1 Introduction
Multicomponent reactions (MCRs) have emerged as a vital field of chemistry and a powerful tool in modern synthetic organic chemistry, allowing the facile synthesis of complicated compounds in a one-pot reaction without the isolation of any intermediates. Clearly, for multi-step synthetic procedures the number of reaction and purification steps is among the most important criteria for the efficiency and practicability of the process and should be as low as possible. Therefore, it has drawn the attraction of organic chemists to develop novel MCRs for a broad range of products [1].
On the other hand, pyrrole nucleus is a versatile and valuable building block for a number of biologically and pharmaceutically active compounds (Fig. 1). A range of pharmacological properties, such as antibacterial, antitumor, anti-inflammatory, antioxidant, anti-anginal and antifungal activities of this important class of heterocycles are found in the literature [2].
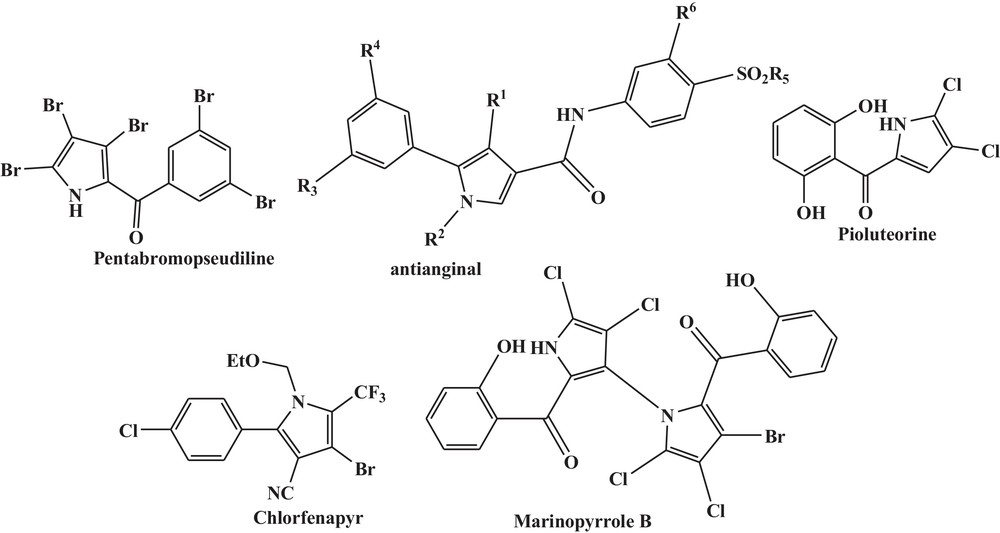
Some pyrrole-containing bioactive compounds.
The known used routes for synthesizing these compounds are Hantzsch [3], Knorr [4], and Paal–Knorr [5] reactions. However, most of these methods employ expensive catalysts, multi-steps synthetic operations, long reaction times or harsh reaction conditions. Thus, it is clearly evident that the need for the development of new and flexible methods is required. Recently, literature has highlighted the importance of nanosized materials in several scientific and technological areas, and many research councils have intensified investments in nanotechnology for the coming years [6]. The surface of metal oxides, such as TiO2, Al2O3, ZnO, CuO and MgO, exhibits both Lewis acid and Lewis base character [7]. They are excellent adsorbents for a wide variety of organic compounds and increase the reactivity of the reactants. In any metal oxide, surface atoms make a distinct contribution to its catalyst activity. In powder particles, the number of surface atoms is a large fraction of the total. The high surface area to volume ratio of metal oxide nanoparticles is mainly responsible for their catalytic properties [8]. High yield, selectivity and recyclability have been reported for a variety of nanocatalyst-based organic reactions [9]. Nano copper oxide is certainly one of the most interesting metal oxides, because it has surface properties, which suggest that a very rich organic chemistry may occur there [10]. Although a few number of new pyrrole syntheses, including MCRs [2c], have been reported in recent years, no example is known for the synthesis of highly substituted pyrroles, using CuO nanoparticles.
In the context of our general interest in the synthesis of heterocycles [11] and following our research on nanoparticles applications in organic synthesis as nanocatalysts [12], herein, we propose a facile synthesis of highly substituted pyrroles using CuO nanoparticles as an efficient, commercially available and non-toxic nanocatalyst via a four-component reaction (Scheme 1). A joint of experimental and DFT calculations was used to confirm the structure of (1-benzyl-4-(4-chlorophenyl)-2-methyl-1H-pyrrol-3-yl)(phenyl)methanone 14 and 1-(1-benzyl-4-(4-chlorophenyl)-2-phenyl-1H-pyrrol-3-yl)ethanone 14′. It was shown that DFT methods perform NMR spectra calculations very well and give accurate results [13].
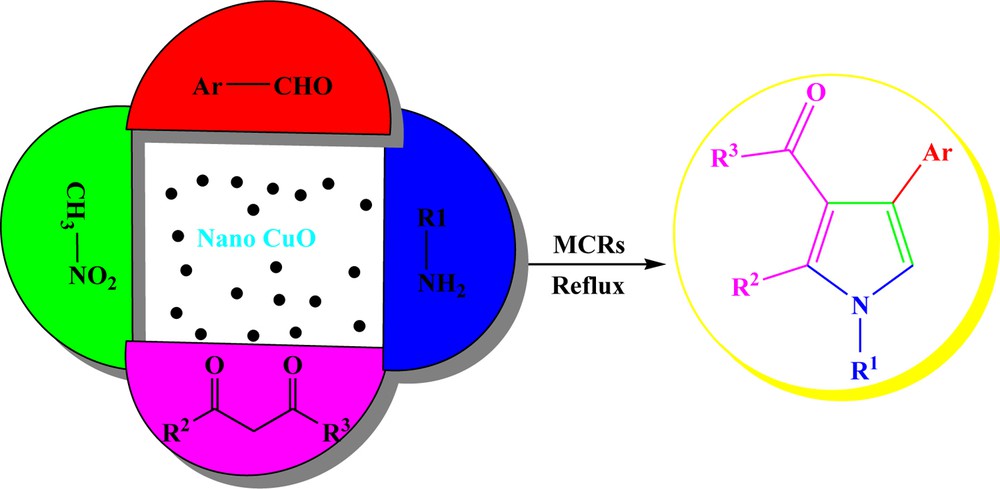
Synthesis route of highly substituted pyrroles via a four-component reaction using nano CuO.
