1 Introduction
In an inspired essay, Roald Hoffmann reflected on how chemists perceive “molecular beauty” [1]. According to Hoffmann, beauty may arise from novelty, epitomized at the time by metal clusters containing six-coordinated carbon atoms. Beauty may also be associated with molecular shape, and some examples given by him are “simple, symmetrical and devilishly hard to make”, but other forms of molecular beauty have to do with the perception of the chemist of what is unique about its composition, structure or bonding. Finally, what we may consider beautiful is the way a molecule behaves in front of some external stimulus, as exemplified by haemoglobin, a porphyrin-bearing protein that experiences important changes in response to the binding of oxygen.
In this article, I wish to present more recent examples of beautiful molecules. In some of them I find beauty in the way they surprise us by adopting a structure in space that is hard to anticipate from a structural scheme. Conversely, in some cases it is the two-dimensional (2D) scheme of a molecule that excites our imagination. The choice of structures to be discussed is completely subjective, based on my perception of what is interesting, beautiful or just funny in the molecular shapes displayed by them.
2 Deceiving structural drawings
An example of how a structural drawing may give us poor information of a remarkable 3D molecule is provided by hexadecaphenyl-dodecabenzene [2]. Although it is drawn on paper as a square molecule (Fig. 1a), its structure in space is that of a tetrahedron such that chains of four phenyl groups occupy four out of the six edges of the tetrahedron (Fig. 1b). Continuous shape measures [3] of the centres of the four phenyl groups at the corners indicate a nearly perfect tetrahedron, with just a slight (9%) distortion towards a square. The shapes of the 2D scheme and the real molecular structure can hardly be more different!
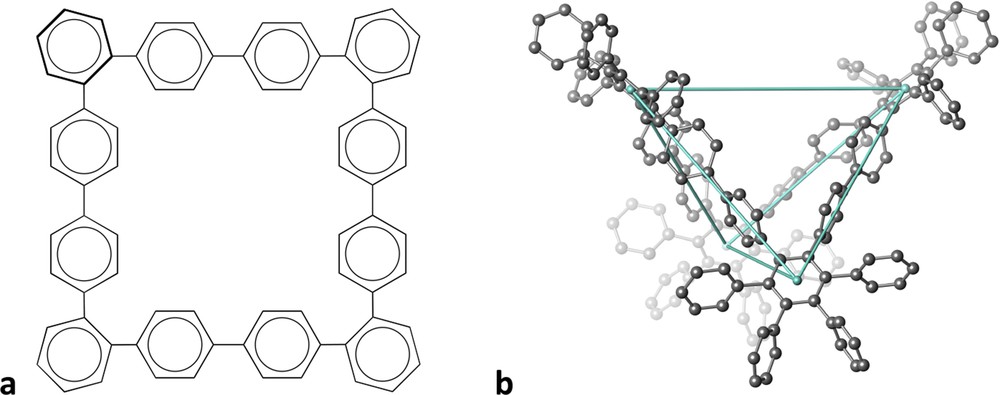
Schematic drawing of a dodecabenzene skeleton (a) and its 3D tetrahedral structure (b).
To the variety of molecules that have the shape of daily live objects [4], two cases have been chosen that also stress the inability of 2D schemes to suggest their 3D structures. Both are fully aromatic molecules composed of fused phenyl rings around a central five- or seven-membered ring (Fig. 2a and b, respectively) that look quite similar when represented as a 2D scheme. However, their 3D structures are non-planar and very different: while the former has the shape (Fig. 2c) of a bowl [5] – and is often referred to as a “buckybowl” –, the latter is non-planar as well, but bent in such a way as to form a sawhorse [6] (Fig. 2d).
