1 Introduction
Inorganic supramolecular chemistry of octacyanometallate 〚M(CN)8〛3–/4– (M = Mo and W) molecular building blocks is currently an area of extensive research 〚1–8〛. The driving force for the research in this field is the generation, by deliberate design, of functional molecular networks with predetermined structures and potential for technologically useful molecule-based electronic, magnetic and photomagnetic applications. The design and control of the structure of multidimensional networks based on octacyanometallate 〚M(CN)8〛3–/4– molecular building blocks is also the area of great fundamental structural interest and importance. We do not intend however to cover here the very important and expanding research area of the ‘low-oxidation’ organometallic chemistry of cyanometallates 〚9〛.
By means of crystal engineering, solid-state multimetal arrays can be constructed by using coordinative, π–π stacking, hydrogen bonds interactions, electrostatic forces and Van der Waals interactions 〚10–20〛. The rational design of such molecular architectures may be achieved using 〚M(CN)8〛3–/4– as the bridging moiety to build a multidimensional structure with a second coordination centre by thoughtful choice of ligands, transition metals, and reaction conditions.
Our successful synthetic strategy leading to heteropolymetallic systems with extended structures consists in self-assembly processes involving substitutionally inert octacyanometallate anions or ligand-substituted octacyano complex 〚W(CN)6(bpy)〛2– (bpy = 2,2’-bipyridine), and complex cations with labile coordination sites.
In each case, the information for the self-assembly of the entire structure is encoded into the individual building blocks due to their steric, topological and intermolecular bonding capabilities. Furthermore, the inherent coordination preferences of the metal cation used in the assembly process are coupled with the stereochemical non-rigidity of octacyanometallate moiety 〚21–24〛. Polytopal rearrangements of three idealised eight-coordination geometries: square antiprism (SAPR–8), dodecahedron (DD–8) and bicapped trigonal prism (TPRS–8) are found to be barrierless.
As a part of our programme of crystal engineering, we are devising and studying octacyanometallate-based frameworks involving M–CN–M’ linkage, in order to acquire the control over the way molecules assemble in the solid state. The control over the structure allows us to manipulate the properties of these supramolecular materials.
Herein, we will examine the structures according to the dimensionality of the observed structures based on 〚M(CN)8〛3–/4– molecular building blocks and cationic precursors: 0D architectures generated by cis and trans octahedral precursors, 1D and 2D architectures generated by Cu(II) centres, as well as 2D coordination polymer generated by octahedral node of 〚Mn(H2O)6〛2+ and geometrically anisotropic 〚W(CN)6(bpy)〛2–.
2 0D (discrete) architectures generated by cis and trans octahedral precursors
The possible structures that might result from the metal-directed self-assembly of cis-protected octahedral complexes of transition metals are molecular square and 1D zigzag chain 〚13〛. We have used this strategy to synthesise hexanuclear cluster {〚MnII(bpy)2〛4〚MIV(CN)8〛2}·8 H2O (M = Mo, W) 〚25〛 and pentanuclear high-spin molecule 〚MnII(bpy)2〛〚MnII(bpy)2(H2O)〛2〚WV(CN)6〛2·7 H2O 〚26〛 based on cis-〚Mn(bpy)2(H2O)2〛2+. In the case of 〚MIV(CN)8〛4– building block, the hexanuclear cluster {〚MnII(bpy)2〛4〚MoIV(CN)8〛2}·8 H2O has octahedral geometry (Fig. 1). The Mo centres located at the almost orthogonal axis to the plane of the four Mn centres have four bridging and four terminal cyano ligands arranged in square antiprismatic geometry. The molecule is realised by two, mutually perpendicular, MnII2MoIV2 squares, sharing the Mo corners. The Mn atoms are hexacoordinate, having a distorted octahedral geometry with two bpy and two cis bent cyano-bridges. Each Mo atom has four bridging and four terminal cyano ligands arranged in a square antiprismatic geometry. The supramolecular architecture of the MnII4MoIV2 lattice is controlled by CN-bridging, intra- and inter-molecular π–π stacking (face-to-face and edge-to-face) interactions and extensive network of hydrogen bonds between terminal cyano ligands and the water molecules (Fig. 2).
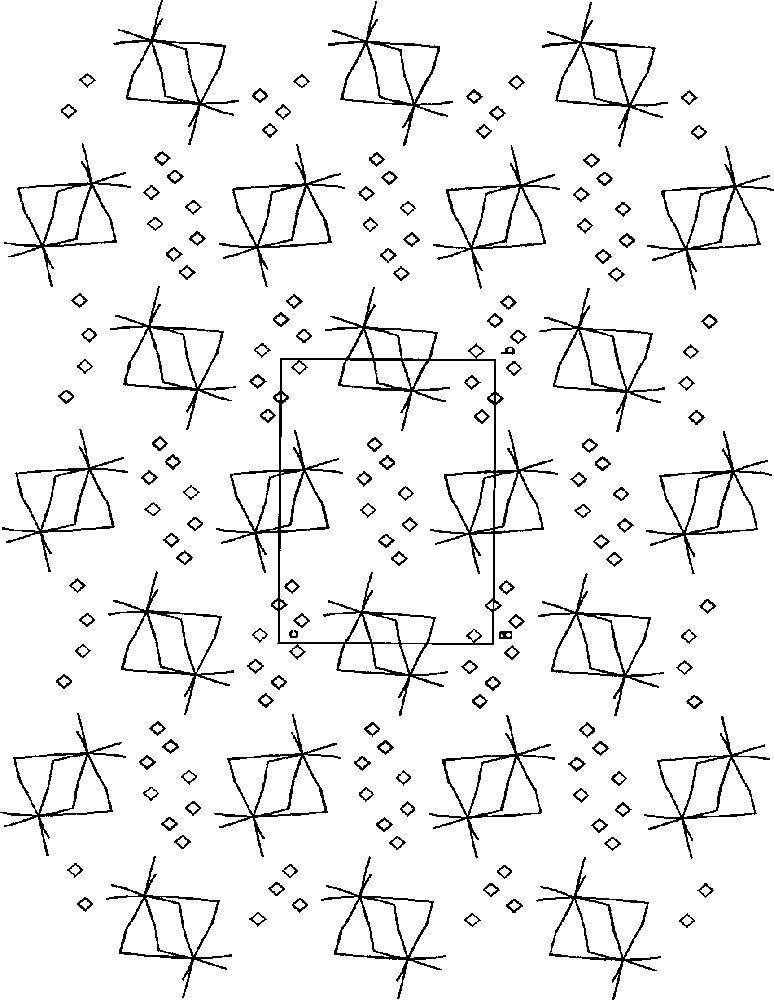
The projection of {〚MnII(bpy)2〛4〚MIV(CN)8〛2}·8 H2O (M = Mo, W) on the bc crystallographic plane showing packing of the hexanuclear Mn4Mo2 clusters (sticks) and H2O molecules (diamonds). The cluster has octahedral geometry with four Mn centres at equatorial positions and two Mo centres at axial positions. 2,2’-bipyridyl ligands are omitted for clarity 〚25〛.

The projection of {〚MnII(bpy)2〛4〚MIV(CN)8〛2}·8 H2O (M = Mo, W) on the bc crystallographic plane showing π–π stacking of bpy rings. Water molecules are omitted for clarity 〚25〛.
The self-assembly of cis-〚MnII(bpy)2(H2O)2〛2+ and 〚WV(CN)8〛3– precursors results in formation of pentanuclear 〚MnII(bpy)2〛〚MnII(bpy)2(H2O)〛2〚WV(CN)8〛2·7 H2O molecule 〚26〛. The pentanuclear molecule has the slightly distorted V-shape with two tungsten(V) atoms linked by single cyano bridges to three manganese(II) centres in alternating sequence Mn–W–Mn–W–Mn (Fig. 3). In the molecule, the central 〚Mn(bpy)2〛2+ moiety is bound to two 〚W(CN)8〛3– ions through a single cyano bridge. Each one of the 〚W(CN)8〛3– is coordinated also to terminal 〚Mn(bpy)2(H2O)〛2+ moiety through a single cyano bridge. The Mn atoms are hexacoordinate and present a distorted octahedral geometry. 〚Mn(bpy)2〛2+ moiety contains either two cis cyano bridges in the central Mn unit or single cyano bridge and aqua ligand in cis arrangement in the terminal one. Each W atom has two bridging and six terminal cyano ligands arranged in a square antiprismatic geometry slightly distorted towards bicapped trigonal prism geometry. The bridging CN ligands exhibit almost linear W–C–N units and significantly bent Mn–N–C sequences.
