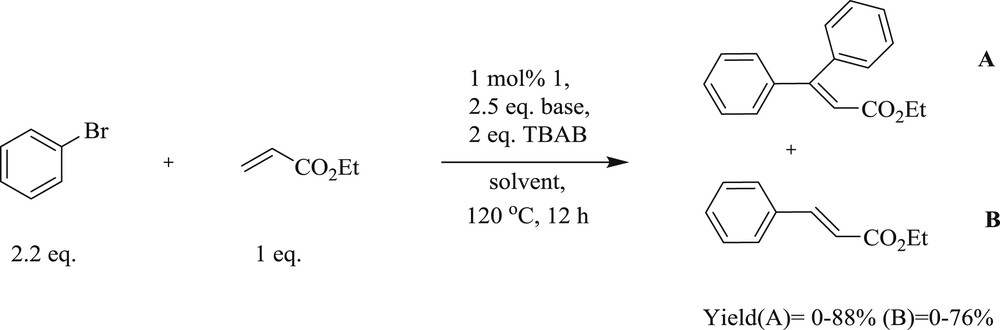
Diarylation reaction of ethyl acrylate.
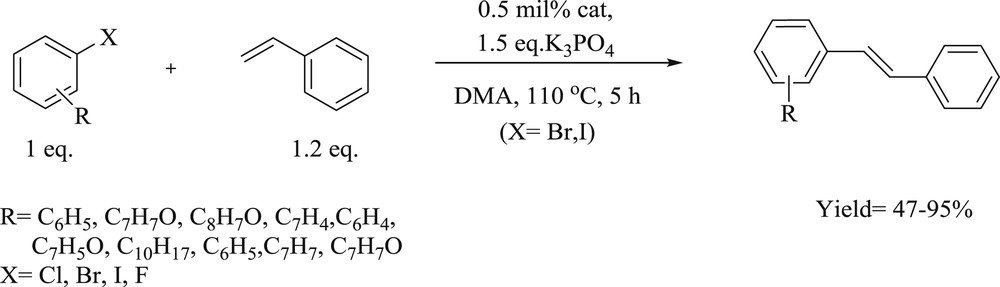
Mizoroki–Heck cross-coupling reactions of styrene with aryl halides.
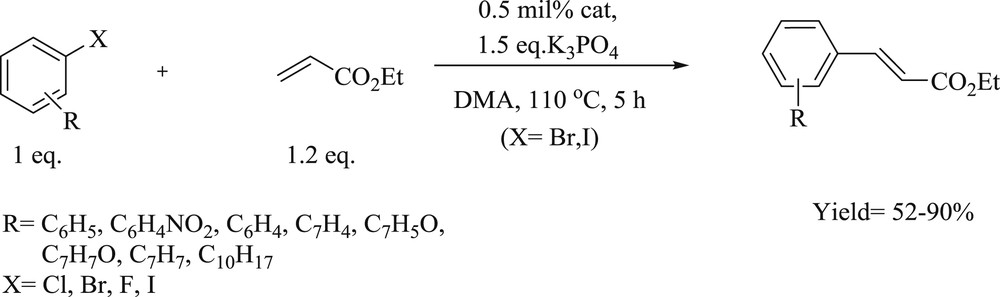
Mizoroki–Heck cross-coupling reactions of ethyl acrylate with aryl halides.
By using [Pd(μ-Cl)-Cl(SIPr)]2 (SIPr = {N,N-bis[2,6-(diisopropyl)phenyl]imidazolidine-2 ylidine}) salt (Fig. 28) (20–200 ppm), Cazin et al. [65] introduced a highly efficient mode of the Mizoroki–Heck reaction (Scheme 56) involving a range of aryl and heterocyclic bromides.
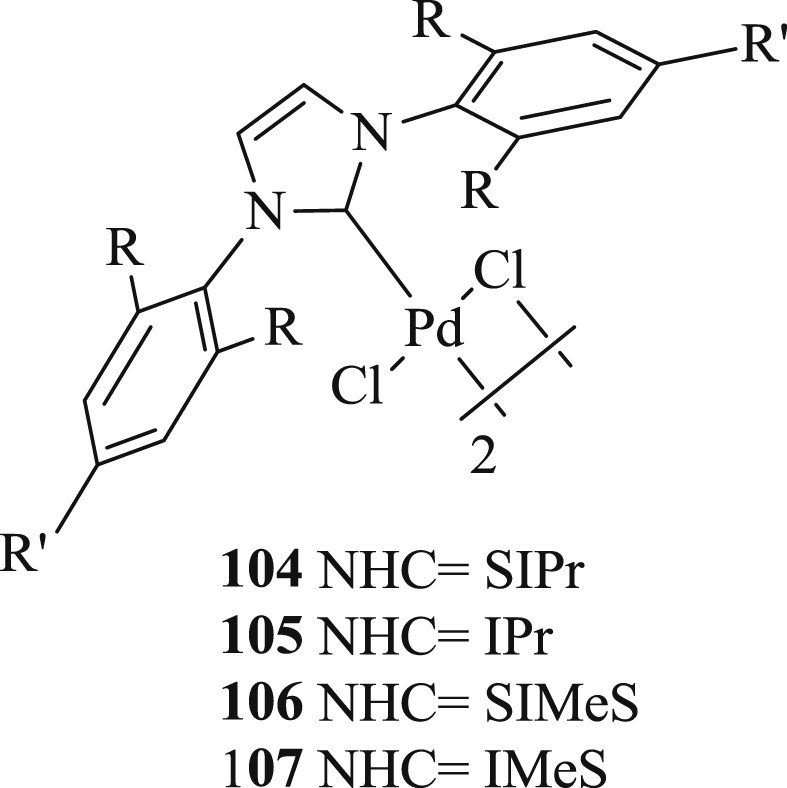
Dimeric Pd–NHC complexes 104–107.
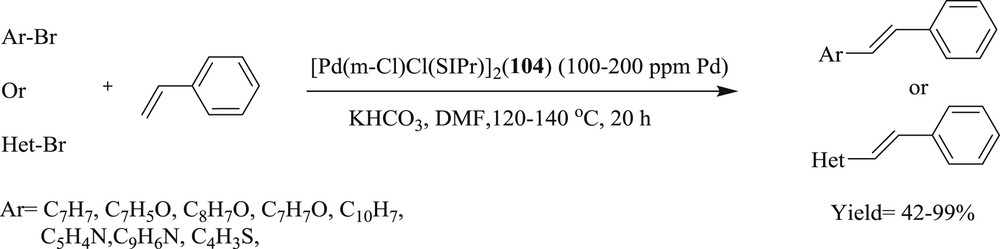
Coupling of styrene with aryl and heterocyclic bromides.
This pioneering work designed six pyridine-enhanced precatalyst preparation stabilization and initiation (PEPPSI) complexes [66], [PdBr2(NHC)(Py)] (108–119) (Fig. 29), from substituted imidazolium or benzimidazolium salts, and also provided an efficient system for the cross coupling reactions (Scheme 57). Benzimidazole-derived compounds exhibited better reactivity at 0.1 mol % loading compared with imidazole-based analogues. Formation of palladium nanoparticles (NPs) in the reaction mixture was evidenced by dynamic light scattering, transmission electron microscopy studies, and mercury poisoning experiment, suggesting the possible participation of palladium NPs during the reactions.
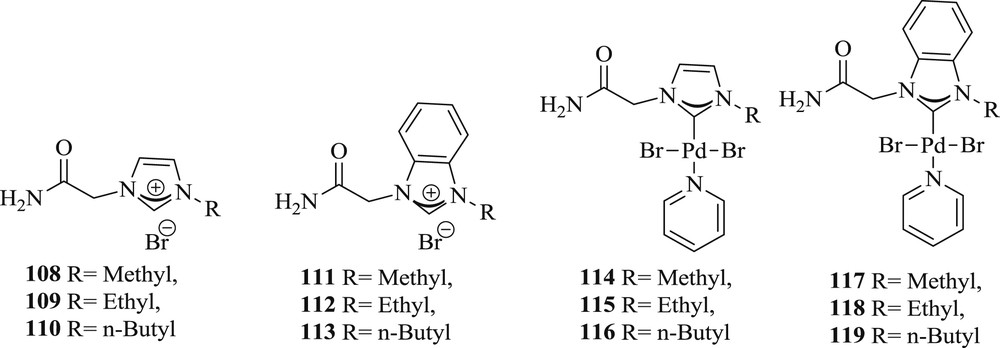
NHC ligands 108–113 and PEPPSI–Pd–NHC complexes 114–119.

Mizoroki–Heck reaction of aryl chlorides and styrene.
In addition, a study of alkyl bridges of different chain lengths on the catalytic activities of various imidazole- and benzimidazole-based di-NHC dipalladium complexes was demonstrated [67]. The authors concluded that the length of linker affects the catalytic activity of these complexes. Imidazole-based complex (Fig. 30), with the shortest ethylene bridge, exhibited good activity and selectivity in both mono- and double-Heck coupling reactions (Schemes 58 and 59).