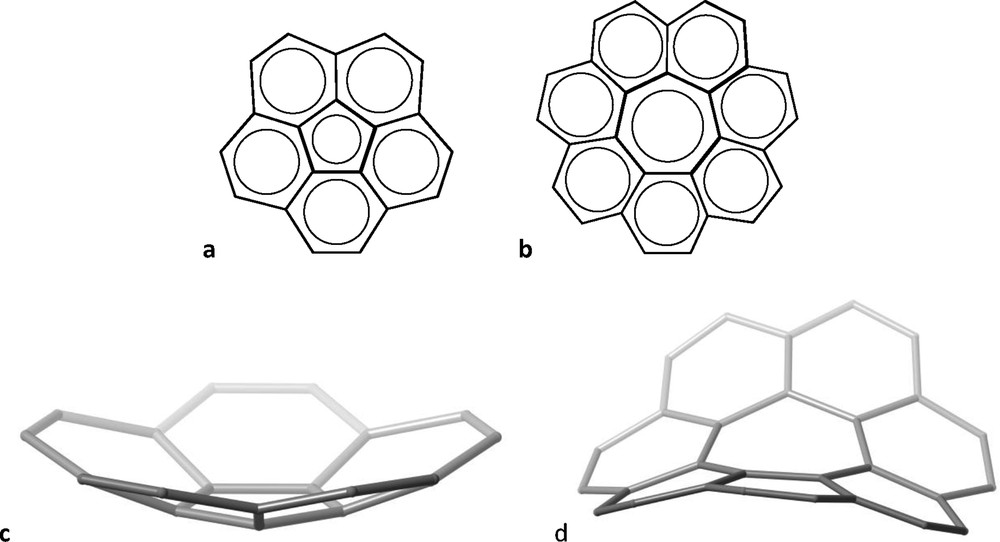
Two-dimensional representation of corannulene (a) and [7] circulene (b), and their 3D structures (c and d, respectively).
3 Impossible structural drawings
When we try to draw a sequence of fused phenyl rings everything seems to work right for up to five rings (Fig. 3a). However, our drawing suggests a planar molecule, far from what we find in its crystal structure [7], that shows a helical geometry in keeping with its name “pentahelicene” (Fig. 3b). If we wish to add one more phenyl ring, with all of the rings being regular hexagons, we end up (Fig. 3c) with a representation of coronene, C24H12, instead of hexahelicene, C26H16. To make a 2D representation of the latter, we must make use of irregular hexagons (e.g., Fig. 3d), but we still misrepresent the helical shape of the molecule. The situation worsens as we go to higher helicenes, requiring highly distorted hexagons. An alternative is to use hexagons of increasing size, to avoid the rings to overlap and additionally providing some sense of perspective that transmits better the idea of helicity, as shown in Fig. 3e for heptahelicene. But, regardless of how we represent them in two dimensions, the 3D structures of the helicenes look highly attractive and reminiscent of the spiral staircase of the entrance Hall of the Louvre museum, as illustrated by undecahelicene [8] and hexadecahelicene [9] (Fig. 4).
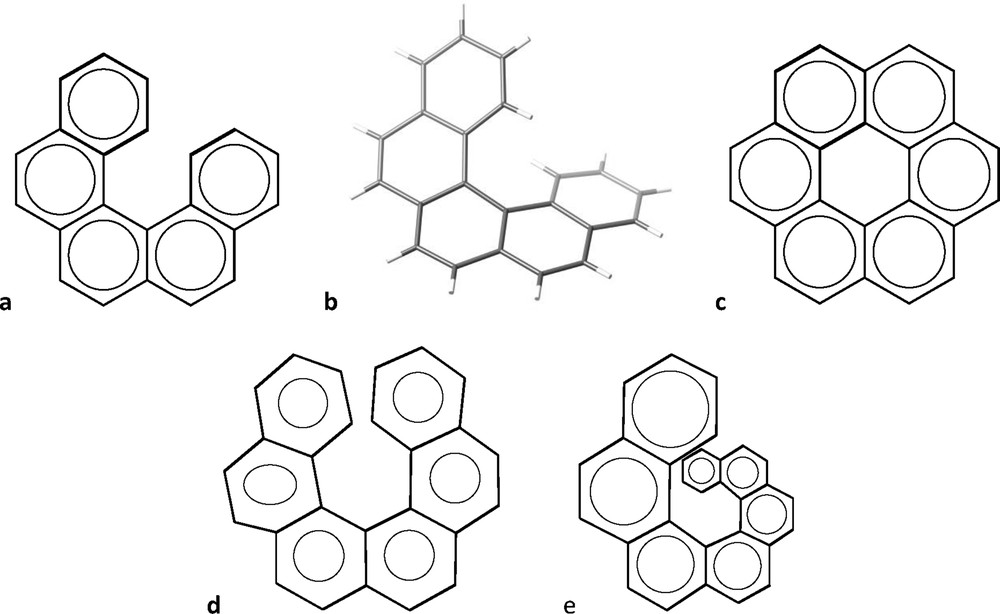
Two-dimensional representation of pentahelicene (a) and its 3D molecular structure (b), an arrangement of six regular hexagons that corresponds to the formula of coronene (c), a set of six distorted hexagons representing the molecular formula of hexahelicene (d), and fused six-membered rings of different sizes that give some sense of the helical nature of heptahelicene (e).