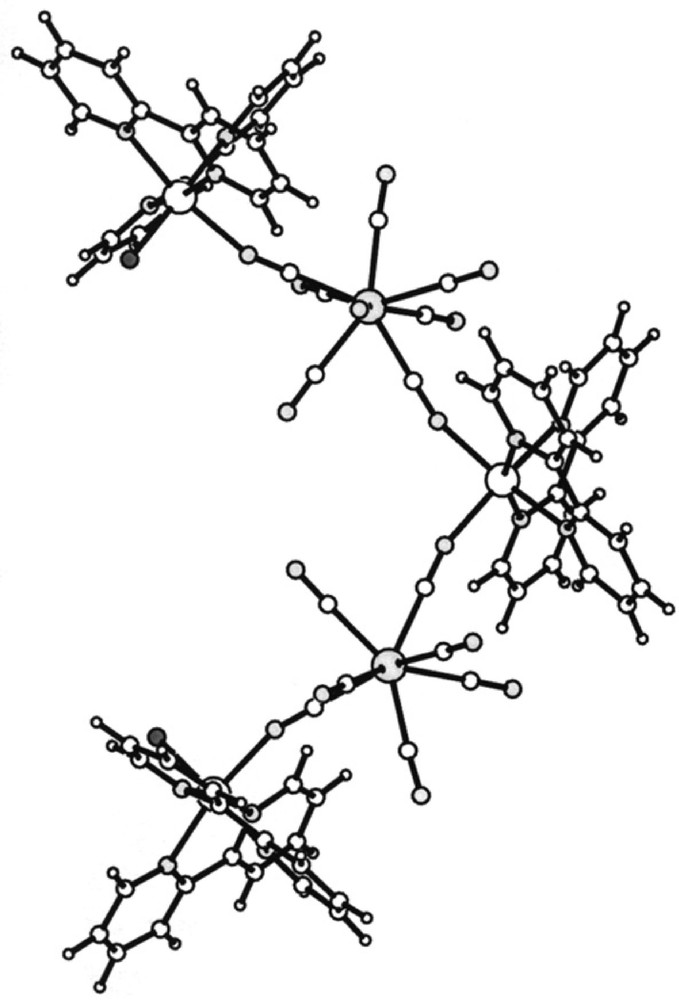
View of 〚MnII(bpy)2〛〚MnII(bpy)2(H2O)〛2〚WV(CN)8〛2·7 H2O molecule along the a crystallographic direction; empty big spheres: Mn(II); grey big spheres: W(V); empty small spheres: C; grey small spheres: N, black small spheres: coordinated H2O molecules 〚26〛.
In the crystal, the V-shaped molecules are packed into infinite columns due to head-to-head and arm-to-arm π–π stacking of the bpy rings of the terminal (‘arms’ of the pentamer) and central (‘head’ of the pentamer) Mn atoms of neighbouring molecules. Such an arrangement leads to a chain-like structure consisting of columns of pentamers clutched alternatively with their ‘heads’ and ‘arms’ (Fig.4) forming linear tubes, where H2O molecules are located (Fig. 5).
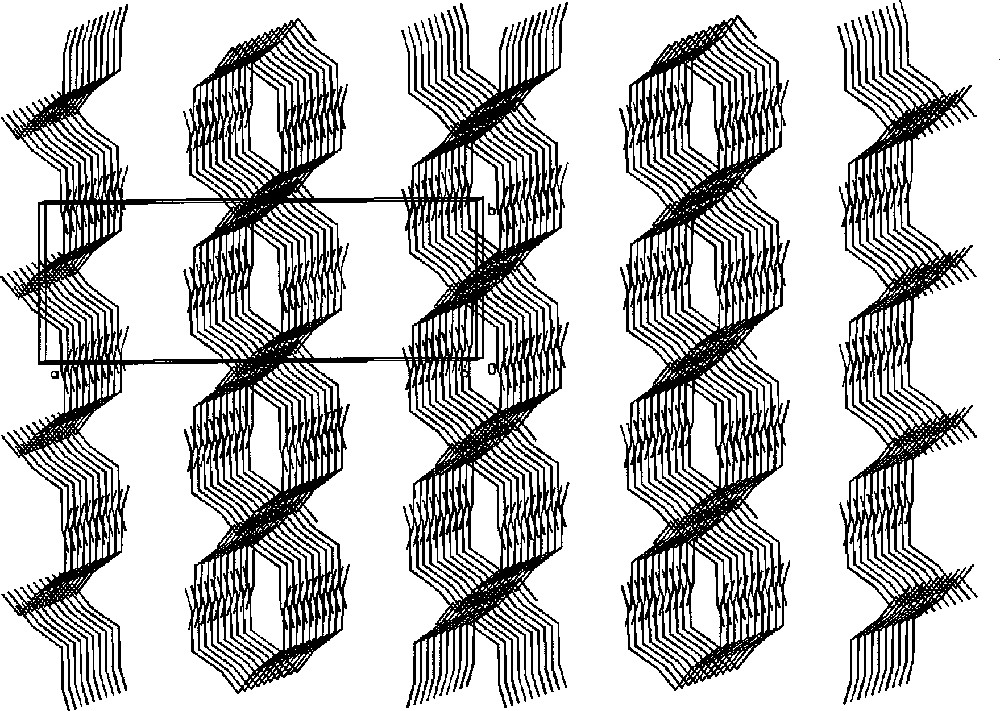
Part of the X-ray structure of 〚MnII(bpy)2〛〚MnII(bpy)2(H2O)〛2〚WV(CN)8〛2·7 H2O showing column chain-like ordering of the molecules along the c crystallographic direction (the aromatic rings are omitted for clarity) 〚26〛.

Part of the X-ray structure of 〚MnII(bpy)2〛〚MnII(bpy)2(H2O)〛2〚WV(CN)8〛2·7 H2O showing the channels parallel to the b crystallographic direction, in which the lattice H2O are located; Mn3W2 molecules: sticks; H2O molecules: diamonds 〚26〛.
The complex exhibits intramolecular antiferromagnetic coupling between Mn(II) and W(V) centres, consistent with a ground state spin of S = 13/2 and ferromagnetic long range transition at 0.66 K.
The preference for the formation of clusters in the self-assembly of cis-〚MnII(bpy)2(H2O)2〛2+ and 〚W(CN)8〛3–/4– is enforced by the electroneutrality of resulting {〚MnII(bpy)2〛4〚MIV(CN)8〛2}·8 H2O and 〚MnII(bpy)2〛〚MnII(bpy)2(H2O)〛2〚WV(CN)6〛2·7 H2O products as well as to the π–π stacking of bpy ligands, which seems to prevent the growth of the larger structures.