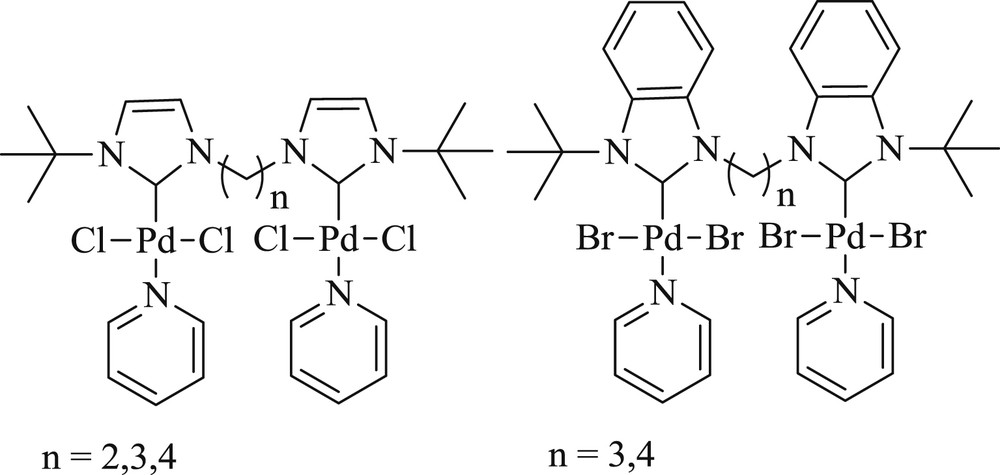
NHC–palladium complexes.

Monoarylation of styrene catalyzed by palladium complexes.
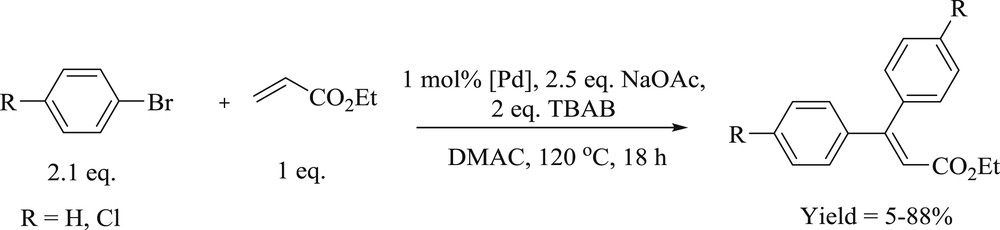
Diarylation of ethyl acrylate catalyzed by palladium complexes.
Subsequently, another group effectively synthesized and characterized two novel Pd(II)–NHC complexes bearing benzimidazole and pyridine groups [68]. The structure of palladium complexes was confirmed as a typical square planar, with palladium surrounded by two pairs of trans-arranged benzimidazole and carbene ligands. The study found that Pd–NHC complexes (Fig. 31) show a better performance in the Mizoroki–Heck (Scheme 60) coupling reaction of aryl halides with various substituted acrylates under moderate conditions.
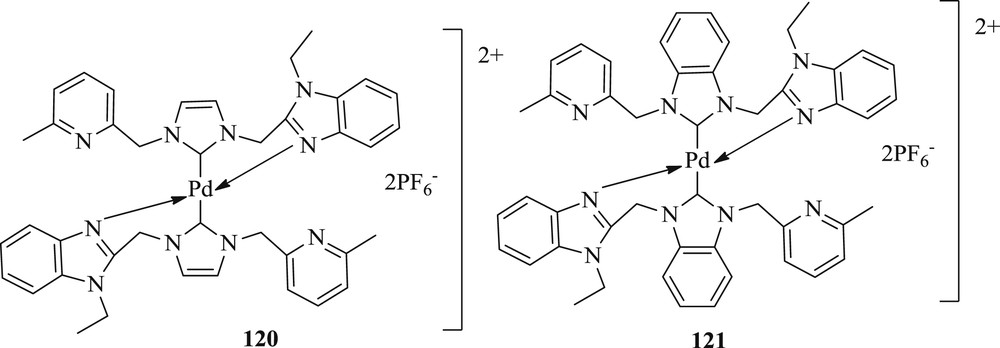
NHC complexes of Pd(II) complexes 120 and 121.
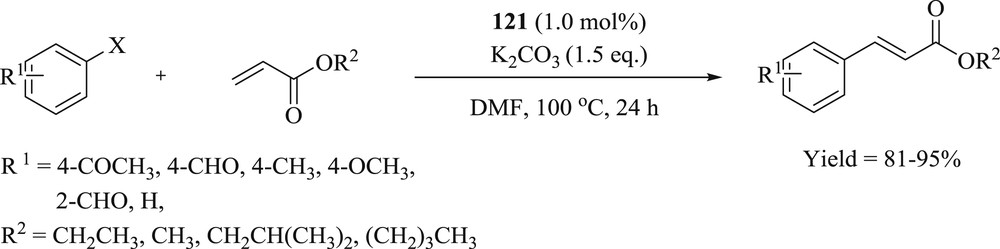
Reaction of aryl halides and various substituted acrylates catalyzed by 121.
A new approach has been developed by Teng et al. [69] for the synthesis of functionalized bis(NHC)–Pd(II) complex (124) using trans-[PdBr2(C3Br-bimy)2] (122) and trans-[PdBr2(C3Br-imy)2] (123) (Fig. 32) as common parents. The catalytic activity of trans-[PdBr2 (C3NEt3-bimy)2]Br2 (124) (Fig. 32) shows superior performance in the coupling reaction (Scheme 61) in comparison to its parent complexes 122 and 123.
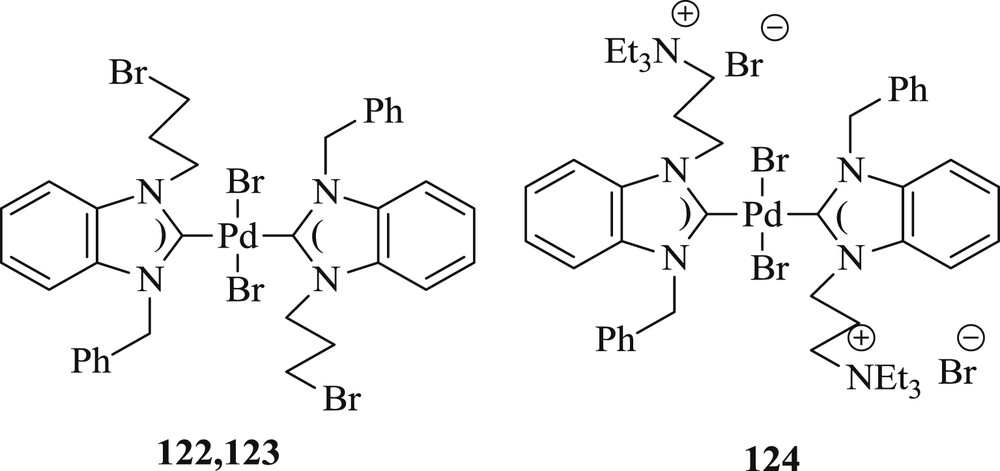
Bis(functionalized NHC) palladium(II) complexes 122–124.
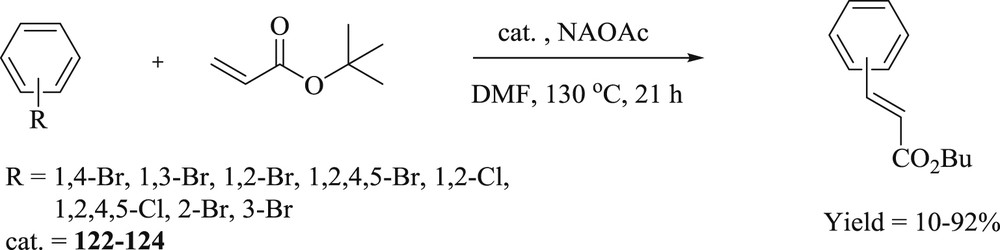
Mizoroki−Heck coupling reactions catalyzed by bis(functionalized NHC) palladium(II) complexes.
During the same period, the synthesis of [PdCl2{(CN)2IMes}(PPh3)] (126) and the palladacycles [PdCl(dmba){(CN)2IMes}] (127) was reported [70] using NHC precursor 4,5-dicyno-2-(pentafluorophenyl)imidazole (125). The new NHC precursor 1-benzyl-4,5-dicyno-(pentafluororophenyl)-3-picolylimidazoline (128) can be easily converted to precatalyst [PdCl2{(CN)2IBzPic}] (130) (Fig. 33). These compounds (126, 127, and 130) were applied in the Mizoroki–Heck reaction at a low dosage with sterically hindered and electronically deactivated substrates to give moderate yields (Scheme 62).