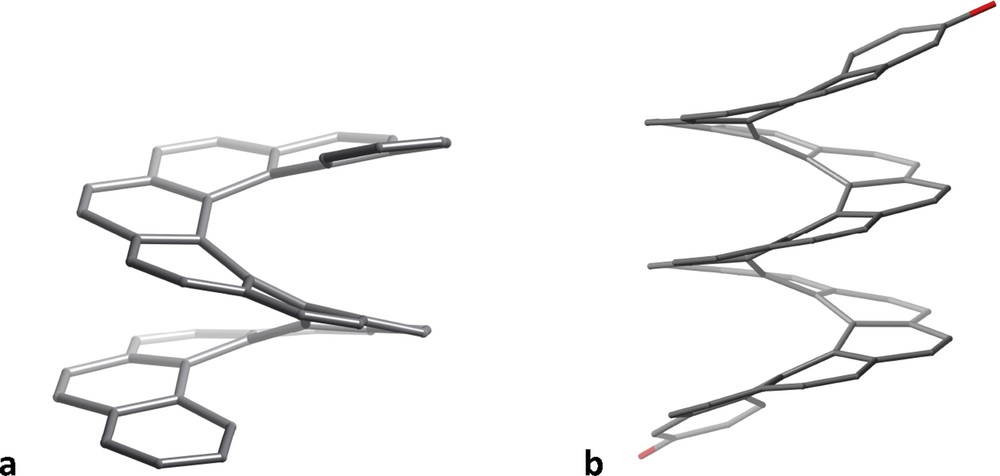
Helical structures of the skeletons of undecahelicene (a) and 3,34-bis(triisopropyl-silyloxy)hexadecahelicene (b), the two terminal triisopropoylsilyl groups are omitted for clarity).
4 Unusual coordination geometries
In the past years, we have extensively studied polyhedral molecular structures and their symmetry properties [10–14]. A number of supposedly uncoordinated or monocoordinated transition metal compounds were also recently analysed and showed the existence of hidden beautiful molecular shapes [15]. But the ability of chemists to design and synthesize molecules with new shapes gives me the opportunity to call the attention to some interesting new polyhedral shapes.
The coordination of a cyclodimethylsiloxane with an O6 donor set to an Ag(I) ion [16] produced a complex with three short Ag-O distances of about 2.42 Å, which would correspond to a canonical trigonal planar coordination of a d10 ion, but the three remaining oxygen atoms are held at not much longer distances of about 2.67 Å, thus making this molecule a reasonable example of an unusual hexagonal planar coordination of a metal ion (Fig. 5a). In a related compound the counter ion establishes a coordination bond to Ag at an axial position, thereby forming an also unusual hexagonal pyramidal coordination. A similar situation can be found [17] with the 18-crown-6 ligand that, together with an oxygen atom of the triflate counter ion, forms an apparent heptacoordination around a Ag(I) ion, in a rather distorted hexagonal pyramid (Fig. 5b).
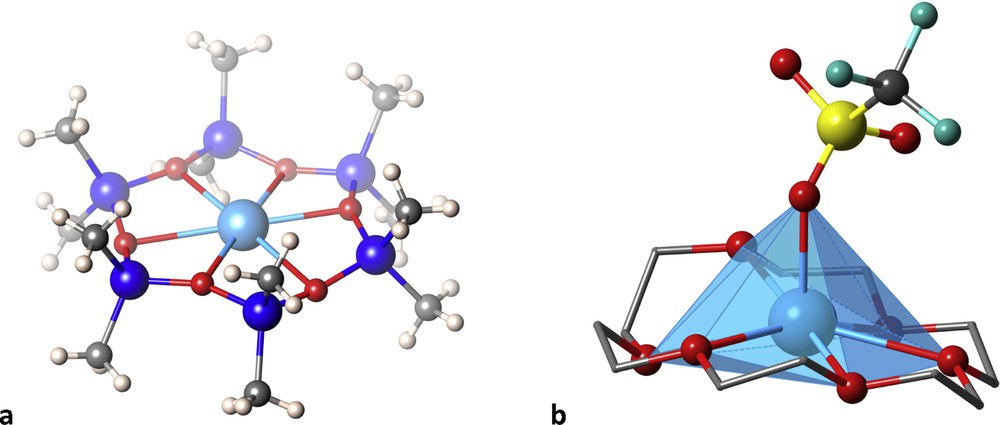
Two cyclic ligands with O6 donor sets that favour apparent hexagonal planar (a) and hexagonal pyramidal (b) coordinations to Ag(I) ions.
