1 Introduction
Although the reactions of thiols and isocyanates have been known for a long time [1] and the resultant polythiourethanes (containing a structure unit as -NH-CO-S-) are used in some modern applications including coatings [2], adhesive and optical lenses [3], they are a relatively poorly developed group of polymeric materials, in contrast to their oxygen counterparts, i.e. polyurethanes, which gained a great industrial significance. One of the distinct advantages of the thiourethane chemical structural units is the feasibility of incorporating them into polymerizable systems which has the potential of opening up whole new application areas. In our previous papers [4–8], we proposed a similar approach to apply polythiourethanes (PTU) for curing epoxy resins. One of the major weakness of epoxy resins is their brittleness and low fracture toughness. Toughening can be achieved by flexibilizing the epoxy structure by the reduction of crosslink density or incorporating modifiers such as liquid rubbers or thermoplastic, which leads to increased plastic deformation. These methods generally cause a decrease in loss modulus and solvent resistance. The toughness and flexibility of the cured epoxy resin can by markedly affected, without sacrificing its other useful properties, by the application of suitable hardeners. For such a purpose, we used polythiourethane hardeners obtained by convenient synthesis methods from dithiols and diisocyanates [5,6]. This new type of epoxy resin hardener has an influence on the reactivity of the curing composition and also has strong impact on the chemical properties of the product. These hardeners revealed high reactivity toward curing epoxy resins, also at low-temperature condition (–10 °C) and impart excellent adhesion of the resin to the metal substrate. We found that incorporation of polythiourethane curing agents into epoxy resin enhanced the values of impact resistance and tensile strength. The key limitation in the practical use of various mercaptan compounds as crosslinking agents is their odour. By converting mercaptans into polythiourethane derivatives, we have succeeded in eliminating the unpleasant smell of thiols. Prepared polythiourethanes give homogeneous systems with epoxy resin and have good storage stability with other coreactants of epoxy formulation like diamines. The curing characteristic and thermal behaviour of the formulated curing mixtures with earlier studied linear polythiourethanes, indicate that epoxy/polythiourethane stoichiometry and thermal history during cure may greatly affect the curing mechanism and final properties of the epoxy network. The subsequent reaction of isocyanate groups liberated by heat of reaction with free amine and hydroxyl groups remaining in the condensed resin provide adequate points for epoxy crosslinking. The present work extends our studies on characterization of polythiourethane hardeners for epoxy resin prepared through the reaction of hexamethylene diisocyanate (HDI) and multifunctional thiols, whose branched structure may influence curing characteristic and final properties of the cured resin. The toughening mechanism and the relationship between the chemical structure of the polythiourethane hardeners and curing characteristic were discussed. The thermal degradation behaviour and climatic ageing resistance were also investigated.
2 Experimental
2.1 Materials
All organic reagents used in this study were supplied from Sigma-Aldrich and were used as received without further purification. The commercially available epoxy resin (diglycidyl ether of bisphenol A) Epidian-5 having epoxide number of 0.487 was obtained from Organika-Sarzyna Inc., Poland. The viscosity of the resin is 23.9 Ns/m2 at 25 °C.
2.2 General synthesis of polythiourethanes
Hexamethylene diisocyanate (HDI) and particular mercaptan in 2 molar excess, were put in a three-necked flask equipped with stirrer, nitrogen inlet, water-cooled condenser with a capped CaCl2 drying tube and thermometer. The reaction mixture was stirred and heated at temperature of 50 °C for 12 h. The end of reaction was detected by FTIR. Products were purified by column chromatography and analysed by 1H NMR.
2.3 Curing reactions and gel time measurement
Typically, a mixture of 5 g of epoxy resin and hardener was poured into a glass vial, and intensively stirred for 1 min with glass bar. The gel time was determined according to EN ISO 179-1:2000, at temperature of 25 °C. In this paper, gel times were the average of two samples. Curing compositions for prepared cured epoxy systems are presented in Table 2. For all cured samples, 0.01% of 2,4,6-tris(dimethylaminomethyl)phenol (DMP-30) was used as an accelerator.
Curing characteristics of epoxy/polythiourethane systems.