The self-assembly of 〚Co(tren)Cl2〛Cl〚tren = tris(2-aminoethylene)amine〛 and K4〚W(CN)8〛·2 H2O affords K2{〚CoIII(tren)〛2〚WIV(CN)8〛2}·9 H2O 〚27〛, containing tetranuclear square {〚Co(tren)〛2〚W(CN)8〛2}2– bridged by potassium ions into a two-dimensional array (Fig. 6). The tetranuclear square consists of alternating 〚CoIII(tren)〛 and 〚WIV(CN)8〛 corners. The Co(III) atoms are hexacoordinate and present a distorted octahedral geometry. Two cis bridging CN– in 〚Co(tren)(NC)2〛+ exhibit bent Co–N–C sequences with the angle ranging from 166(1)° do 173(1)°. Each W(IV) centre has two bridging and six terminal cyano ligands arranged in a slightly distorted square antiprismatic geometry. The {〚Co(tren)〛2〚W(CN)8〛2}2– squares are linked by potassium cations involving two terminal cyano ligands of each W centre to form a two-dimensional layer structure. The C–N–K bridges are significantly bent with the angle ranging from 148(1)° do 164(1)°. Formation of the layered structure by –CN–K–NC– linkage conforms to the analogous phenomena in some cyano-bridged networks based on hexacyanometalates 〚28, 29〛. The K+ centres bind additionally to solvent water to give seven- and six-coordinate K+ ions. One of the water molecules forms the bridge between potassium ions leading to an additional triangular WK2 motif of the bridged layer. The given layer and neighbouring layer related by (–x, –y + 1, –z + 1) symmetry are linked in pairs by cyano groups coordinated to potassium ions. This results in formation of bilayers connected by centrosymmetric square units made of seven coordinated K+ and one of 〚W(CN)8〛4– corners of {〚Co(tren)〛2〚W(CN)8〛2}2– square (Fig. 7). Layers are also linked through extensive network of hydrogen bonds involving interactions between cyano ligands, tris(2-amminoethylene)amine NH2 groups, and water molecules. The preference for the layered structure is attributed to the presence of potassium cations and hydrogen bonds, which are involved in aggregation with terminal cyano ligands. Therefore, the countercation and solvent can be used to control the dimensionality of the lattice.
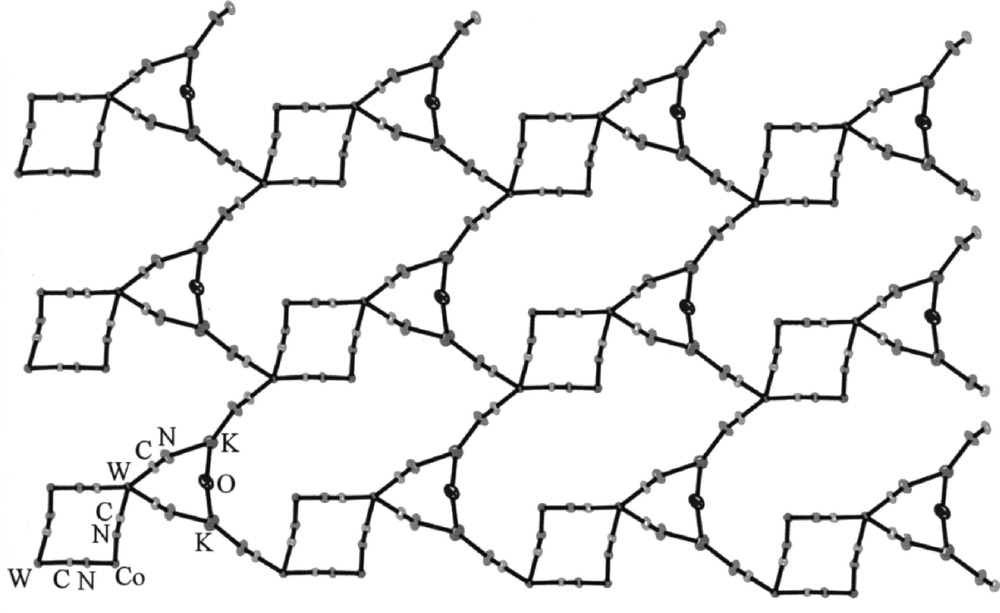
View of the layer in K2{〚CoIII(tren)〛2〚WIV(CN)8〛2}·9 H2O on the bc crystallographic plane, showing tetranuclear squares linked by potassium cations and bridging water molecules (the tren ligand, terminal CN ligands and non-bridging water molecules are omitted for clarity) 〚27〛.
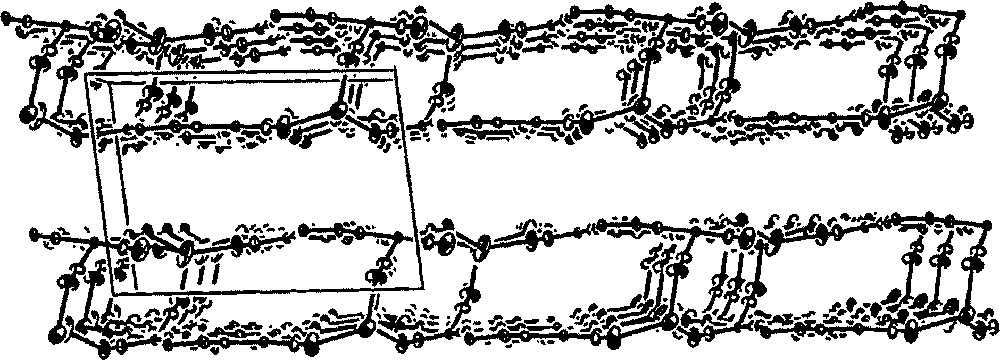
Packing diagram of K2{〚CoIII(tren)〛2〚WIV(CN)8〛2}·9 H2O along the b crystallographic direction, which shows bilayer arrangement of the crystal structure (the tren ligand, terminal CN ligands and water molecules are omitted for clarity) 〚27〛.
The trans-directional bonding approach along with redox reactivity of Pt(II) centre 〚30〛 has been applied by us to the construction of cyano-bridged 〚(CN)7MoIV–CN–PtIV(en)2–NC–MoIV(CN)7〛4– 〚31〛 (en = ethylenediamine) species. The trinuclear complex can be assembled by employing the inner-sphere electron-transfer process between Pt(II) and 〚Mo(CN)8〛3–. On the process of crystallisation, the mixed-cation crystals of Cs2〚PtIV(en)2Cl2〛〚(CN)7MoIV–CN–PtIV(en)2–NC–MoIV(CN)7〛·10 H2O are formed.
In the trimeric anion, octacyanomolybdate(IV) units are bonded to 〚PtIV(en)2〛 moiety through trans CN-bridges. The CN-bridges are characterised by bending of the Pt–N–C array (Fig. 8). The 〚Mo(CN)8〛4- has strongly distorted antiprismatic shape. The extended crystal structure reveals the alternating columns of trimers and isolated 〚Pt(en)2Cl2〛2+ cations (Fig. 9). The important feature of the network is the presence of large Cs+ cations trapped within the ‘cage’ formed by Cl, N and O atoms of 〚Pt(en)2Cl2〛2+, terminal CN ligands and water molecules, respectively. This arrangement indicates a crucial role of Cs+ in stabilisation of the structure in addition to the three-dimensional hydrogen bonds network.
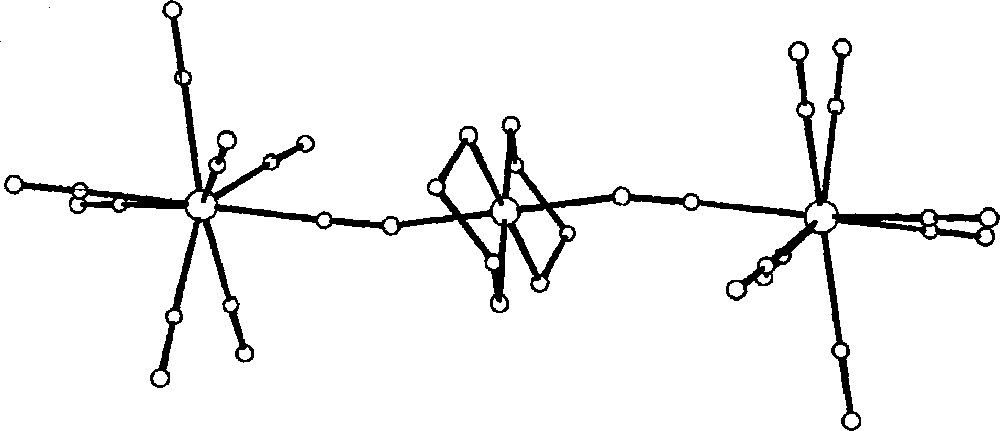
View of the 〚(CN)7MoIV–CN–PtIV(en)2–NC–MoIV(CN)7〛4– trimeric species in Cs2〚PtIV(en)2Cl2〛〚(CN)7MoIV–CN–PtIV(en)2–NC–MoIV(CN)7〛·10 H2O showing bent cyano-bridges 〚31〛.