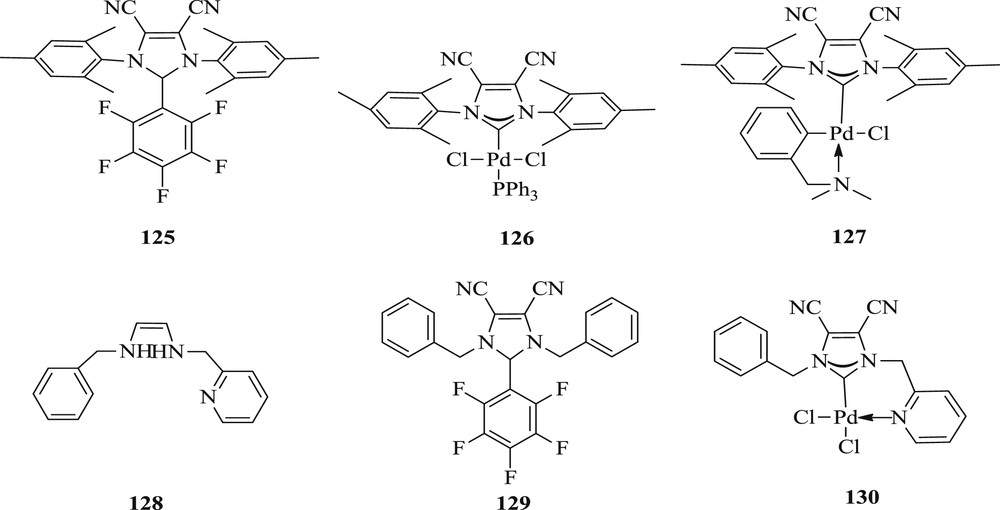
Precursors 125, 128, 129 and NHC–Pd complexes 126, 127 and 130.

Mizoroki–Heck reaction of n-butyl acrylate with various aryl halides catalyzed by 126, 127 and 130.
In 2014, the preparation of a new class of chiral hydroxyalkyl-NHC ligands was disclosed [71] (Fig. 34) that contained two stereogenic centers on the N-substituted and imidazolium ring backbones. The coordination of these chiral hydroxyalkyl-NHC ligands with Pd salts provided a proficient system for the carbon coupling reaction (Scheme 63). Also, these chiral hydroxyalkyl-NHC ligands were studied in enantioselective additions of diethyl zinc to benzaldehyde.
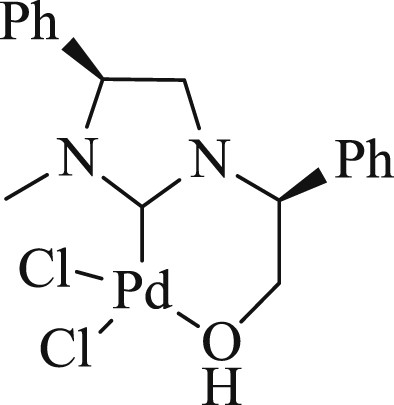
NHC ligand.
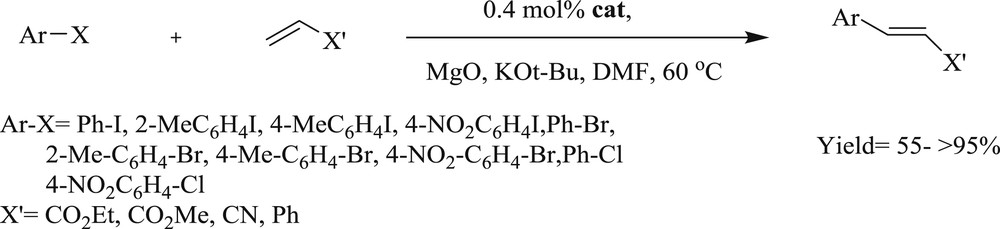
NHC–Pd catalyzed Mizoroki–Heck reaction.
Huynh et al. [72] have identified the synthesis of palladium(II) sulfonate–NHC complexes (Fig. 35) and their performance in aqueous Mizoroki–Heck reactions (Scheme 64). The conversions in the reactions with deactivated substrates like 4-bromotoluene and 4-bromoanisole furnished were not appreciable with these catalysts. The authors also could not explain the coupling of aryl chloride in aqueous media using the same catalyst.
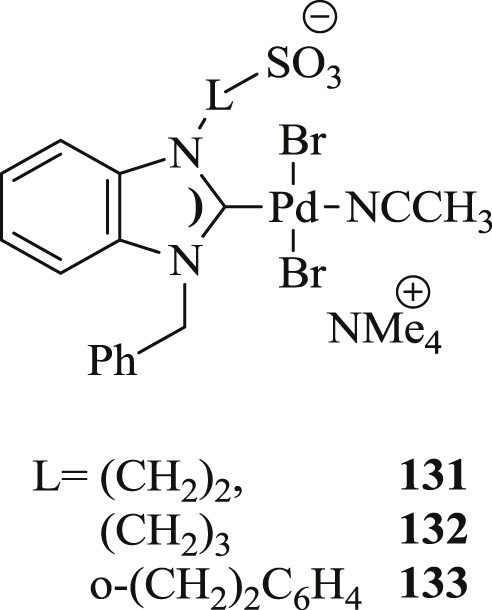
Palladium(II) sulfonate–NHC complexes.
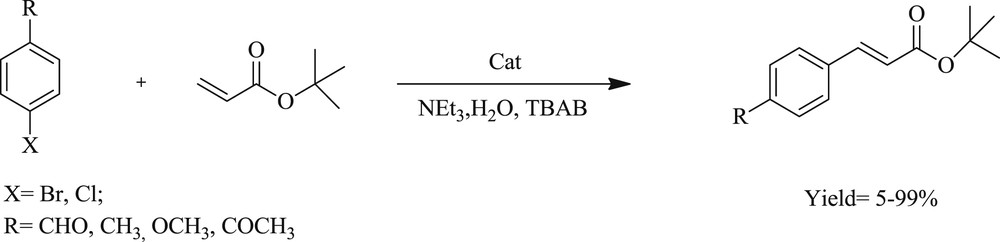
Mizoroki–Heck coupling reactions catalyzed by 131 and 133.
Interestingly, the Heck reaction (Schemes 65 and 66) mediated by chelated bis(NHC)–Pd complexes [73] (Fig. 36) along with the Density Functional Theory (DFT) study of the reaction at the B3LYP/6-31G* level, accompanied by M06/def2-TZVP single-point calculations have been examined. The authors have predicted that olefin insertion is the rate-determining step and the most likely mechanism is the cationic catalytic cycle involving the palladium oxidation state.
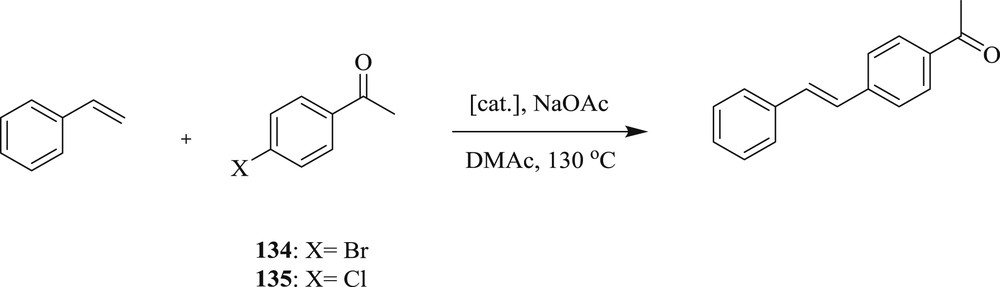
Heck reaction catalyzed by 134–135.

Heck reaction catalyzed by 134–136.
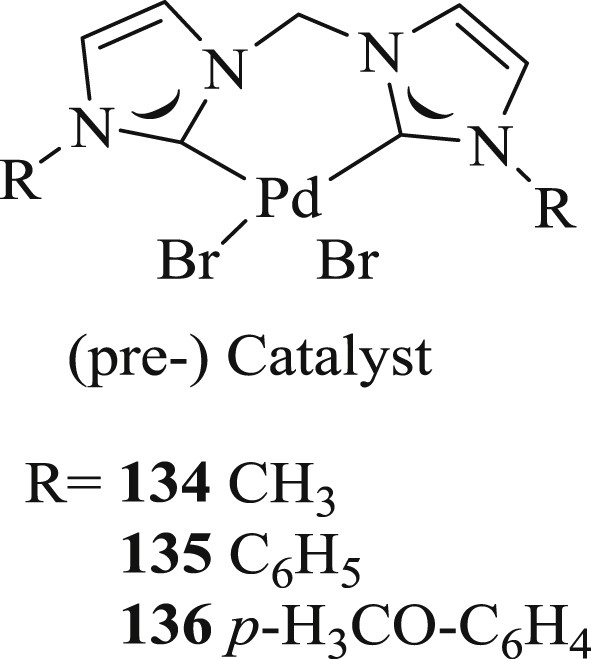
Palladium(II)−NHC complexes 134–136.