Very high coordination numbers are rare, but once they are achieved, some unexpected coordination polyhedra can be found, such as the octagonal antiprismatic “drum” of the CoB16− cluster [18] (Fig. 6a), or the hexacapped hexagonal prism of the LaNi18 group (Fig. 6b) in the solid state structure of LaNi5 [19], with the so-called CaCu5 structure found in hundreds of binary intermetallic compounds.
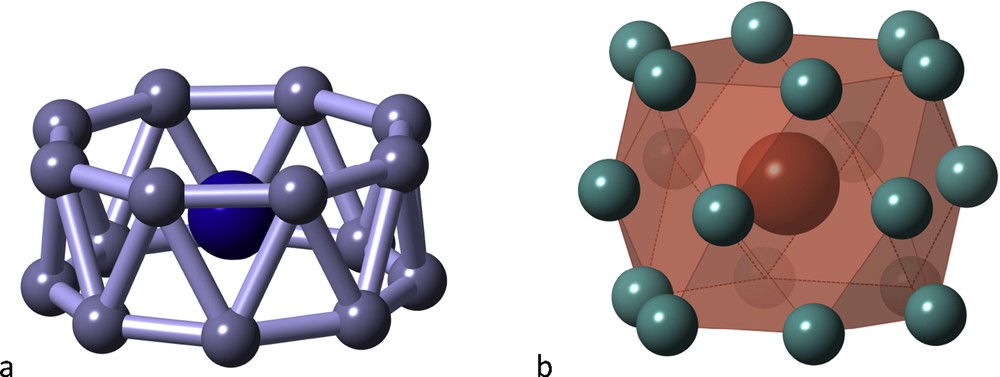
Two drums with very high coordination numbers: the CoB16− anion (a) and the LaNi18 group in LaNi5 (b).
An attractive double decker sandwich structure (Fig. 7) combines three slices of “bread”, the outer ones formed by two different porphyrins and the inner one by a phthalocyanine ring [20]. The metal ions sandwiched by these rings are Ce(III) and Gd(III). Although both metal ions are octacoordinated, Ce is in a cubic environment and Gd in a square antiprismatic one and both polyhedra share a square face.

A Gd and Ce double decker sandwich complex with a square antiprismatic and a cubic coordination spheres, respectively, that share a square face.
5 Stars and planets of several sorts
Some molecules may remind us of planets and stars. For instance, a ring made up of 11 phenyl rings surrounding a C70 fullerene [21], resembles the planet Saturn (Fig. 8a) with its planetary rings. Also simple molecules, such as the XeF5− anion [22] or the well-known tetramethylsilane, SiMe4 [23], appropriately coloured, look like shining stars (Fig. 8b and c, respectively).

A ring made up of 11 phenyl rings encircling a C70 fullerene that reminds the planet Saturn (a), a XeF5− star (b) and the tetramethylsilane molecule (c).
Some time ago Michel Verdaguer suggested me the analogy between the representation made by artist Joan Miró of stars, be them six- or eight-pointed, and the way coordination chemists schematically draw an octahedron. Some reflections on representation in art and chemistry, based on the discussions we had, can be found in a recent book [24], where an outstanding heptanuclear compound [25] created by the Jussieu group illustrates a sort of constellation formed by a central star surrounded by six more six-pointed stars (Fig. 9). It corresponds in fact to a central chromium atom connected via cyanide bridges to six nickel atoms.