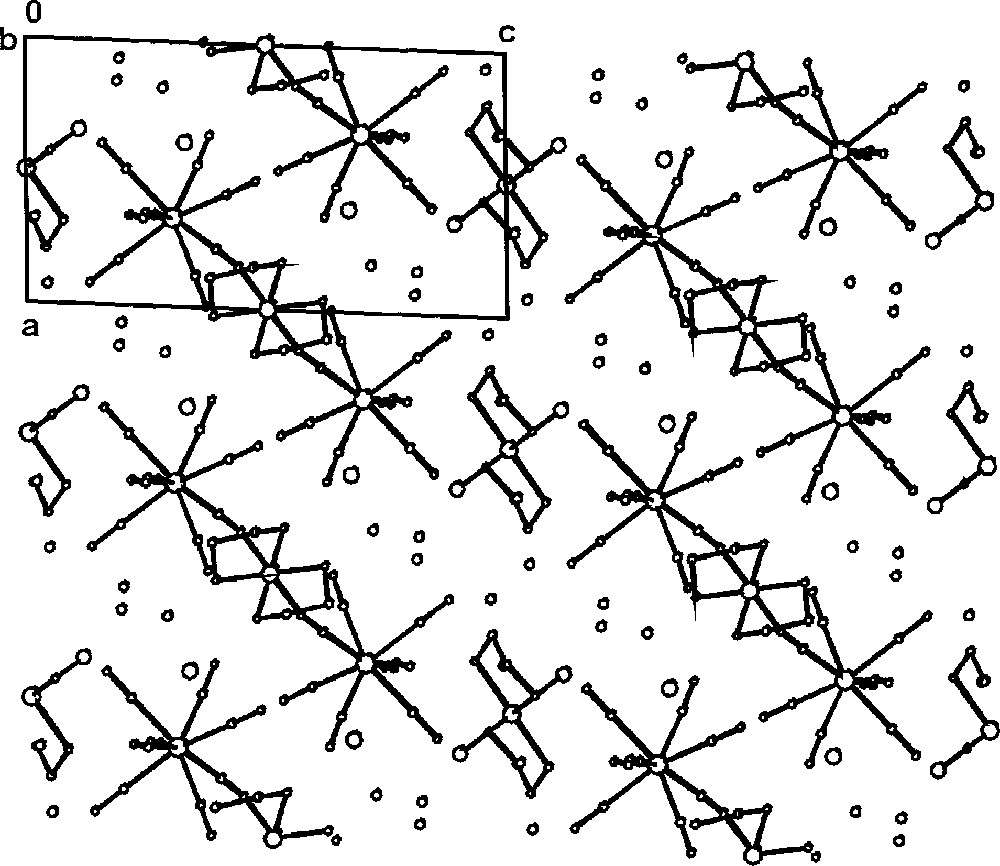
Packing diagram of Cs2〚PtIV(en)2Cl2〛〚(CN)7MoIV–CN–PtIV(en)2–CN–MoIV(CN)7〛·10 H2O along the b crystallographic direction, showing 〚(CN)7MoIV–CN–PtIV(en)2–NC–MoIV(CN)7〛4– trimeric cyano-bridged species, 〚PtIV(en)2Cl2〛2+ and Cs+ cations and lattice H2O molecules 〚31〛.
The reaction of trans-directing 〚MnIII(salen)H2O〛+ 〚salenH2 = N,N’-bis(salicylidene)ethylenediamine〛 with 〚WIV(CN)8〛4– resulted in the formation of 〚MnIII(salen)H2O〛3〚WV(CN)8〛·H2O 〚32〛 discrete species. The complex consists of trinuclear anion {〚MnIII(salen)H2O〛2〚WV(CN)8〛}– and 〚MnIII(salen)H2O〛+ countercation. Within the trinuclear unit, the W centre (template) of distorted square antiprism geometry has six terminal and two bridging cyano ligands, whereas the 〚MnIII(salen)H2O(NC)〛+ units display a distorted octahedral geometry (Fig. 10). The W centre is linked to two 〚Mn(salen)H2O〛+ moieties through its two adjacent cyano corners of triangular face of SAPR–8. The Mn–N–C sequences are exceptionally long and strongly bent. In the crystal, the V-shaped trinuclear anions and isolated 〚MnIII(salen)H2O〛+ cations are packed separately into infinite columns due to face-to-face π–π stacking of the salen rings. Such an arrangement leads to a broken wave-like structure (Fig. 11). The complex exhibits intramolecular antiferromagnetic coupling between Mn(III) and W(V) centres consistent with a ground state spin of S = 7/2 and ferromagnetic long range transition with TN = 6 K.

The structure of {〚MnIII(salen)H2O〛2〚WV(CN)8〛}– anion showing bent cyano-bridges 〚32〛.
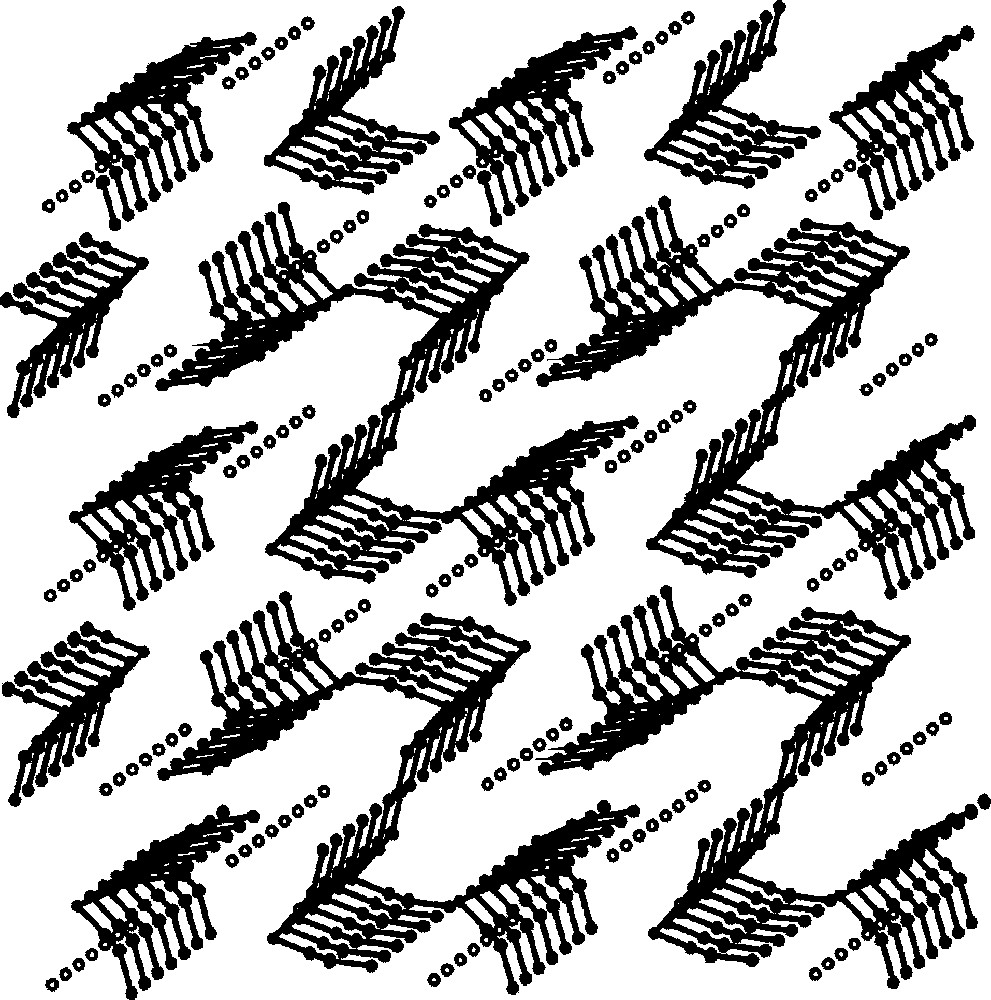
Perspective view of packing diagram of 〚MnIII(salen)H2O〛3〚WV(CN)8〛·H2O along the a crystallographic direction. Only the skeleton of {〚MnIII(salen)H2O〛2〚WV(CN)8〛}– and Mn centres of 〚Mn(salen)(H2O)〛+ countercations (separated empty spheres) are shown. Salen ligands and H2O molecules are omitted for clarity 〚32〛.