Amount of polythiourethane (phr)a | Ti (°C) | Tp (°C) | Tf (°C) | ΔH (J/g) | Gel time (min) | |
DODT-HDI | 20 | 175 | 212, 257 | 276 | 276.6 | 13 |
TTMA-HDI | 15 | 234 | 265, 275 | 294 | 283.6 | 18 |
PETMP-HDI | 10 | 220 | 256 | 283 | 248.5 | 20 |
a phr, parts per 100 parts of epoxy resin.
2.4 Gel permeation chromatography (GPC)
Molecular weights (Mn and Mw) were measured by GPC using a HP 1100 series liquid chromatograph, equipped with PLgel 5 μ Mixed-C column. Tetrahydrofuran was used as an eluent at flow rate 0.5 ml/min. Standard polystyrenes (Fluka) having Mw in the range of 400–2000000 g/mol were used as references for molecular weight evaluation.
2.5 DSC measurement
Differential scanning calorimetry (DSC) measurements were conducted using a Mettler Toledo apparatus. Sample of approximately 10 mg was used at a heating rate of 10 °C/min. DSC curves were recorded within a temperature range from 30 to 400 °C.
The curing thermal data were obtained by means of DSC1 Mettler Toledo differential scanning calorimeter heated from 25 to 350 °C. The instrument was calibrated using indium and n-octane. The epoxy resin was mixed with appropriate amount of a hardener and then degassed in a vacuum oven to remove the air included during the mixing process. After homogenization, a little amount of the composition (about 10 mg) was put into the DSC hermetic aluminum sample pan. The measurement was carried out under nitrogen atmosphere (20 ml/min) at a heating rate of 10 °C/min. To check the possibility of some postcuring reactions occurring, the second scan was conducted after cooling sample to room temperature.
2.6 Spectroscopy characterization
The FTIR spectra were measured with BIORAD FT-IR 175C spectrophotometer in an air atmosphere. 1H NMR measurements were performed on a Bruker Avance II Plus NMR spectrometer at 700.08 MHz, in deuterated toluene.
2.7 Swelling study
The powdered samples were swollen in 1,2-dichloroethane for total of 6 weeks. To prepare the samples for weighing and to maintain equilibrium conditions, excess solvent on the surfaces of the samples was removed, and then the samples were in contact with the vapours of a solvent for 24 h. Samples were weighed in sealed vials to reduce solvent evaporation. To determine the mass of the dry network after extraction of soluble materials, the samples were dried in vacuum oven at 50 °C for 2 days. Percentage of swelling was calculated for each sample as:
2.8 Mechanical testing
For the preparation of test specimens, the mixed samples of epoxy resin and hardener were degassed for a few minutes under vacuum at room temperature to remove any trapped air bubbles. To crosslink the samples, the mixtures were poured into a silicone-rubber mould.
The finally prepared samples were conditioned at 50 °C for 24 h before the mechanical tests and swelling measurements were performed.
The Charpy impact strength of the cured resin was determined according to EN ISO 179-1:2000. The dimensions of unnotched specimens were approximately 80 × 10 × 4 mm.
Tensile tests were performed by Zwick 1435 tensile testing machine according to EN ISO 527-1:1996. The dimension of the specimens was specified according to this standard.
Curing of the specimens and all mechanical tests were performed at room temperature and at least seven specimens were tested and the average results were reported with the standard deviation.
2.9 Ageing characteristics
Climatic ageing was carried out using a Ci4000 Xenon Weather-Ometer. The measurements lasted for 200 h and involved repetition of consecutive day and night segments with the following parameters: day segment (radiation intensity: 0.4 W/m2, temperature: 70 °C, moisture 70%, duration: 8 h), night segment (no UV radiation, temperature: 60 °C, moisture 60%, duration: 4 h).