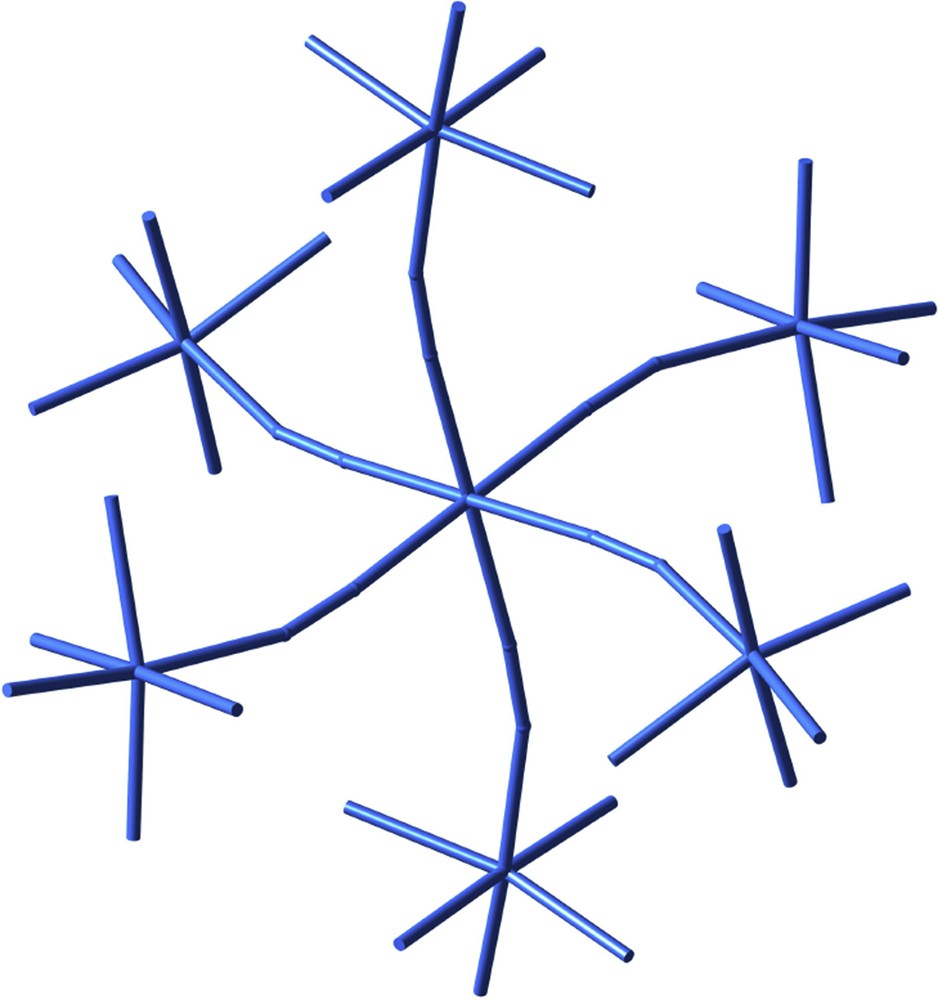
A cyano-bridged heptanuclear CrNi6 complex as a constellation of six-pointed stars.
6 Molecules that are just beautiful, funny or curious
Let me show here two examples of polyhedra surrounded by planar rings. One is a Pd13-centred cuboctahedron with its square faces covered by C7H7+ tropylium ions whose centres form an octahedron [26] (Fig. 10a). Related structures showcase a tetrahedron of tropylium ions, each on top of a vertex of a tetrahedral Mo4 cluster [27]. In contrast, with the larger COT (C8H82−) ligands one can get at most four tetrahedrally arranged rings around the titanium atoms of a Ti4Cl4 cubane (Fig. 10b) [28].
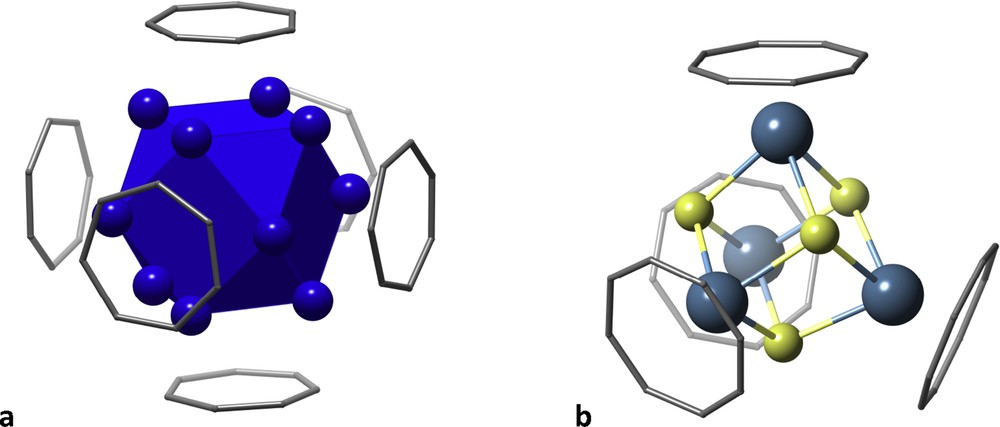
Large aromatic rings covering metal clusters: (a) tropylium ions on a Pd13 cluster and (b) COT2− anions on a Ti4Cl4 cubane.