Very recently, chelating benzimidazolylidene palladium(II) complexes have been extensively prepared (Fig. 37) and evaluated in Heck and Suzuki reactions (Scheme 67) [74]. These compounds promote catalysis reactions efficiently in the presence of the coordinating carboxylate group. It was noticed that the catalytic performance accelerates when the carboxylate is dangling or coordinated in the CC coupling. Greater competences were achieved for substrates with a methoxy substituent in para rather than meta position. The presence of diverse types of donor sites (neutral and ionic) establishes an attractive concept for tailoring catalysts in cross-coupling reactions.
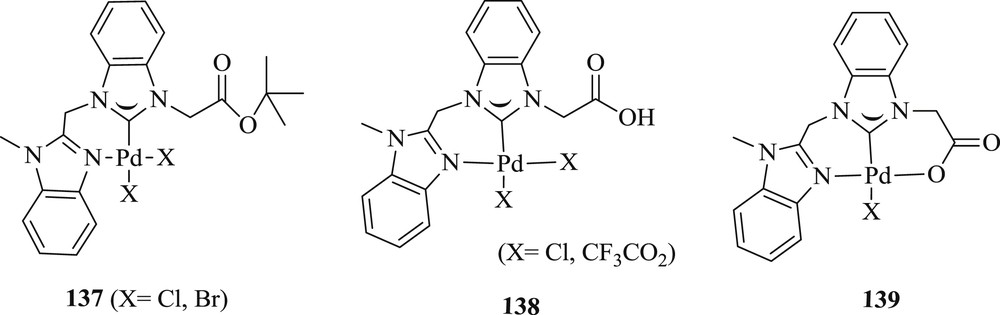
Pincer-type NHC complexes containing both anionic and neutral donors 137–139.
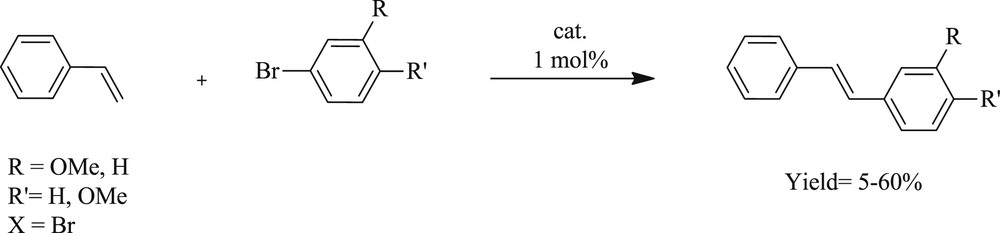
Pincer-type NHC complexes catalyzed Mizoroki–Heck cross-coupling.
The group of Navarro et al. [9] synthesized [TBA][(SIPr)PdCl3] (Scheme 68) by reacting (SIPr)PdCl2(TEA) with tetrabutylammonium chloride at room temperature in quantitative yields (Scheme 68). The complex has shown remarkable reactivity for the Heck reaction (Scheme 69) with high regio- and stereoselectivity.
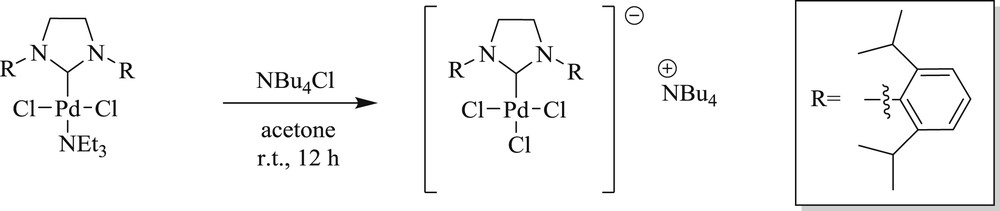
Synthesis of [(SIPr)PdCl3][TBA].

Heck couplings of aryl chlorides and bromides with styrene catalyzed by [(SIPr)PdCl3][TBA].
Lee et al. [75] have devoted a lot of research in the synthesis of a variety of Pd(0) complexes with NHC ligands with hanging NMe(CO) functionalities (LMe). The reactivity of bulky N-mesityl (Fig. 38) groups toward the oxidative addition of pentafluoroiodobenzene was studied and it confirmed the formation of decafluorobiphenyl, the Pd(II) diiodide complex (141) and an intriguing Pd(II) complex with four carbon-donor ligands coordinated to the metal center.
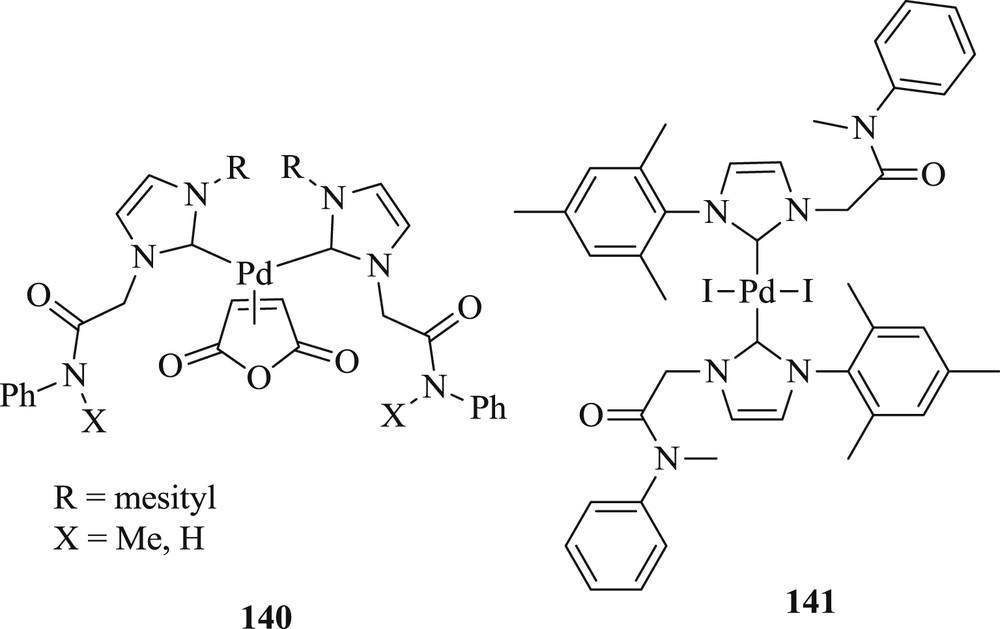
NHC–Pd precatalysts.
The Pd(0) complex has been shown to be a promising mode for the coupling reaction (Scheme 70) between 4-chloroacetophenone and styrene with a higher yield than LMe, which can be correlated with the probable deprotonation of the NH proton in the anterior ligand and the formation of the highly electron-rich anionic [Pd(0)L] dynamic species. It was also illustrated that the activity order was not the same for (140) with bulky N-substitution on the ligand system, because of decomposition of Pd(0) intermediate.
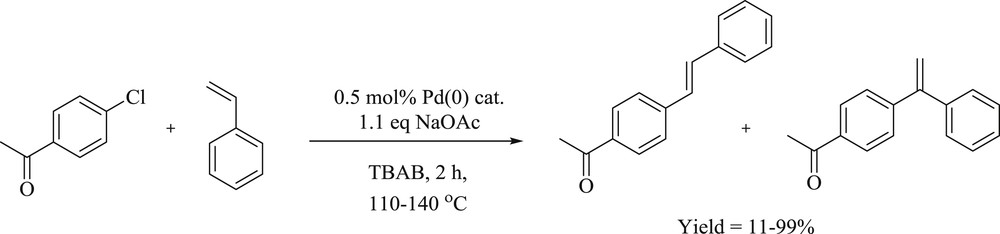
Mizoroki–Heck coupling reaction using the NHC–Pd catalyst.
In the same year, another research group [76] described new ligand precursors (142) with different dangling alkyl chain length in 1-alkyl-2-methyl-1H-imidazoles by reacting with chloroacetone (Fig. 39). These precursors were converted to simple zwitterion complexes (143) and applied as metal precursors to generate Pd NPs. The authors have described the catalytic activities of these Ionic Liquid (IL)-stabilized NPs for the reaction between 4-iodoacetophenone and n-butyl acrylate (Scheme 71). They highlighted the fact that a high yield of the coupled product with very good recyclability using 143 as a precatalyst was produced. Furthermore, it was pointed out that no significant effect on activity and recyclability was noticed by incorporating longer alkyl chain on the ligand framework.