3 Results and discussion
3.1 Synthesis and characterization of polythiourethanes
Three new polythiourethane oligomers were prepared and characterized according to the procedure presented in our earlier papers [5]. For all samples, the polymerization reaction was conducted at mercaptan/HDI molar ratio of 2. The resulting polymers were white solids, showed good solubility in common organic solvents such as tetrahydrofuran, DMF, and chloroform at room temperature. The solubility of the various epoxy resin hardeners is a limiting factor for uniform curing with amines or other coreactants. The solubility tests for all of the prepared polythiourethane hardeners showed very good solubility in both aliphatic and aromatic diamines. Molecular weight data of polyurethanes obtained by GPC are presented in Table 1. The data showed that polydispersity of synthesized oligomers decreased with increasing the functionality of mercaptan. This result is expected because the excess of the –SH groups limits the growing of the chains to high molecular weight. Broad molecular weight distribution of DODT-HDI indicates the presence of low-molecular-weight fraction which consists of “both-end blocked” hexamethylene diisocyanate.
Molecular weight distribution of polythiourethanes.
Mercaptan monomer | Polymer abbreviations | Molecular weight (g/mol) | PD | |
Mn | Mw | |||
3,6-dioxa-1,8-octanedithiol (DODT) | DODT-HDI | 3095 | 4926 | 1.59 |
Trimethylopropane tris(3-mercaptopropionate) (TTMA) | TTMA-HDI | 4945 | 5742 | 1.16 |
Pentaerythritol tetrakis(3-mercaptopropionate) (PETMP) | PETMP-HDI | 5054 | 5781 | 1.14 |
The chemical structure of the polymers was confirmed by FTIR. Fig. 1 shows IR spectra of synthesized polythiourethanes. The lack of absorption peaks of SH vibration at 2545 cm−1 and isocyanate group (–NCO) at 2272 cm−1, demonstrates the quantitative polyaddition of thiol and isocyanate groups. The characteristic vibrations of the thiourethane linkages at 1716–1731 cm−1 (nonbonded CO stretching), 1606 cm−1 (bonded CO stretching) 1507–1580 cm−1 (NH bending), 3301–3407 cm−1 (NH stretching), were observed for all samples.
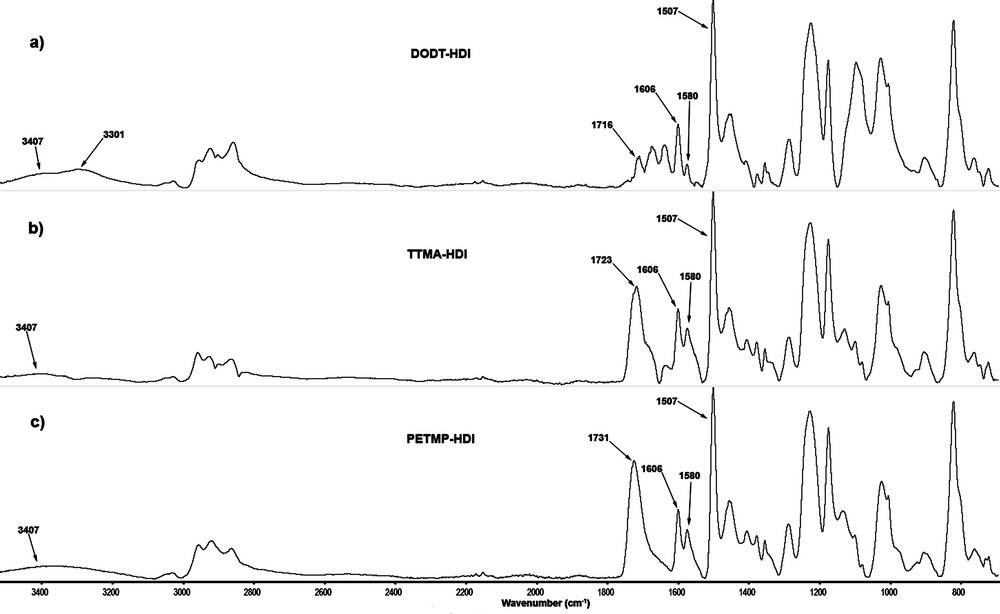
FT-IR analysis of polythiourethanes: (a) DODT-HDI; (b) TTMA-HDI; (c) PETMP-HDI.