Some molecules that are highly interesting for their architecture and bonding can also be associated with funny or curious shapes. Three examples are shown here. The extensive double bond systems of two β-carotene molecules, which are responsible for the orange colour of carrots, have been coordinated to a Pd102+ chain [29], altogether presenting the aspect of a hot dog sandwich (Fig. 11a). A hamburger sandwich, on the other hand, has a molecular representation in the Pd4(COT)2+ cation [30] (Fig. 11b).
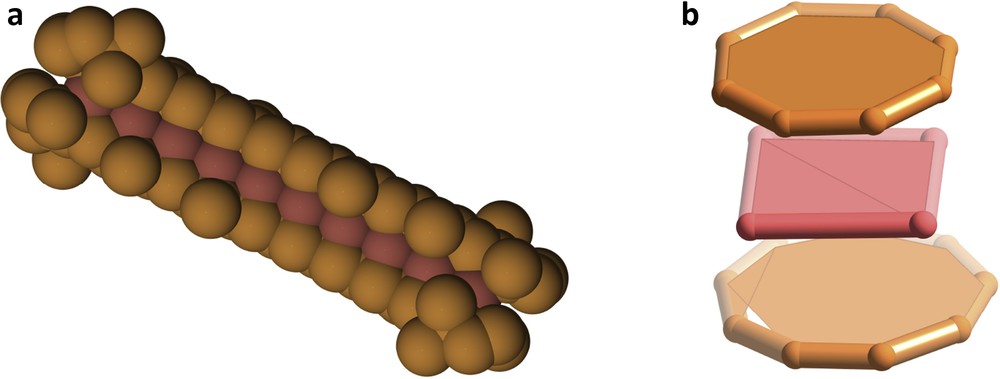
Two molecular sandwiches: a hot dog sandwich (a) and a hamburger sandwich (b) in which the meat is made of palladium atoms.
Our-third example is an organometallic Au(I) complex coordinated by two cyclopropenyl groups [31]. The N (i-Pr)2 groups hanging from the cyclopropenyl rings make the ligands look like two frogs, and they face each other to kiss at the metal atom, indeed a golden kiss (Fig. 12).

A bis(cyclopropenyl)Au(I) complex representing two kissing frogs.
7 Sticky arene rings
One of the design tools extensively developed in the past years has been the use of terphenyl groups forming a metal–carbon bond to the central ring, in such a way that the flanking arenes provide steric protection to the metal, enhanced by substituents R and R′ that are commonly Me, i-Pr or t-Bu (Fig. 13a). A relevant success of this strategy is showcased by the first synthesis of a quintuple bond between two chromium atoms, obtained by Nguyen et al. [32], subtended by only two such terphenyl groups (scheme in Fig. 13b and crystal structure in Fig. 14). Although initially the role of the flanking arene rings was assumed to be only to provide steric protection, it is now clear that besides preventing the approach of further ligands to the metal centre, they are actually π-coordinated to a metal atom in both quadruple Mo-Mo and quintuple Cr-Cr bonds [33,34], a phenomenon often referred to as “secondary interactions”.

Ligands with a terphenyl group that form a carbon–metal bond to a single metal centre (a) or to a multiply bonded metal (b) and related amido- and thiolato-terphenyl ligands (c).