3 1D and 2D architectures generated by square-pyramidal Cu(II) centre
The strategy of exploiting square-pyramidal geometry of Cu(II) centre to generate cyano-bridged structures by connecting with octacyanotungstates(IV,V) has yielded 1D and 2D coordination polymers, with various Cu(II) moieties and architectures. The nature of the coordination of terminal ligand and the charge and geometry of octacyanides are the primary factors that determine the topology of the network.
The self-assembly of 〚CuII(dien)(H2O)2〛2+ (dien = diethylenetriamine) and 〚WV(CN)8〛3– in aqueous solution generates the one-dimensional chain {WV〚–CN–CuII(dien)–NC–〛45+}n whose 5+ charge is balanced by isolated 〚W(CN)8〛3– and Na+ counterions in Na{〚CuII(dien)〛4〚WV(CN)8〛}〚WV(CN)8〛2·8 H2O 〚33〛. The core of the chain (Fig. 12) is based on (i) square antiprismatic 〚W(CN)8〛3– with all cyano ligands involved in bridging to eight adjacent Cu(II) sites and (ii) 〚Cu(dien)(NC)2〛 units of square pyramidal geometry, where N-bonded cyanides occupy the axial and equatorial sites. The exact match of parallel squares of N-donors of the cyanides of W(V) sites to the squares of Cu(II) sites gives rise to a unique quadruple W-knotted cord pattern, formed by four bent threads of –CN–Cu(dien)–NC– sequences knotted at the W(V) centres. The geometry and orientation of copper centres limit the propagation of the polymer to one direction converging all threads to the neighbouring tungsten node of the chain.

Projection of the backbone of the chain {MV〚–CN–CuII(dien)–NC–〛4}n in Na{〚CuII(dien)〛4〚MV(CN)8〛}〚MV(CN)8〛2·8 H2O (M = Mo, W), along the b crystallographic direction; black big spheres: W(V), grey big spheres: Cu(II), black small spheres: N, grey small spheres: C 〚33〛.
Within the chain, the bridging 〚W(CN)8〛3– anion has slightly distorted square antiprismatic D4d local geometry. The copper presents a square pyramidal (SPY–5) coordination, where the three nitrogen donors of dien ligand occupy the equatorial positions. The 5-coordination of the Cu centre is completed by two N-donors of CN bridges from two W centres. The cyanide groups show different geometries: being short coordinated in the equatorial position of copper, but significantly longer at the axial position of the pyramidal Cu. These two sets of distances are related to two different non-linear geometries of W–C–N–Cu unit: being less bent in equatorial short CN bridges and significantly more bent in axial long CN bridges. These two distinguishable types of M–CN–Cu (M = nd cation) linkages are common for cyano-bridged assemblies based on (SPY–5) copper complexes 〚34〛. The projection of the molecular entity in the lattice is presented in Fig. 13. The isolated 〚W(CN)8〛3– ions are distorted from D4d geometry. The Na+ cations are located within the ‘cages’ formed by N atoms of isolated 〚W(CN)8〛3– and oxygen atoms of H2O. The lattice molecules of water are located within the ‘tubes’ formed by isolated 〚W(CN)8〛3–, parallel to the c axis, and around tungsten knots of the chain. They participate in the extensive hydrogen-bonding network between themselves, with the nitrogen atoms of the 〚W(CN)8〛3– anion and with the hydrogen atoms of the dien N–H groups. The molybdenum analogue Na{〚CuII(dien)〛4〚MoV(CN)8〛}〚MoV(CN)8〛2·8 H2O was found to be isomorphous.
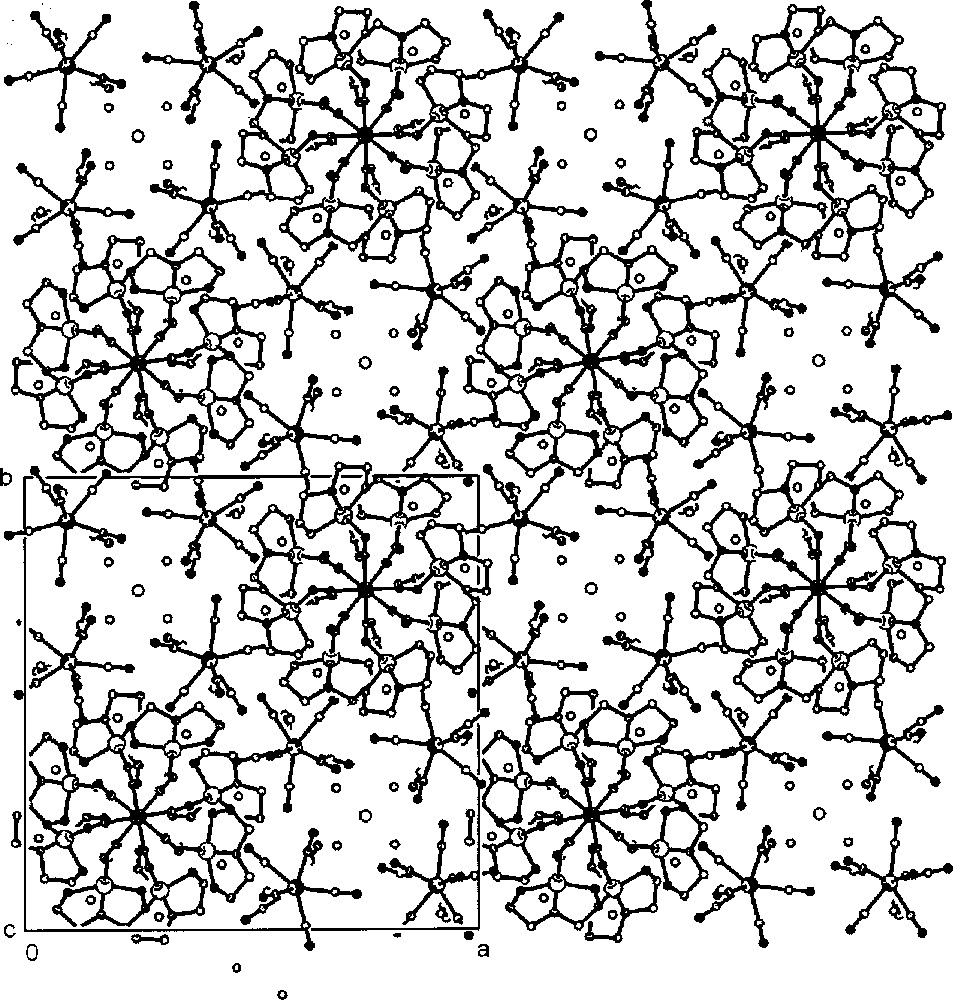
Projection of compound Na{〚CuII(dien)〛4〚MV(CN)8〛}〚MV(CN)8〛2·8 H2O (M = Mo, W) along the c crystallographic direction showing the chain, non-bridging 〚M(CN)8〛3- anions, Na+ cations and lattice water molecules: view parallel to the axis of the chain and perpendicular to the square planes of bridging 〚M(CN)8〛n– square antiprisms 〚33〛.
The magnetic behaviour is the one of two isolated paramagnetic 〚WV(CN)8〛3– entities and of WCu4 pentanuclear units, with a weak ferromagnetic intra-unit interaction between W and Cu and a very weak antiferromagnetic interaction between the WCu4 units. The weak ferromagnetic interaction is due to the orthogonality of the
The self-assembly of 〚CuII(dien)(H2O)2〛2+ and 〚WIV(CN)8〛4– in aqueous solution generates 2D layers of {〚CuII(dien)〛2〚WIV(CN)8〛·4 H2O}n 〚35〛. The crystal structure of {〚CuII(dien)〛2〚WIV(CN)8〛·4 H2O}n (Fig. 14) comprises strongly folded layers of square grid pattern (Fig. 15). Four tungsten centres are located at the corners and four Cu centres on the edges of the squares. The structure of the layer resembles of the egg holder with two W and two Cu atoms at the corners of the bottom and top, respectively. Each 〚WIV(CN)8〛4– of square antiprismatic geometry links four Cu centres. The four cyano bridges are formed by CN– groups occupying the corners of two adjacent triangular faces of SAPR–8. The 〚Cu(dien)(NC)2〛 units are of square pyramidal geometry with N-bonded cyanides occupying one axial and one equatorial site. Similarly, as in Na{〚CuII(dien)〛4〚WV(CN)8〛}〚WV(CN)8〛2·8 H2O, the equatorial bridge is shorter and less bent than the axial one. The remaining four terminal CN– ligands of 〚WIV(CN)8〛4–, standing out of the layer, as well as RNH2 groups from dien ligand and lattice water molecules are involved in the extensive network of hydrogen bonds stabilizing 3-dimensional architecture. The molybdenum analogue 〚CuII(dien)〛2〚MoIV(CN)8〛·4H2O was found to be isomorphous.