Chemical composition of polymers was also proven by their 1H NMR spectra. No attempt has been made to assign all individual resonances in the rather complicated multiplets from the all polymers. All spectra show a peak at 7.0–7.1 ppm, which is due to the thiourethane N-H protons. Fig. 2 shows 1H NMR spectrum of PETMP-HDI sample. The average molar share of HDI and PETMP monomer units of 20:3, was calculated from (a) peak area of –NH- group in the HDI unit; and (b) peak of corresponding –CH2- group in PETMP units, for spectrum presented in Fig. 2. The composition of TTMA-HDI polymer calculated in a similar way, was HDI:TTMA = 16:3. In the case of linear DODT-HDI polymer, the content of HDI mer units was much lower (HDI:DODT = 3:1), what indicates the presence of low-molecular weight fraction of oligomer particles ended with DODT units. It was confirmed by the GPC measurements which revealed a high polydispersity of this polymer (Table 1).

1H NMR spectrum of PETMP-HDI polymer.
3.2 IR characterization of cured epoxy resin
FTIR spectroscopy was utilized to investigate the chemical structure of different polythiourethane crosslinked epoxy resins. Fig. 3 shows IR spectra of epoxy resin cured with DODT-HDI, TTMA-HDI and PETMP-HDI hardeners at room temperature. The results strongly differentiate the cure potential for polythiourethanes prepared from bifunctional and multifunctional mercaptans. It is evident, from DSC curing plots of DODT-HDI cured sample (Fig. 3a), that curing mechanism involves the dissociation of thiourethane linkage and the reaction of released thiol and isocyanate groups with the oxirane rings. The absence of band at 1716 cm−1 clearly shows the decomposition of thiourethane moiety for this sample. The presence of characteristic absorption peak of isocyanate groups at 2260–2268 cm−1 and absorption of the thiol group at 2554 cm−1 (Fig. 3b,c) indicates that dissociation of thiourethane moiety occurs also for TTMA-HDI and PETMP-HDI cured samples. On the other hand, the remaining signals for thiourethane structure at 1731–1733 cm−1 suggests that for polythiourethane hardeners with high functionality, even a partial dissociation of thiourethane moiety could unbalance the stoichiometry of the epoxy curing. It is also clearly visible when we compare peak intensity of the free hydroxyl groups at about 3313 cm−1 for all samples. The presence of urethane bands at 1682 cm−1 gives proof for urethane crosslinking process. The free hydroxyl groups remaining in the condensed resin provide adequate points for epoxy crosslinking by the extremely reactive isocyanate groups, which are present in excess for TTMA-HDI and PETMP-HDI resin formulation. The band of –CH2-S- at 1054 cm−1 indicates reaction of released thiol groups with free epoxy rings.
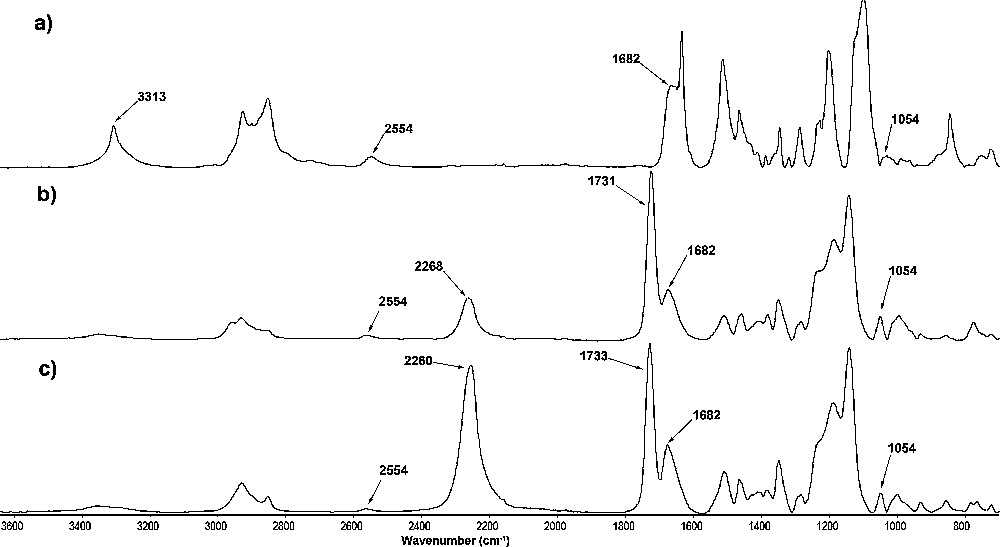
FT-IR analysis of the epoxy resin cured with polythiourethanes: (a) DODT-HDI; (b) TTMA-HDI; (c) PETMP-HDI.