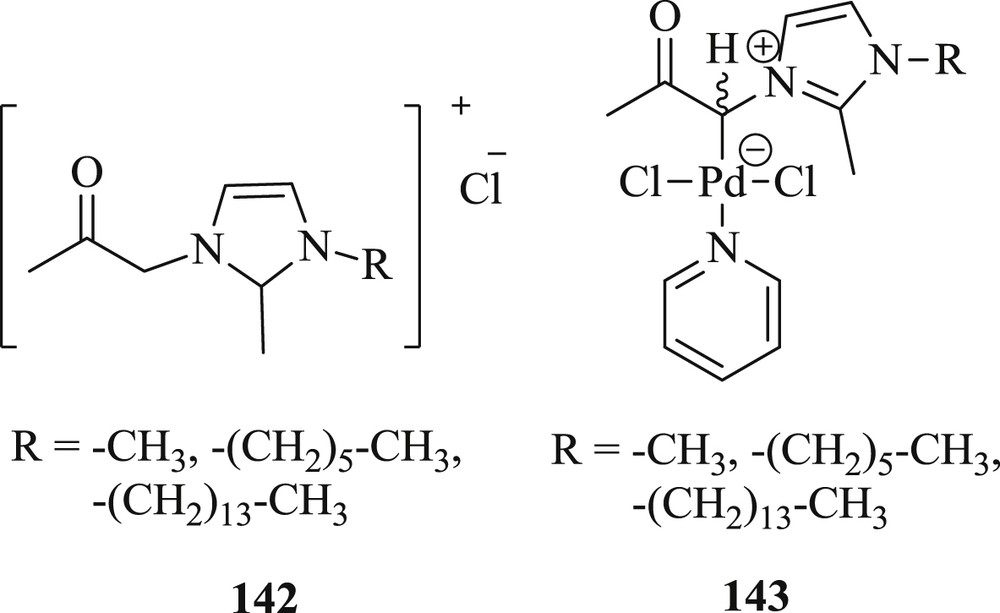
Pd(II) NHC complexes 142 and 143.
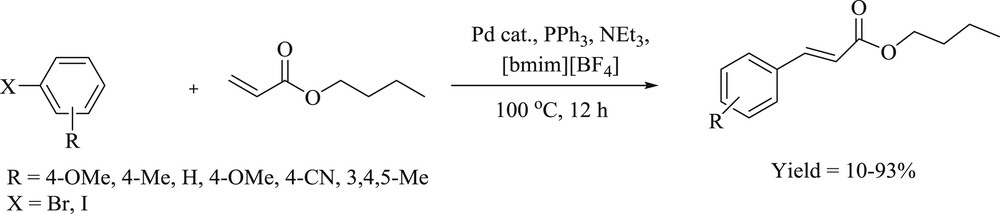
Mizoroki–Heck reaction of aryl halide with alkene using 143 as precatalyst.
A new strategy has been used by Richeter et al. [77] to synthesize palladium(II) complexes (Fig. 40) by using porphyrins fused to NHCs (144), with inner metal as M = Ni, Cu, Zn, and H2. These complexes (145) demonstrated high-temperature Mizoroki–Heck coupling reactions between iodobenzene and styrene (Scheme 72). It was observed that catalytic activity was considerably high, depending on a central metal incorporated in the porphyrin core, that is, higher yield conversion with metaloporphyrins (M = Ni, Cu, and Zn) compared with free base porphyrin. The authors have also proved that Pd leaching occurred in the course of the reaction, and it was confirmed by poisoning experiments with Hg and Transmission Electron Microscopy (TEM) analyses that Pd NPs play a key role in the catalytic activity.
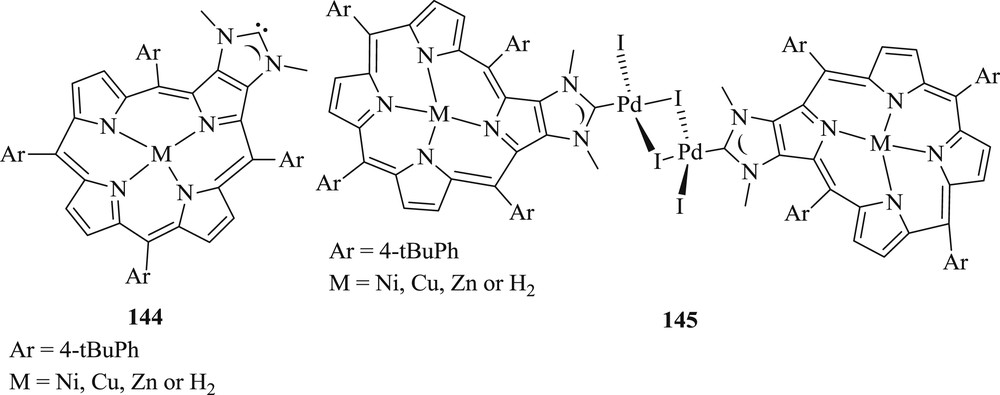
Pd–NHC complexes.
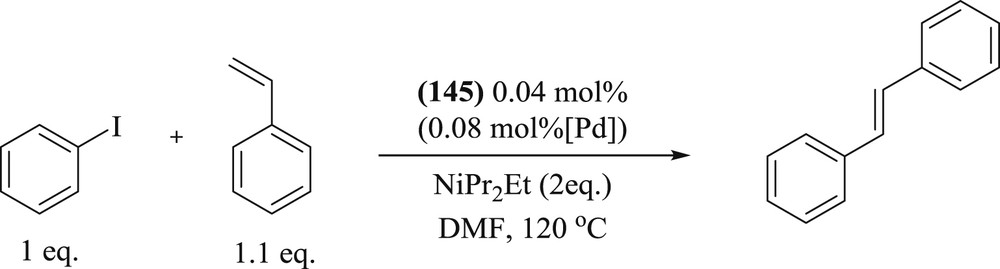
Mizoroki–Heck coupling reactions catalyzed by 145.
Bis-benzimidazolium salt (S)-2,20-bis[200-(N-picolyl-benzimidazoliumyl)ethoxy]-1,10-binaphthyl hexafluorophosphate [(S)-LH2](PF6)2 and its NHC–palladium(II) complex [(S)-LPd](PF6)2 (Fig. 41) have been reported [78]. The synthesized catalyst (Fig. 41) gave an optimal coupling product for Heck, Suzuki, and Sonogashira reactions in the presence of 10 mol % of PEG-400 as a cocatalyst at 100 °C in air. Thus, it was proven to be a valuable precatalyst to obtain diaryl derivatives, styrene derivatives, or phenylacetylene derivatives.
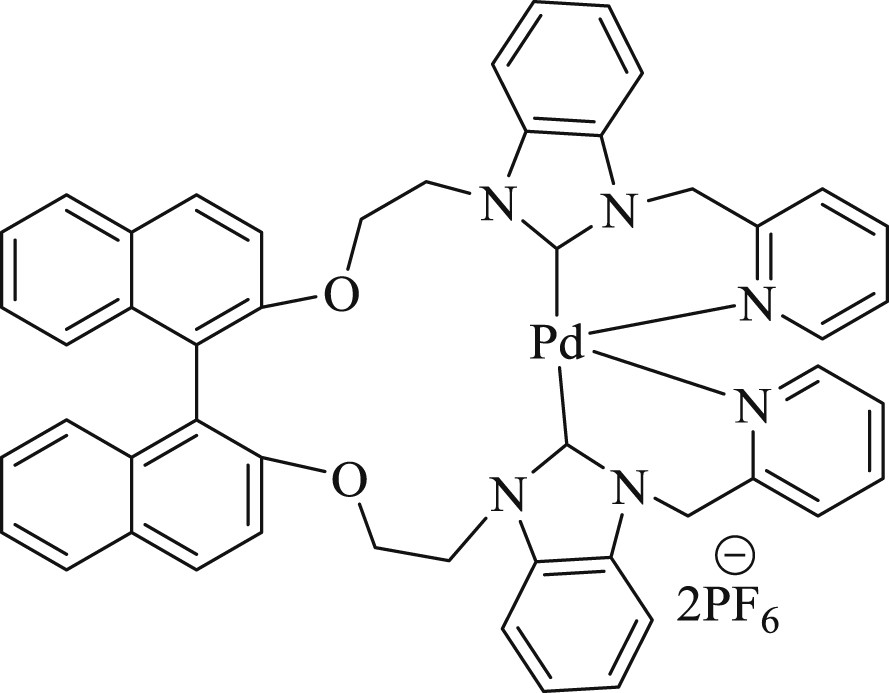
Pd–NHC complex.
Subsequently, two NCN pincer ring-expanded six-membered NHC–palladium complexes containing 1,3-dipicolyl-3,4,5,6-tetrahydropyrimidin-2-ylidenes have been synthesized by the direct metalation of the corresponding tetrahydropyridin-1-ium salts [79]. Catalytic activity study showed that the complexes catalyzed the Heck reaction of aryl bromides with acrylate/styrene efficiently using Et3N as base and DMA as a solvent. The results revealed that complexes (146 and 147) (Fig. 42) offered nearly similar catalytic activity, giving the required products in moderate to high yields (Scheme 73). The substituents on the phenyl ring of the aryl bromides did not show any noticeable electronic effect. But because of steric effect, low yield was observed in some cases of ortho-substituted phenyl bromide and 1-naphthyl bromide. The Heck reaction of phenyl bromide or para-substituted phenyl bromide with olefins was achieved in lesser time or with lower catalyst loading, using these complexes.