Packing diagram of {〚CuII(dien)〛2〚MIV(CN)8〛·4 H2O}n (M = Mo, W) along the b crystallographic direction showing 2-dimensional cyano-bridged polymeric layers and lattice H2O molecules (dots) 〚35〛.

View of the square-grid pattern in {〚CuII(dien)〛2〚MIV(CN)8〛·4 H2O}n (M = Mo, W) on the ab crystallographic plane; big empty spheres: M(IV); medium empty spheres: Cu(II); small spheres: C, N 〚35〛.
We have demonstrated that by controlling the charge of octacyanometalate moiety, Na{〚CuII(dien)〛4〚MV(CN)8〛}〚MV(CN)8〛2·8 H2O with 1D chain {MV〚–CN–CuII(dien)–NC–〛45+}n or 2D 〚CuII(dien)〛2〚MIV(CN)8〛·4 H2O neutral layers may be generated. In the self-assembly process of 〚CuII(dien)(H2O)2〛2+ and octacyanometalates, the interplay of charges and geometry of cationic and anionic building blocks are important factors influencing the topology of coordination polymers formed.
In order to elucidate the influence of the degree of chelation of terminal ligand, the 〚Cu(tetren)〛2+ (tetren = tetraethylenepentaamine) complex has been reacted with 〚WV(CN)8〛3- in aqueous solution at different pH 〚35〛. The 〚Cu(tetren)〛2+ is the single source of pre-programmed Cu(II) centres formed by protonation of N atoms of tetren ligand: penta-coordinated 〚Cu(tetren)〛2+ at pH > 6, tri-coordinated 〚CuII(tetrenH2)〛4+ in the pH range 3–5 and aqua complex of Cu(II) formed by the release of fully protonated tetren ligand at pH < 2.
The crystal structure analysis of the 〚Cu(tetren)〛(ClO4)2 precursor reveals that 〚Cu(tetren)〛2+ cation of distorted trigonal bipyramidal geometry has significantly longer terminal equatorial Cu–N bonds compared to the remaining central Cu–N bonds 〚36〛. The geometry of coordinatively saturated 〚Cu(tetren)〛2+ precursor implies that in the self-assembly process the terminal N–Cu bonds would be the first to be broken in favour of cyano bridges formation. Indeed, the reaction between 〚Cu(tetren)〛2+ and 〚WV(CN)8〛3– in Cu:W = 3:2 molar ratio in aqueous solution at pH 7.0 affords the assembly of 〚CuII(tetrenH2)〛4+. However, the obtained 〚CuII(tetrenH2)〛4〚WIV(CN)8〛4·10 H2O contains spontaneously reduced tungsten(IV) centre. The structure of generated compound is unique with cyano-bridged W2Cu2 squares joined by single cyano bridges into infinite one-dimensional polymer of necklace pattern (Fig. 16) 〚35〛. The W2Cu2 squares with alternative W–Cu corners comprise two different types. The one W2Cu2 subunit contains W centres of distorted dodecahedral (DD-8) geometry with three bridging cyano ligands and penta-coordinated Cu centres, whereas the other one consists of W centres with two bridging cyano ligands and hexa-coordinated Cu centres. The blocking tetren ligand is coordinated by three central nitrogen donor atoms to Cu atoms in equatorial positions, while the remaining terminal nitrogens of tetren are protonated and uncoordinated. The 〚CuII(tetrenH2)(NC)2〛2+ units are of square pyramidal geometry with bent N-bonded cyanides occupying the axial and equatorial sites. The mer-〚CuII(tetrenH2)(NC)3〛+ units are of distorted octahedral geometry with almost linear equatorial and strongly bent axial cyano bridges. The terminal amino groups of tetren ligand, terminal cyano ligands, and water molecules are involved in hydrogen bonds strengthening the internal structure of the chain.
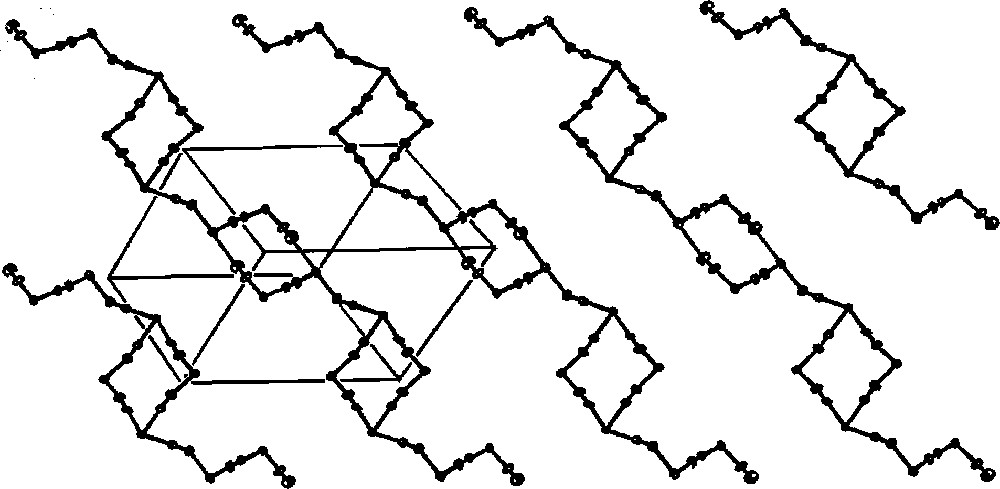
Cyano-bridged skeleton of 1D polymer of necklace pattern in 〚CuII(tetrenH2)〛4〚WIV(CN)8〛4·10 H2O (pH = 7). The skeleton is formed by W2Cu2 squares joined through CN– bridges. Lattice H2O molecules are omitted for clarity 〚35〛.
The same molecular building blocks: 〚CuII(tetrenH2)〛4+ and either 〚WV(CN)8〛3– or 〚WIV(CN)8〛4– in Cu:W = 3:2 molar ratio generates at pH 3.5 another type of the network superstructure: 〚CuII(tetrenH2)〛4〚WIV(CN)8〛4·10 H2O assembly forms unique 1D chain of long and short brick three-row wall pattern (Fig.17) 〚35〛. The wall comprises the fused W2Cu2 squares forming the central row and of W3Cu3 distorted rectangles forming two external rows. The W centres comprise two different types of distorted square antiprismatic structure: W2Cu2 subunit contains W centres with four bridging cyano ligands, whereas W3Cu3 rectangle contains W centres with four and two bridging cyano ligands. Cu sites are hexa-coordinate with mer-tetrenH2 ligand. The mer-〚CuII(tetrenH2)(NC)3〛+ in W2Cu2 subunit has bent cyano bridges, one equatorial and two axial ones. W3Cu3 rectangle contains mer-〚CuII(tetrenH2)(NC)3〛+ present in W2Cu2 as well as Cu site of short and almost linear equatorial CN bridge and significantly bent two axial bridges. The terminal cyano ligands of 〚W(CN)8〛4– are presumably involved in the network of hydrogen bonds linking parallel chains through the lattice water molecules.