3.3 Investigation of the curing reaction
The curing reaction of epoxy resin with polythiourethane hardeners were monitored with DSC. Fig. 4 shows the DSC thermograms of curing processes with different PTU-hardeners, the temperature of the curing onset (Ti), the exothermic peak position (Tp), temperature of completion of the exotherm (Tf) and the total reaction heats obtained from the DSC exotherms are shown in Table 2. The cure conditions were also determined from gel time measurements (Table 2). The exothermic peaks denote the proceeding of the curing reaction between oxirane ring and thiol group of polythiourethane oligomers. The reactivity of PTU-hardeners toward the epoxy could be read from the start point of the exothermic peak, and the results were presented in Table 2. The temperature of curing onset of DODT-HDI is considerably lower than for TTMA-HDI and PETMP-HDI cured samples. This result was reasonable since the incorporation of the branched PTU-oligomers reduces reactivity of the curing system owing to the effect of steric hindrance. Also, the endset of the exotherms shifted to higher temperatures and the longer values of gel time, confirming such explanation for reactivity differences of branched crosslink agents. As we reported before [5], the single exothermic peak during crosslinking with PETMP-HDI, could reflect that curing mechanism involves the reaction of thiol groups with epoxide, or that thiourethane moiety can react directly with the epoxy group with subsequent cyclization to an oxazolidone. However, the lack of characteristic absorption peak of oxazolidone at 1756 cm−1 at IR spectra, seems to exclude the second possibility, and together with the presence of urethane and isocyanate absorption peaks, could indicate that isocyanate is released continuously, and the subsequent formation of the polyurethanes occurs without any significant thermal effect. An additional rise in temperature appeared distinct from the main exotherm observed for DODT-HDI and TTMA-HDI cured samples, along with high value of enthalpies, showing clearly that these hardeners increase the initial temperature of curing and bring about vigorous reaction of isocyanate groups. The participation of thiourethane moiety dissociation step, which provide tightly crosslinked structure by the “co-curing” reaction of extremely reactive isocyanate groups, is reflected in differences of mechanical properties and ageing resistance between samples curing with PTU and standard polyamines.

DSC thermograms on the curing reactions of epoxy resin with polythiourethanes: (a) DODT-HDI; (b) TTMA-HDI; (c) PETMP-HDI.
3.4 Thermal properties of the cured epoxy resins
The thermal analysis of cured epoxy resins is very useful in understanding their molecular architecture. DSC measurements allow to characterize the behaviour of the final form of the resin composition under various environmental conditions and decomposition mechanisms.
The DSC scan plots for PTU-cured epoxy samples are presented in Fig. 5, the glass transition temperature (Tg) values are reported in Table 3. In contrast to our earlier results [7], the observed single Tgs indicate continuous phase morphology for all specimens.