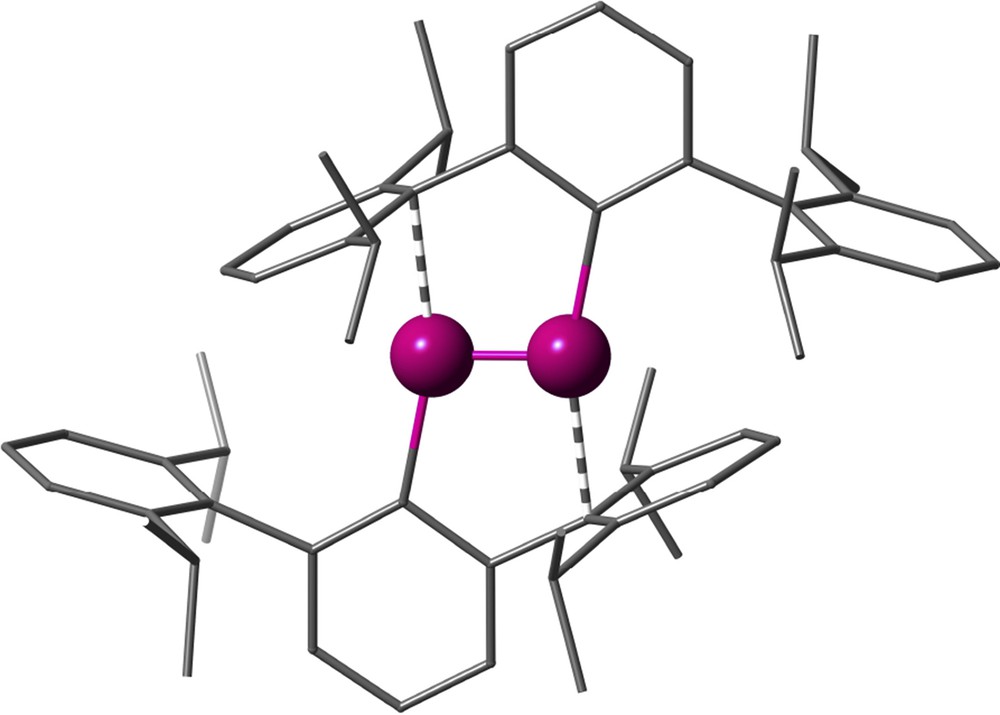
Crystal structure of the first reported compound with a quintuple Cr-Cr bond [32].
A further step in this direction has been the addition of a coordinating group such as amido [41,43,44] or thiolato [35] to the central phenyl ring, in such a way that the terphenyl group is somewhat separated from the metal atom whereas at the same time moving it laterally (Fig. 13c).
In all of the complexes with those ligands, the flanking arene rings act as sticky flaps that try to compensate the electronic unsaturation of nearby metal centres through π-coordination with varying hapticities, which are apparently affected by the nature of the R and R′ substituents. All of the structures can be classified in one of the following three cases (Table 1): (1) when both R and R′ are i-Pr groups, the MX2 core is linear and one flanking arene from each terphenyl makes a low hapticity contact (η1 to η3) with the metal (Fig. 15a), although for Zn(II) and high-spin Mn(II) these contacts are at relatively long distances; (2) when R is Me and R′ is Me or H, the MX2 core is bent and one flanking arene from each terphenyl group interacts with the metal in an η2 or η3 mode, resulting in a pseudo-tetrahedral coordination (Fig. 15b), and (3) when R is i-Pr and R′ is H, the MX2 core may be either linear or bent, in which case one of the flanking arene groups coordinates in an η6 mode (Fig. 15c). Although it seems clear that the substituents have some influence on the choice of one of the three alternative structures, one should not rule out the possible existence of stereoisomers.
Geometry of the MX2 core of bis(terphenylthiolato) and bis(terphenylamido) (X = S and NH, respectively) complexes and hapticity of the flanking arene rings, together with the ranges of close interatomic M···C distances.