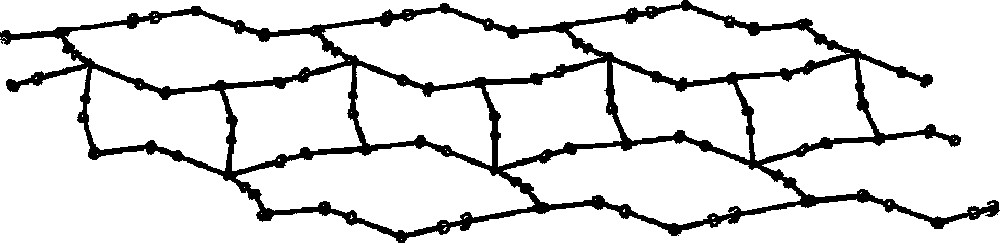
Cyano-bridged skeleton of 1D polymer of long and short brick three-row wall pattern in 〚CuII(tetrenH2)〛4〚WIV(CN)8〛4·10 H2O (pH = 3.5). The central row is formed by W2Cu2 squares and the external rows are formed by W3Cu3 distorted rectangles. Lattice H2O molecules are omitted for clarity 〚35〛.
Both compounds of the formula 〚CuII(tetrenH2)〛4〚WIV(CN)8〛4·10 H2O obtained for different pH conditions exemplify the structural supramolecular isomerism 〚13〛: the components of the network remain the same but they have a different superstructure. The networks are effectively different compounds. The preference for the either structural supramolecular isomer can be attributed to the substitutional reactivity of 〚Cu(tetren)〛2+ at different pH. Therefore, the pH can be used to control the topology of the assemblies.
The self-assembly of 〚W(CN)8〛3– and 〚Cu(tetren)〛2+ in acidic aqueous solution generates {(tetrenH5)0.8CuII4〚WV(CN)8〛4·7.2 H2O}n 〚36〛. The 〚Cu(tetren)〛2+ complex ion provides the pre-programmed bare Cu(II) centres formed by release of fully protonated tetren ligand. The {(tetrenH5)0.8CuII4〚WV(CN)8〛4·7.2 H2O}n consists of anionic double-layers {CuII4〚WV(CN)8〛44-}n, the charge of which is balanced by tetrenH55+ cations (Fig. 18). Each 〚W(CN)8〛3– of bicapped trigonal prism geometry links five Cu centres, coordinated by (NC) bridges in a square pyramid. Four slightly bent equatorial cyanide bridges Cu–N–C gives rise to the square-grid pattern of the single layer, while the practically linear axial CN– bridge joins two layers and thus forms the double-layer (Fig. 19). In consequence, the double-layer is realised by the two stacked sheets of edge sharing Cu–(NC–W)5 square-pyramids of antiparallel orientation. The cation and the inferred water molecules lie in planes between the Cu–W double layers.

Projection of double layers in {(tetrenH5)0.8CuII4〚WV(CN)8〛4·7.2 H2O}n on the ab crystallographic plane: big black spheres: M(IV); big empty spheres: Cu(II); small black spheres: N; small empty spheres: C. The disordered tetrenH55+ cations and H2O molecules are omitted for clarity 〚36〛.
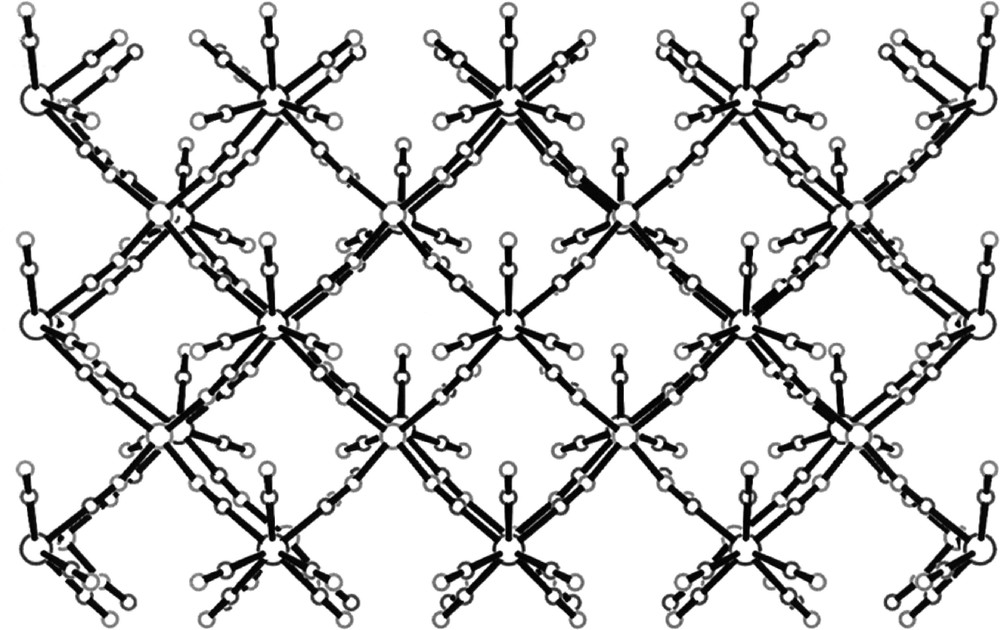
Projection on the ac crystallographic plane showing square-grid pattern of single double-layer in {(tetrenH5)0.8CuII4〚WV(CN)8〛4·7.2 H2O}n. The disordered tetrenH55+ cations and H2O molecules are omitted for clarity 〚36〛.
The remaining three terminal CN ligands of 〚W(CN)8〛3–, standing out of the layer, are presumably involved in the extensive network of hydrogen bonds linking parallel double-layers through the lattice water molecules and are undoubtedly significant in construction of the system. The {(tetrenH5)0.8CuII4〚WV(CN)8〛4·7.2 H2O}n exhibits soft ferromagnetic behaviour, with an ordering temperature TC of 34K. The ferromagnetic coupling can be rationalised in terms of the interactions of unpaired electrons originating from the mutually orthogonal 3dx2-y2 orbital of Cu(II) and the mixture of 5dz2 and 5dx2-y2 ground state orbitals of the W(V) centre of bicapped trigonal prism geometry along both types of Cu–NC–W bridge.
4 2D coordination polymer generated by octahedral node of 〚Mn(H2O)6〛2+ and geometrically anisotropic 〚W(CN)6(bpy)〛2–
For highly symmetrical 〚M(CN)8〛n– units, the design and synthesis of geometrically and magnetically anisotropic polynuclear systems encounter large difficulties 〚4b, 37〛. These observations prompted us to explore the possibility of lowering the symmetry of octacoordinated cyano building block by using the molecular precursor related to the octacyanotungstate(IV) geometrically anisotropic 〚WIV(CN)6(bpy)〛2– complex 〚38, 39〛. Our strategy is convergent on the new synthetic approach based on the replacement of a highly symmetrical hexacyanoferrate(III) ion by lower symmetry 〚FeIII(CN)4(phen)〛– 〚40〛 (phen = 1,10-orthophenantroline) and 〚FeIII(CN)4(bpy)〛– 〚41〛 precursors. In 〚W(CN)6(bpy)〛2–, which is not compatible with cubic geometry of hexacyano moieties, two coordination sites are occupied by bpy ligand acting as terminal coordinator and participating in secondary π-stacking interactions. Therefore, 〚W(CN)6(bpy)〛2– has the advantage of the formation of anisotropic, directional network over the homoleptic hexacyano complexes producing the highly symmetrical three-dimensional arrays.
To gain the evidence on the binding modes of 〚W(CN)6(bpy)〛2– with transition metal centres, we have studied the reaction of 〚Mn(H2O)6〛2+ with 〚W(CN)6(bpy)〛2–, and obtained the two-dimensional basket weave-like 〚11,13〛 coordination polymer {〚fac-(H2O)3MnII-(μ-NC)3-WIV(CN)3(bpy)〛·4.5 H2O}n 〚42〛 of 4.82 topology 〚43〛. There is only one previous example of such topology observed in a 2D parallel interpenetrated network of coordination polymer formed between Cd(NO3)2 and 2,4-bis(4-pirydyl)-1,3,5-triazine (dpt), 〚Cd2(NO3)4(dpt)2(MeCN)〛∞, which exists as a doubly interpenetrated 4.82 two-dimensional sheet in the solid state 〚44〛.