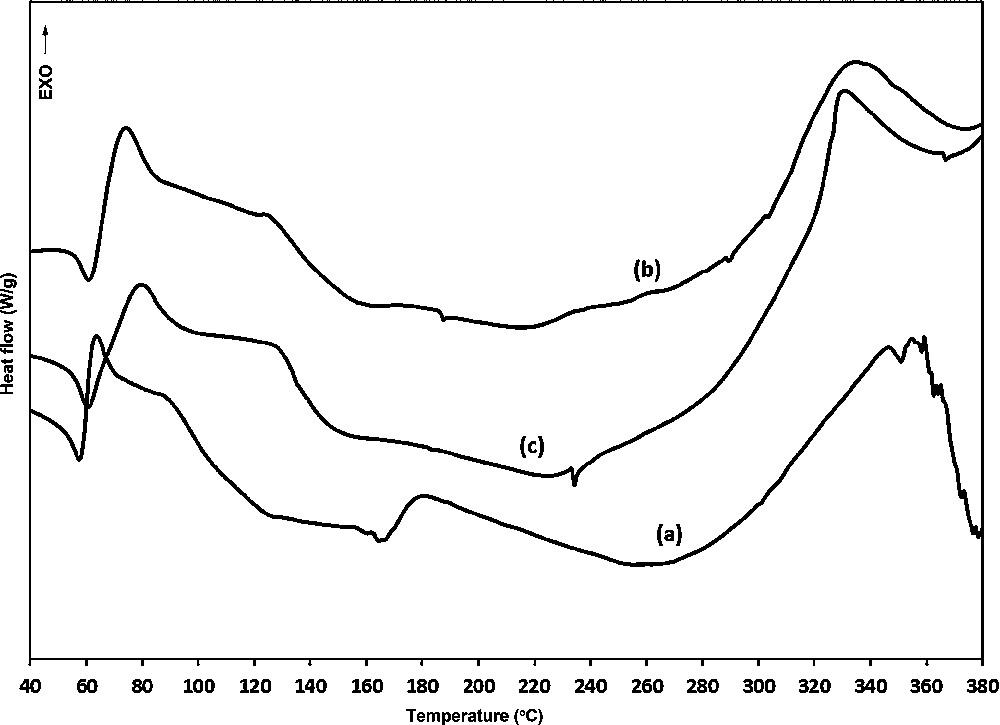
DSC thermograms of epoxy resin cured with polythiourethanes: (a) DODT-HDI; (b) TTMA-HDI; (c) PETMP-HDI.
Thermal and mechanical properties of the epoxy/polythiourethane systems.
Curing agent | Tg (°C)c | Swelling value (%) | Impact strengthd (kJ/m2) | Tensile strengthd (MPa) | Elongation at breakd (%) |
TETAa | 10.1 (6,2) | 28.7 (15.8) | 3.0 (1.9) | ||
DODT-HDIb | 101 | 61 | 24.6 (22.1) | 34.2 (30.8) | 16.2 (13.8) |
TTMA-HDIb | 136 | 56 | 20.3 (17.3) | 38.7 (36.0) | 12.4 (11.3) |
PETMP-HDIb | 138 | 50 | 15.1 (14.3) | 46.3 (44.9) | 9.1 (8.3) |
a Reference specimen cured with 12 phr of triethylenetetraamine (TETA) at room temperature, without of DMP-30 accelerator.
b Composition of epoxy/polythiourethane systems, as in Table 2.
c Determined from DSC measurements.
d Values in brackets represent the mechanical properties after climatic ageing.
The low Tg for DODT-HDI cured sample can be explained both by the presence of ether linkage in crosslinked matrix, as well as by the increased flexibility caused by uncrosslinked polyurethane and polythiourethane content. Introducing PTU synthesized from multifunctional mercaptans enhanced the Tg. The branched structure of TTMA-HDI and PETMP-HDI oligomers results in cured resins with high crosslink density, consequently to higher the Tg of the resins, respective to their functionality. The exothermic transitions observed for specimen cured with DODT-HDI at about 180 °C might be due to the formation of a new compounds as a result of dissociation of thiourethane and urethane linkage or degradation processes. The endo/exothermic transitions below glass transition temperatures observed for all samples in the range 50–85 °C, presumably indicate melting and exothermic decomposition of polythiourethane/urethane fraction, which is not covalently linked with cured epoxy network. The decomposition temperatures of all specimens were similar, suggesting that incorporating of branched polythiourethane oligomers into curing formulation did not apparently alter the thermal stability of the epoxy resins.