Stereochem | M | R | R′ | X-M-X (°) | Hapticity | M···C (Å) | Σcova | Refcode | Reference |
X = S | |||||||||
a | Cr | i-Pr | i-Pr | 180 | 2 × 1 | 2.50 | 2.15 | jarjoc | [35] |
a | Mn | i-Pr | i-Pr | 180 | 2 × 3 | 2.95–3.33 | 2.37 | jarjui | [35] |
a | Co | i-Pr | i-Pr | 180 | 2 × 1 | 2.66 | 2.26 | jarket | [35] |
a | Ni | i-Pr | i-Pr | 174 | 2 × 1 | 2.64–2.66 | 2.00 | jarkix | [35] |
a | Zn | i-Pr | i-Pr | 180 | 2 × 1 | 2.80 | 1.98 | jarkod | [35] |
a | Zn | i-Pr | H | 180 | 2 × 3 | 2.96–3.28 | 1.98 | lextoa | [36] |
b | Fe | Me | H | 117 | 2 × 2 | 2.46–2.92 | 2.28 | vilhuu | [37] |
b | Fe | Me | Me | 122 | 2 × 3 | 2.47–2.87 | 2.28 | piyzec | [38] |
b | Zn | Me | Me | 152 | 2 × 3 | 2.65–3.06 | 1.98 | ligbae | [39] |
c | Fe | i-Pr | H | 120 | 1 × 6 | 2.48–2.61 | 2.28 | lexsuf | [36] |
c | Co | i-Pr | H | 92 | 1 × 6 | 2.08–2.19 | 2.26 | lextam | [36] |
c | Ni | Me | Me | 95 | 1 × 6 | 2.07–2.30 | 2.00 | foqxit | [40] |
X = NH | |||||||||
a | Cr | i-Pr | H | 180 | 2 × 1 | 2.63 | 2.15 | sebqib | [41] |
a | Cr | i-Pr | i-Pr | 180 | 2 × 1 | 2.48 | 2.15 | sebqex | [41] |
a | Fe | i-Pr | i-Pr | 180 | 2 × 2 | 2.79–2.94 | 2.28 | wulweg | [42] |
b | Co | Me | Me | 144 | 2 × 1 | 2.56–2.57 | 2.26 | sebwih | [41] |
b | Ni | Me | Me | 155 | 2 × 1 | 2.56–2.57 | 2.00 | sebwon | [41] |
b | Mn | Me | Me | 138 | 2, 3 | 2.60–2.87 | 2.37 | wugcad | [43] |
b | Fe | Me | Me | 141 | 2, 3 | 2.59–2.90 | 2.28 | wulwac | [42] |
a Sum of covalent radii of the high-spin metal atom and a carbon atom.
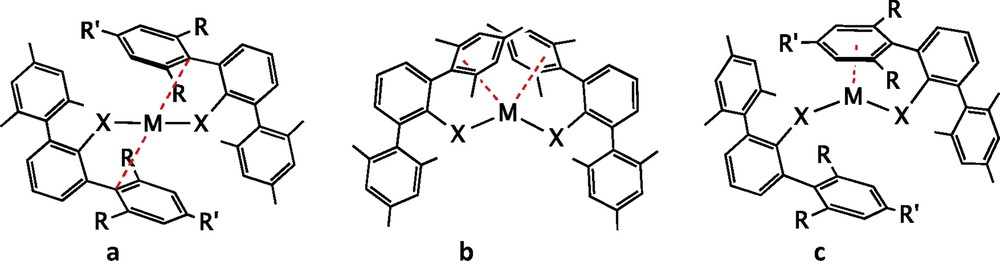
Different stereochemistries of formally dicoordinated transition metal atoms with two amido- (a and b) or thiolato-terphenyl (c) ligands.
8 Conclusions
We chemists probably feel more comfortable with 2D representations of the atomic connectivities in molecules than with their spatial arrangement. In part because more than one million crystal structures determined so far, including small molecules, proteins and extended solids, represent only a small fraction of the total number of chemical compounds known. For that reason, many interesting molecular shapes go unnoticed except for the small group of specialists in a particular area of chemistry. Of course, there are exceptions, and from time to time some innovative or beautiful molecules become widely known, as was the case for buckminsterfullerene, and for the widely developed families of supramolecular knots, rings and cages [45]. Many of the less famous molecules commented in this article have been detected only after hours of careful observation of the molecular structures contained in structural databases such as the Cambridge Structural Database (CSD) and the Inorganic Crystal Structure Database (ICSD). In some cases, the creators of new molecules themselves have called our attention in their communications in a particular way in which we can see them, which helps our visual memory to remind them for the rest of our life.
Acknowledgements
Financial support from MINECO CTQ2015-64579-C3-1-P, the Spanish Structures of Excellence María de Maeztu program, through grant MDM-2017-0767, and AGAUR, Generalitat de Catalunya, through grant 2017-SGR-1289 is gratefully acknowledged.