The self-assembly reaction of the 〚W(CN)6(bpy)〛2– precursor with 〚Mn(H2O)6〛2+ in aqueous solution yields a novel two-dimensional coordination polymer {〚fac-(H2O)3MnII-(μ-NC)3-WIV(CN)3(bpy)〛·4.5 H2O}n. The crystal structure of {〚fac-(H2O)3MnII-(μ-NC)3-WIV(CN)3(bpy)〛·4.5 H2O}n consists of neutral thick waved layers and separated by crystallisation water molecules (Fig. 20). Within each layer, 〚W(CN)6(bpy)〛2– forms three single cyano-bridges to three fac-〚MnII(H2O)3〛 (Fig. 21). Each fac-〚MnII(H2O)3〛 is linked to three 〚W(CN)6(bpy)〛2–. The CN-bridges are significantly bent. The Mn and W centres linked alternatively by single cyano bridge afford spatially distorted small tetranuclear W2Mn2(μ-CN)4 and large octanuclear W4Mn4(μ-CN)8 polygons that run along the layer. The face-to-face π-stacked 〚W(CN)6(bpy)〛2– pairs control the formation of polygons and give rise to the basket weave-like pattern (Fig. 22). Aqua ligands of fac-〚Mn(NC)3(H2O)3〛 and non-bridging cyano ligands of 〚W(CN)6(bpy)〛2– are involved in the extensive network of hydrogen bonds linking parallel layers through the crystallisation water molecules and are significant in the construction of the system.
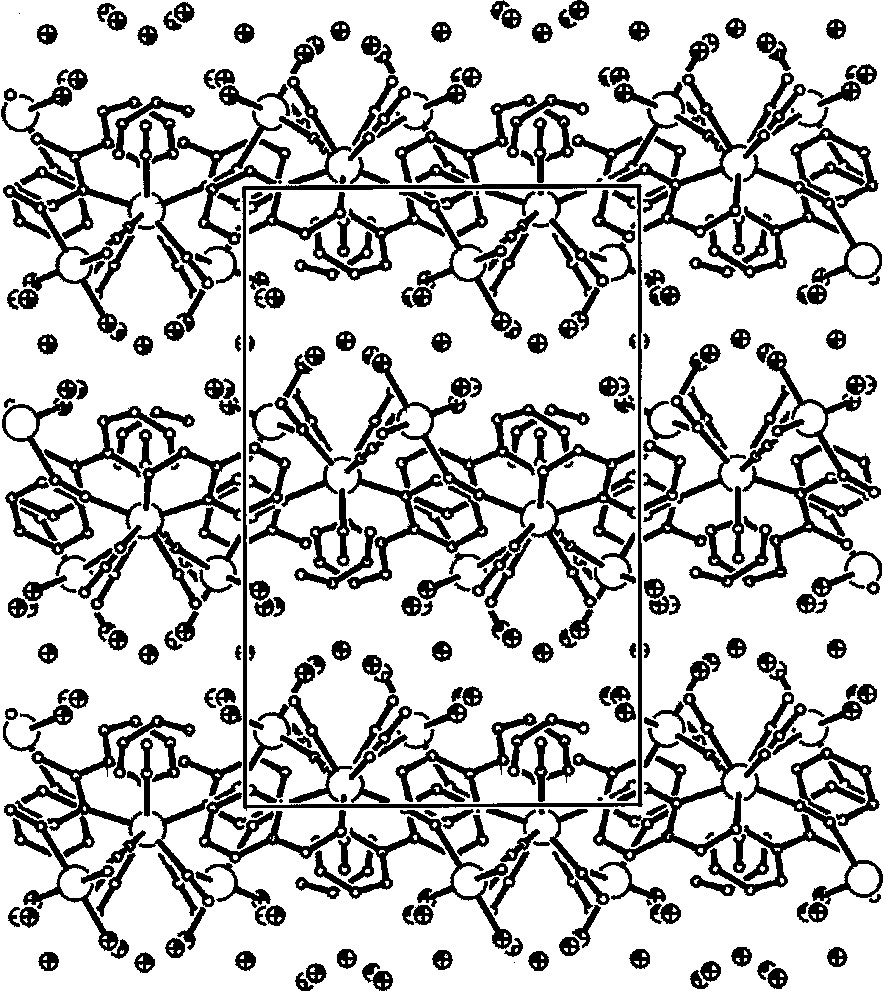
Packing diagram of {〚fac-(H2O)3MnII–(μ-NC)3–WIV(CN)3bpy〛·4.5 H2O}n along the b crystallographic direction, showing the thick waved layers separated by crystallisation water molecules 〚42〛.

View of a representative section of the 2D lattice of {〚fac-(H2O)3MnII–(μ-NC)3–WIV(CN)3bpy〛·4.5H2O}n parallel to the ab crystallographic plane. The layer is built of tetranuclear W2Mn2 and octanuclear W4Mn4 polygons 〚42〛.
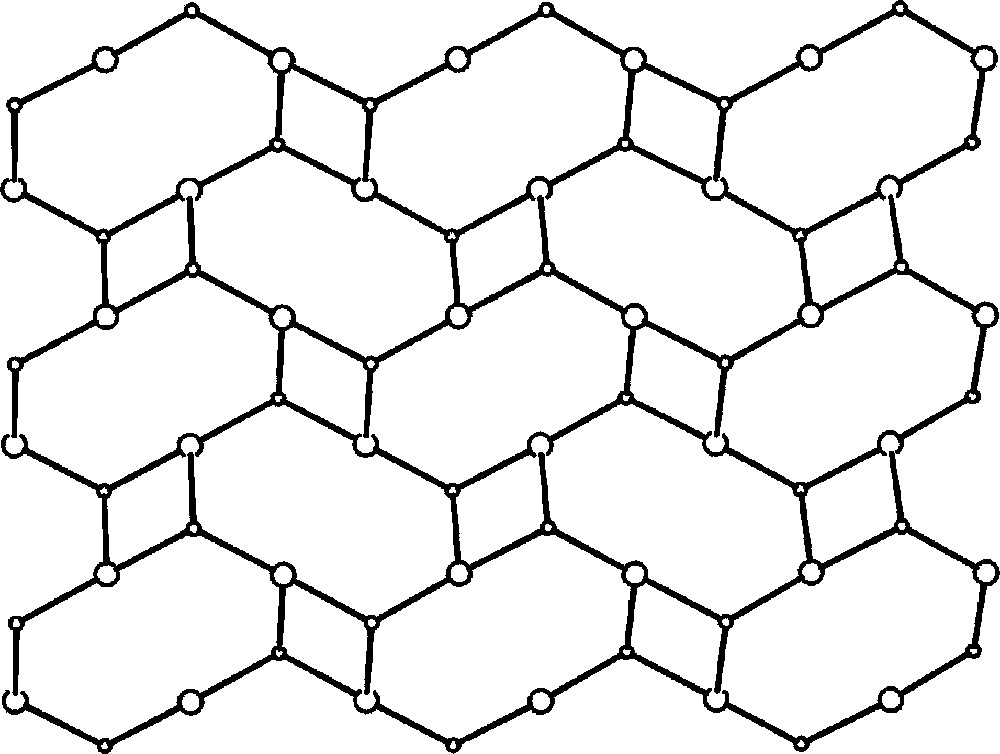
Basket weave-like pattern (4,82 topology) of {〚fac-(H2O)3MnII–(μ-NC)3–WIV(CN)3bpy〛·4.5 H2O}n 〚42〛.
5 Conclusions
We have demonstrated the principles of self-assembly applied towards the design and synthesis of discrete coordination architectures and polymeric coordination networks based on 〚M(CN)8〛3–/4– and cationic complexes as templates and nodes. The rational design of supramolecular structure exploits coordinative, π–π-stacking, hydrogen bonds interactions, electrostatic forces, and Van der Waals interactions. The systematic approach to increasing an understanding of the fundamental and subtle factors that control the architectures in the solid state has resulted in design, isolation, and characterisation of a number of novel compounds. Our results serve to emphasise the difficulty in predictably preparing coordination networks. The control over these materials is far from satisfactory. However, as our understanding increases about the numerous factors involved, supramolecular octacyanometallate-based coordination synthesis is likely to develop considerably further.