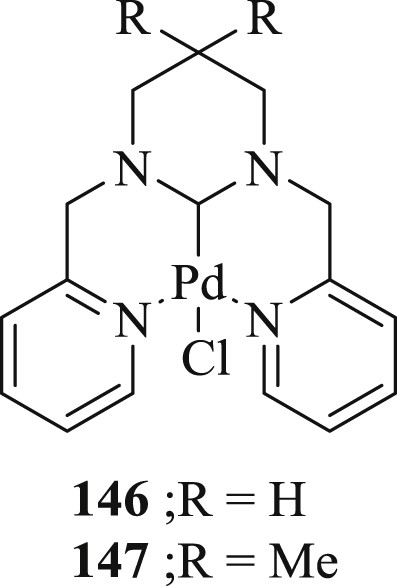
NCN pincer palladium complex.
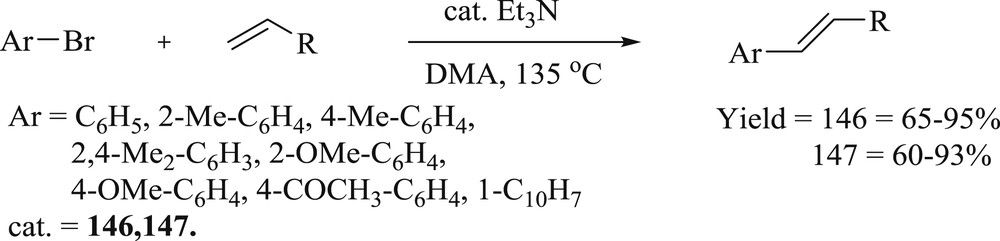
Heck reaction of aryl bromides with olefins catalyzed by 146 and 147.
In the same year, the first air stable SCS pincer complex that uses N-heterocyclic thione donor groups was synthesized and its photophysical properties and catalytic activity in Heck, Suzuki, and Sonogashira cross-coupling reactions were evaluated [80]. The authors have not evaluated the nature of catalytically active species during the reaction as the activity of compound (Fig. 43) was found to be modest.
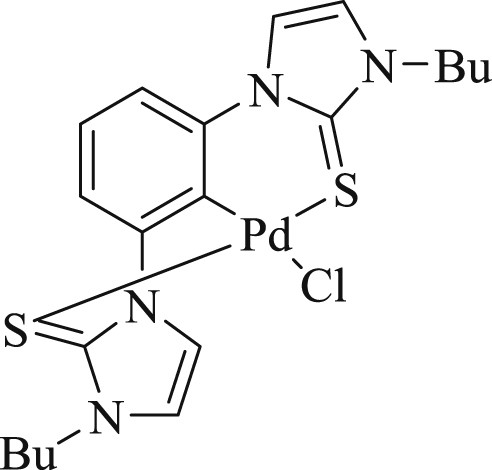
Bis(N-heterocyclic thione) (SCS–NHT) Pd pincer complex.
Taira et al. [81] prepared a surface-active NHC ligand with octaethylene glycol monomethyl ether (Fig. 44) and n-dodecyl chain and successfully used for an aqueous Mizoroki–Heck reaction under heterogeneous conditions (Scheme 74). The role of the catalyst thus obtained was demonstrated in the aqueous CC coupling reaction, affording the product, which could be separated by simple filtration, with an excellent yield (99%). The method is environmentally benign, in that it allows the reactions to proceed attractively as a neat reaction without the addition of additional surfactants and solvents. The limitation of this protocol is that it remains ineffective for aryl chloride.
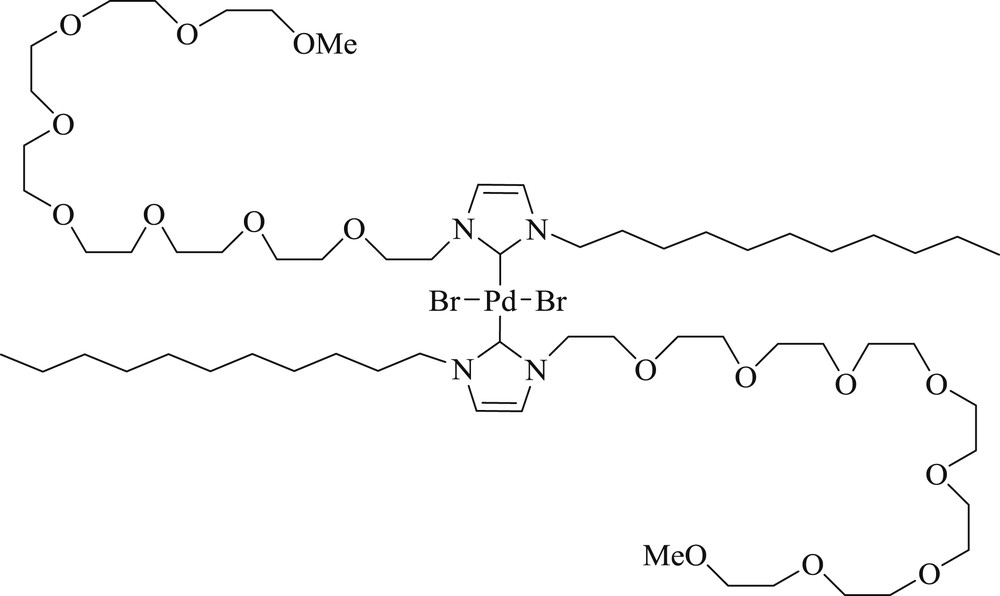
Surface-active N-heterocyclic carbene Pd catalyst.
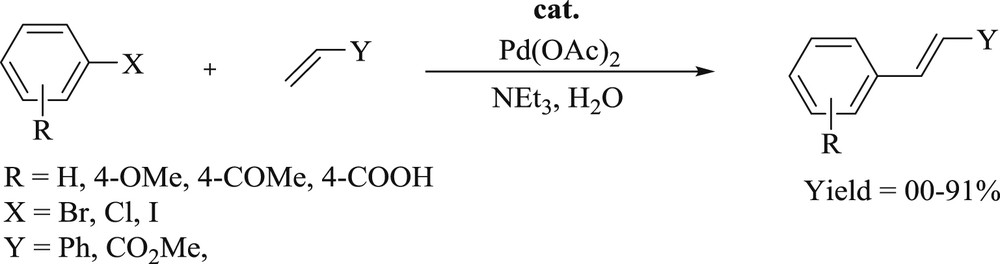
Mizoroki–Heck reactions between iodobenzene and styrene catalyzed by the NHC–Pd catalyst.
Two ligands pentamethylene-functionalized bis-imidazolium dication ligands L1 and L2 (L1 = 1,5-bis(4-(imidazolium-1-yl-phenol)pentane dibromide; (L2 = 1,5-bis(1-vinylimidazolium-1-yl)pentane dibromide) bearing IP (4-(imidazol-1-yl)phenol) and their silver(I) and palladium(II) complexes have been verified [82]. The Pd(II)–NHC complexes (148 and 149) (Fig. 45) showed good activity in Heck and Suzuki–Miyaura CC coupling reactions. It was ascertained that catalysts tolerated the olefination reactions of activated, nonactivated, and deactivated aryl halides with vinyl compounds such as n-butyl acrylate or styrene progressing at 120 °C using only 0.1 mol % of (148 or 149) with good to admirable yields (40–98%) (Scheme 75). The low yields of the coupling product were achieved with aryl chloride or nonactivated aryl chlorides owing to the stronger Csp2–Cl bonds of aryl chlorides than those of the heavier congeners.
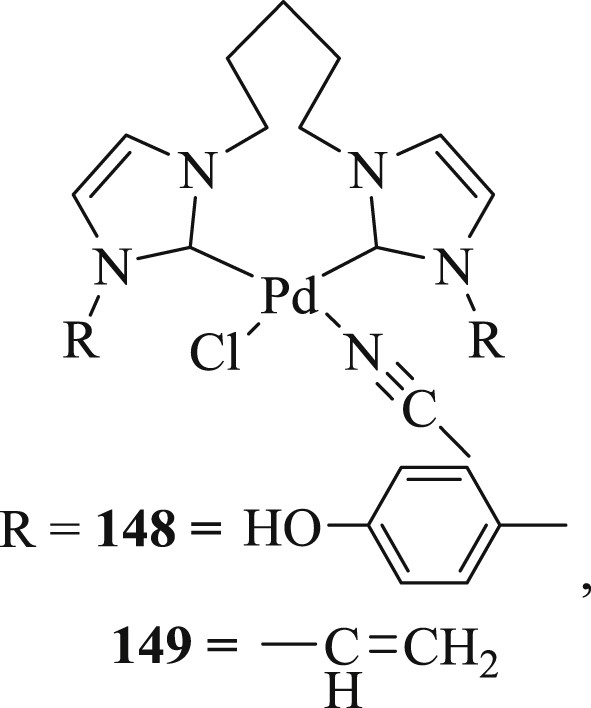
Pd(II) NHC complexes 148 and 149.