3.5 Mechanical properties and ageing resistance
One of the main objectives of this study was to elucidate the influence of the chemical structure of polythiourethane hardener on the thermo-mechanical properties and ageing resistance of epoxy networks. The impact strength and tensile properties of the cured epoxy resin specimens are summarized in Table 3. All PTU-cured samples exhibit higher impact resistances and tensile strength than those epoxy resin cured with standard diamine hardener. Confirmed by IR spectroscopy and DSC studies, toughening mechanism include the increase of crosslink density and rigidity imparted by urethane linkages generated after dissociation of these thiourethane moiety-rich polymers. Among the PTU-cured samples, the TTMA-HDI and PETMP-HDI hardeners gave the epoxy castings with highest tensile strength. It is evident that PETMP-HDI has the best toughening effect on the epoxy resin. The branched structure and high functionality of this hardener result in a stiffer network structure with greater crosslink density and with a higher ultimate strength. On the other hand, highly stiff structures can be less fracture resistant and even brittle. The higher impact resistance of DODT-HDI cured resin may be explained by the flexible spacer of DODT. Also, the highest value of elongation at break of this specimen is probably related to low crosslink density and incorporation of long ether chains, which improve the flexibility of epoxy network. The long polythiourethane chains act as reactive diluents, and tend to inhibit formation network linkages, due to lower functionality of this hardener. For samples cured with TTMA-HDI and PETMP-HDI, reduction in the values of elongation at break may be explained due to the increased crosslinked density of the epoxy matrix. To evaluate the crosslinking of the epoxy resin, swelling of the samples in 1,2-dichloroethane was performed. It is not possible to obtain quantitative crosslink density values by using swelling measurements and the Flory-Rehner equation; however, such results correlate qualitatively to those crosslink density measurements obtained via other routes [9]. As shown in Table 3, the values obtained by this procedure, confirm the correlation between the degree of crosslinking of epoxy resin and the branched structure of polythiourethane hardeners.
In our previous paper [6], we have reported that application of polythiourethane hardeners to epoxy resins contributes to the good chemical resistance properties of the coatings interacting with various chemicals. Epoxy resins have excellent mechanical properties in the initial state. However, when operating in environmental conditions, ageing processes occur. These can significantly deteriorate the complex of stress-strain properties and can make individual components of the epoxy product unusable. In this work we present our preliminary results concerning the evaluation of climatic ageing resistance performed by measuring changes of mechanical properties. Analyses of the mechanical properties after ageing indicate that in all samples cured with PTU-hardeners, ageing resistance increased in comparison to reference specimen cured with standard amine curing agent. As shown in Table 3, as the ageing proceeds, the mechanical properties such as impact strength and tensile strength were reduced. Ageing test results show that decrease of these properties for the specimens cured with polythiourethanes was less significant, as observed in the case of sample cured with standard amount of diamine hardener. The variation in mechanical properties was similar for all PTU-cured samples, which indicated that ageing resistance is not affected by differences in crosslink density caused by branched structure of multifunctional PTUs. A search is now under way to correlate the ageing phenomena with chemical structure of polythiourethane cured epoxies. Further investigation of the ageing of PTU-cured epoxy resins, including studies of changes in molecular structure and characterization of thermal and chemical resistance, will be reported at a later stage.
4 Conclusions
Three new types of polythiourethanes were synthesized and successfully developed as a curing agents of epoxy resin. The influence of the molecular structure of polythiourethane curing agents on curing characteristics and final properties of epoxy resin were studied. The DSC measurements indicated that the reaction exotherm, curing rate and glass transition of the product, can be changed by the presence of polythiourethane hardener with different functionality. The results of this study indicate that the initial stoichiometry of the formulation and thermal condition, related with functionality of used polythiourethane curing agent, are of critical importance in governing the mechanism of the network formation and the final properties of these epoxy compositions. The polythiourethane cured epoxy resins were found to have improved physico-mechanical properties compared to that observed in the case of epoxies cured with standard diamine hardeners. High functionality and branched structure of TTMA-HDI and PETMP hardeners had been proved to serve effectively the toughening of epoxy resins due to the increased crosslink density. Application of these efficient crosslinking agents also increased Tg values. However, impact strength and flexibility of cured resins were decreased by the resultant loss of chain mobility. Measurements of mechanical properties of cured samples showed that the incorporation of polythiourethanes into epoxy resin, greatly improves the ageing resistance of this material. The synthesized polythiourethane hardeners are therefore suitable to be used in epoxy systems for application in many fields where high resistance to environmental factors and excellent thermo-mechanical properties are required.
Acknowledgement
This work was supported by the National Science Centre (Poland) (grant No 0255/B/H03/2011/40).