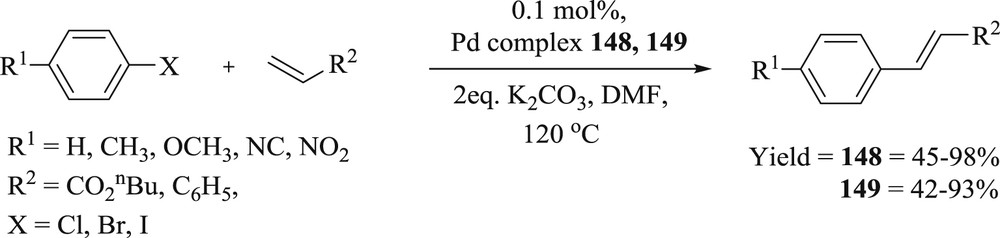
Heck cross-coupling reactions of aryl halides with vinyl compounds using complexes 148 and 149.
Wang et al. [83] described the synthesis of pillar [5] arene-based 1-benzylimidazole hexafluorophosphate (150) and pillar [5] arene-based 1-(9-anthracenylmethyl)imidazole hexafluorophosphate (151) (Fig. 46). These ligands, along with PdCl2(CH3CN)2, were used as catalysts in the coupling reactions of different derivatives of an aryl halide with styrene. It was observed that salt with ligand (150) presented better catalytic activity because of steric bulk of the ligand than ligand (151). The catalyst tolerated all electron-donating groups of iodobenzene and bromobenzene derivatives to yield corresponding coupling products in admirable yields (Scheme 76), and the coupling of chlorobenzene derivatives also progressed easily, giving the products in moderate yields.
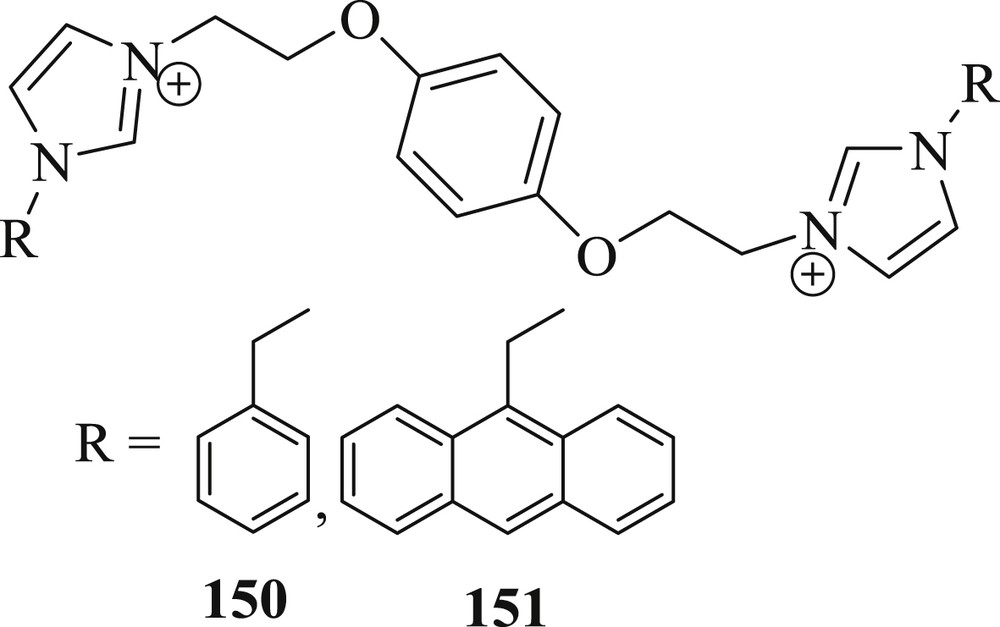
NHC ligand.
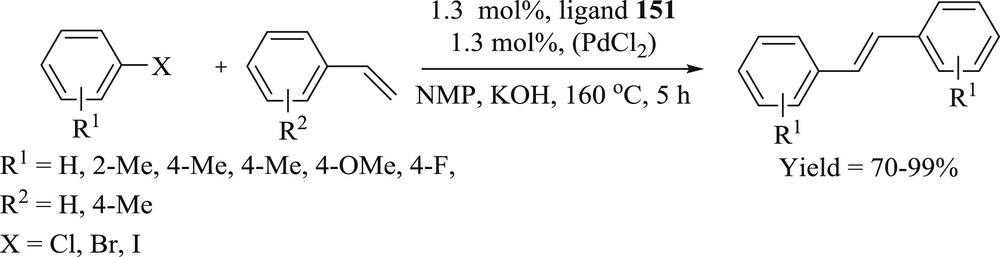
Mizoroki–Heck reaction: coupling of different aryl halides and styrene.
Three palladacycle complexes (152–154) containing N,N-dimethylbenzyl amine (dmba) and the benzimidazol-2-ylidene ligands were prepared [84]: 1,3-di(cyclohexyl)benzimidazol-2-ylidene (BzImCy), 1,3-di(tert-butyl)benzimidazol-2-ylidene (BzImtBu), and 1,3-di(1-adamantyl)benzimidazol-2-ylidene (BzImAd). The catalytic activity of air stable complexes (152–154) (Fig. 47) was studied for the Heck–Mizoroki (Scheme 77) and Suzuki-Miyaura CC coupling reactions of simple aryl bromides. The reactions performed under inert atmosphere showed no appreciable yields in the activities of the precatalysts for the preparation of methyl 4-methoxycinnamate.
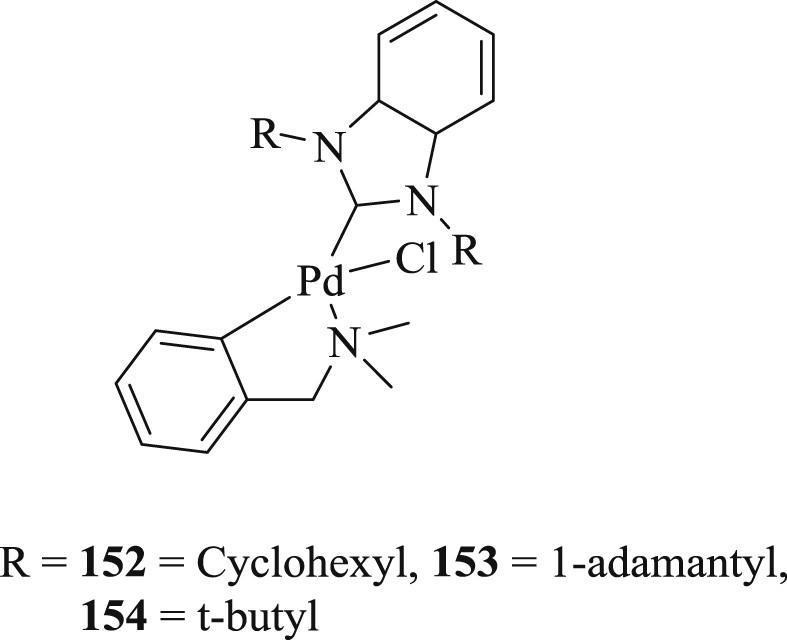
(BzIm)Pd(dmba)Cl complexes 152–154.
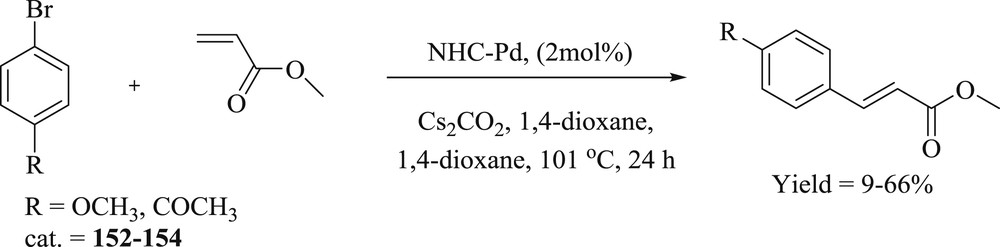
NHC–Pd complexes for the Heck–Mizoroki coupling of 4-methoxybromobenzene and 4-bromoacetophenone with methyl acrylate.
Kumar et al. [85] presented the one-pot tandem Heck alkynylation/cyclization reaction of 2-iodophenol with terminal alkynes, yielding the 2-substituted benzofuran compounds, using a series of binuclear palladium complexes, viz., (155–158) and (159–162) (Fig. 48), of ethyl- and propyl-bridged NHC ligands. As a pioneering study, the present investigation on the NHC-based catalysts provides challenging prospects for additional progress for one-pot tandem Heck alkynylation/cyclization reactions (Scheme 78) in reduced numbers of reaction steps and for similarly associated tandem reactions.
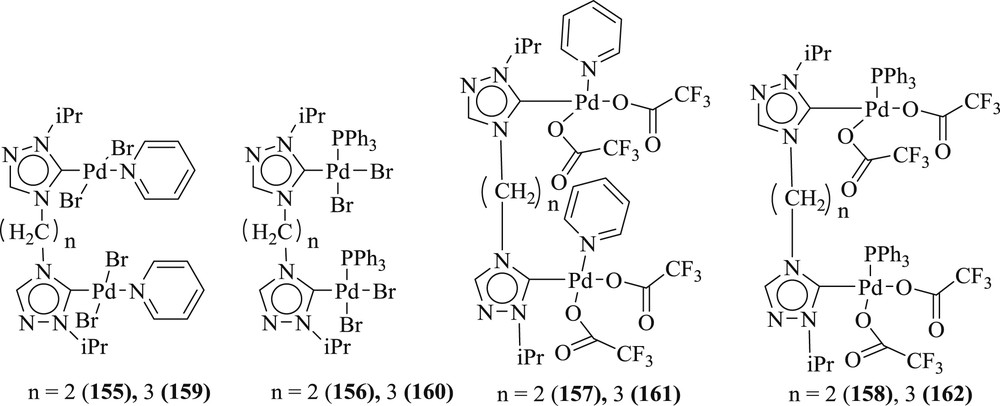
Palladium complexes of 1,2,4-triazole-derived NHC ligands.
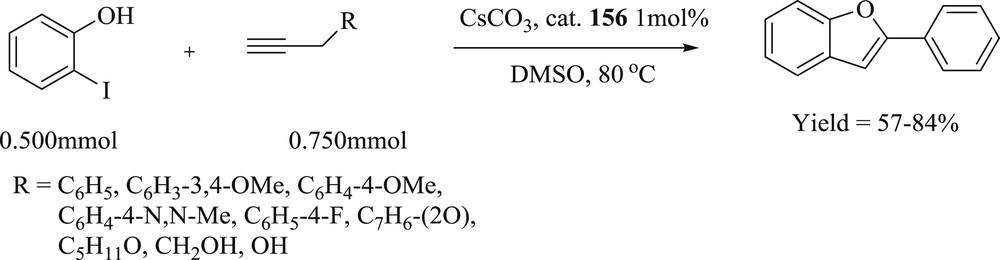
Tandem Heck alkynylation/cyclization reaction of Iodophenol and various and terminal alkyne substrates.
Haque et al. [86] have synthesized a series of pyridine linker containing bis(benz)imidazolium salts serving as precursors to CNC pincer-type NHC (Fig. 49) ligands by the alkylation reaction of the azole core. Binuclear pincer-type palladium(II)–NHC complexes were obtained by trans-metalation of benzimidazolium silver(I) complexes (Fig. 49) in acetonitrile reaction medium. The catalytic abilities of the palladium complexes were verified by their activity toward Heck–Mizoroki (Scheme 79) and even Suzuki–Miyaura in the aqueous phase. In the former coupling reaction, biphenyls displayed low yields of n-butyl cinnamate, whereas Suzuki–Miyaura coupling reaction showed yields up to 71–99%.
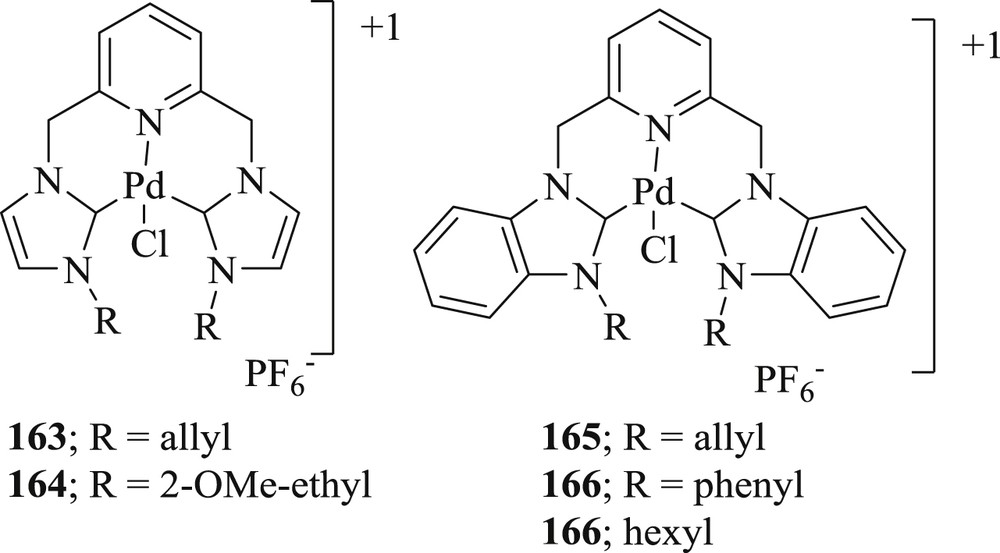
Palladium(II) complexes derived from CNC pincer-type N-heterocyclic carbenes 163–166.

Heck–Mizoroki cross-coupling reaction of bromobenzene with n-butyl acrylate.
Lee et al. [87] have prepared two series of palladium(0) complexes (Fig. 50) with phosphine-functionalized NHC ligands. These complexes contain six- and seven-membered chelate rings in the two series. The activity of these metal complexes was verified toward CC Heck coupling and for direct CH functionalization. This catalyst showed enhanced yield in the Mizoroki–Heck reaction up to 96% (Scheme 80).
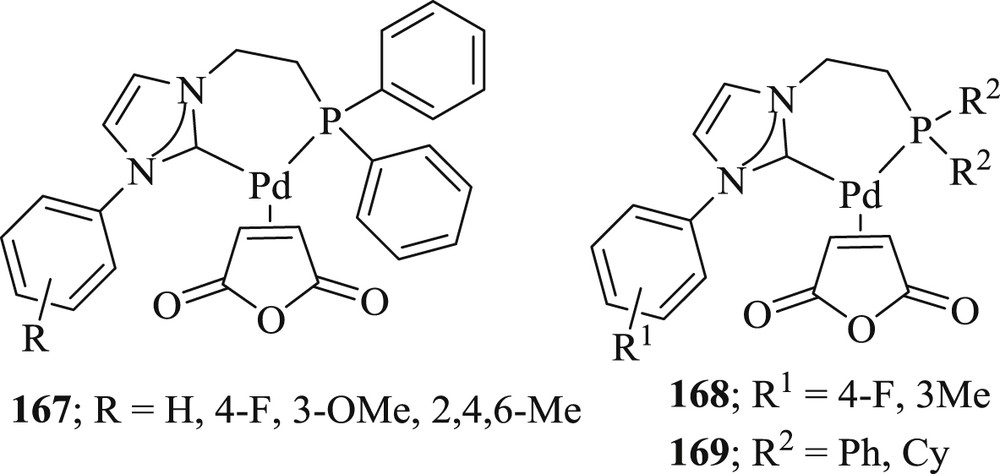
Pd–NHC complexes 167–169.

Mizoroki–Heck reaction of alkenes with aryl halides catalyzed by 167–169.
3 Conclusions and perceptions
The current appraisal deals with the progress in NHC–palladium complexes and their uses in various CC cross-coupling and other reactions. For the last two decades, NHCs have gained importance as ligands in the Pd-mediated reaction because of their advantages over tertiary phosphine ligands. The typical features of NHCs are electron-rich, neutral donor ligands, and their electron donating ability span over a very narrow range as compared with phosphine ligands. It can form very strong bonds with most metals and even generates stable complex below −20 °C. Furthermore, a number of additional high-valent metal complexes have been stabilized with NHCs. Another exclusive feature of NHC is its tunable steric and electronic characteristics that enhance bonding stability including thermal strength. It has been the effort by many researchers to design an extremely capable precatalyst by incorporating bulky carbon substituents on imidazole, benzimidazole, and other suitable moiety for their high catalytic activity. These functional units would also give an extra dimension to control the steric and electronic properties of the heterocyclic ring, apart from the well-established ring-modification method. Despite the remarkable achievement of NHC–Pd compounds, a number of tasks still remain challenging. The combination of low cost and easily available aryl chlorides in CC coupling continue to face difficulty or furnish very low yields using the available protocols. Another crucial limitation that needs to be ascertained is the reusability of Pd(II)–NHC complexes. This parameter needs more study in view of the shortcomings and potentialities of any homogeneous protocol to improve its design that avoids leaching of palladium during the course of reactions. We have all the motivation to improve existing applications for these NHC–Pd catalyzed carbon–carbons and other bond forming reactions to meet the academic and industrial interests. We predict that in the coming era innovative, more effective and green practices for CC coupling and other reactions will become accessible, assisting the production of an enormous range of compounds from the most miscellaneous starting materials. A concluding observation is that none of these schemes is superior in terms of metal loading as the common palladium content used is 1 mol %. Definitely, for cost-effective and ecological industrial applications and to upgrade these NHC–Pd complexes, improvement has to be made to minimize metal loading and stability of homogeneous systems.
Acknowledgments
The authors express their deep gratitude to RGEMS, VIT University, Vellore for providing a necessary facility for their research. They are also thankful to Dr. K. Thenmozhi, School of Advanced Sciences and Prof. Ajanta Sircar, School of Social Science and Languages, VIT University, Vellore for their valuable suggestions and all the Scholars of our Research Laboratory, “Smart Materials Laboratory for Biosensing & Catalysis” VIT University Vellore, who have encouraged us during this period.