2 Experimental
2.1 General information
All the chemicals required for the synthesis of highly substituted pyrroles were purchased from Sigma-Aldrich (St. Louis, MO, USA), Fluka (Neu-Ulm, Germany) and Merck (Darmstadt, Germany) companies and were used as received. Nano copper oxide, with the mean particle size 40 nm and purity 99.9%, was purchased from Nano Pioneers (Mashhad, Iran) company. The synthesized compounds gave all satisfactory spectroscopic data. A Bruker (DRX-500 Avanes) NMR was used to record the 1H-NMR and 13C-NMR spectra. All NMR spectra were determined in CDCl3 at ambient temperature.
All geometry optimizations and frequency calculations of all species were carried out using the Gaussian 03 program [14]. Density Functional Theory with the Becke three parameters hybrid functional (DFT-B3LYP) calculations were performed with a 6-311++G (2d, 2p) basis set for all atoms. Vibrational frequencies were calculated at the same level to ensure that each stationary point is a real minimum. Harmonic-oscillator approximation was also used for the thermodynamic partition functions. After geometry optimization and frequency calculations, zero-point energies (ZPEs) and thermal corrections are obtained at 298 K. The NMR computations of absolute shieldings were performed using the Gauge-Independent Atomic Orbital (GIAO) method [15].
2.2 General procedure for synthesis of highly substituted pyrroles
To a stirred suspension of CuO nanoparticles (10 mol%) in nitromethane (5 mL) were added an aryl aldehyde (1 mmol), a β-keto ester (1 mmol) and an amine (1.2 mol). The reaction mixture was stirred at 100–105 °C for 12 h. The progress of the reaction was monitored by TLC. After completion of the reaction, the catalyst was recovered by filtration. The nano CuO was reused in subsequent reaction without losing any significant activity. The mixture was concentrated under vacuum. The residue was purified by preparative TLC (eluent:petroleum ether/ethyl acetate 5:1) to afford the desired compound. The structure of the products was confirmed by 1H-NMR, 13C-NMR and comparison with authentic samples prepared by reported methods [16,17]1.
3 Results and discussion
To find the optimal conditions, the synthesis of ethyl 1-benzyl-4-(4-chlorophenyl)-2-methyl-1H-pyrrole-3-carboxylate (Table 1, entry 8) was used as a model reaction. A mixture of 4-chlorobenzaldehyde (1 mmol), methyl acetoacatate (1 mmol), benzyl amine (1.3 mol) and nitromethane (5 mL) was stirred under various reaction conditions at 100 °C. The reaction was checked without nano CuO as a catalyst, and we did not get any product, while good results were obtained in the presence of CuO nanoparticles after 12 h. On using the optimized amount of catalyst, we found that 10 mol% of CuO nanoparticles could effectively catalyze the reaction for the synthesis of the desired product. Commercially available CuO bulk also was evaluated for the synthesis of the desired title compounds. Clearly, the reaction time, using CuO nanoparticles, has been reduced by three times with higher yield than CuO bulk (81% versus 45%). The optimum reaction conditions used was 10% nano CuO and boiling nitromethane as the reaction medium. The 1H-NMR spectrum of ethyl 1-benzyl-4-(4-chlorophenyl)-2-methyl-1H-pyrrole-3-carboxylate (Table 1, entry 8) showed two signals (δ 1.21 and 4.22) for the protons of ethoxy group, a singlet (δ 2.52) for the methyl protons of the pyrrole ring, a resonance for the deshielded benzylic proton (δ 5.09), a singlet (δ 6.62) for the aromatic proton of the pyrrole ring. The presence of this signal in 1H-NMR spectra of synthesized compounds is a good indication of the formation of the desired chemicals. The 1H-decoupled 13C-NMR spectrum of ethyl 1-benzyl-4-(4-chlorophenyl)-2-methyl-1H-pyrrole-3-carboxylate showed 17 distinct resonances in agreement with the proposed structure.
After finding the suitable conditions, we subjected a series of other aromatic aldehydes, primary amines and β-keto ester to obtain the corresponding poly-substituted pyrroles under the optimized reaction conditions. The results are summarized in Table 1. Generally, the reactions were performed well and the reaction rates as well as the yields of products are satisfactory.
The suggested mechanism for this reaction is patterned in Scheme 2. We believe that CuO nanoparticles are coordinated to the oxygen of the aldehyde and activate it for nucleophilic attack [18]. On the other hand, CuO nanoparticles facilitate the enaminezation of the β-keto esters. Subsequently, the enamine I reacts with nitrostyrene II to provide the Michael adduct III. The intermediate III undergoes cyclization with a loss of HNO2 to yield the desired products. The high surface area to volume ratio of CuO nanoparticles is mainly responsible for their catalytic properties [8].
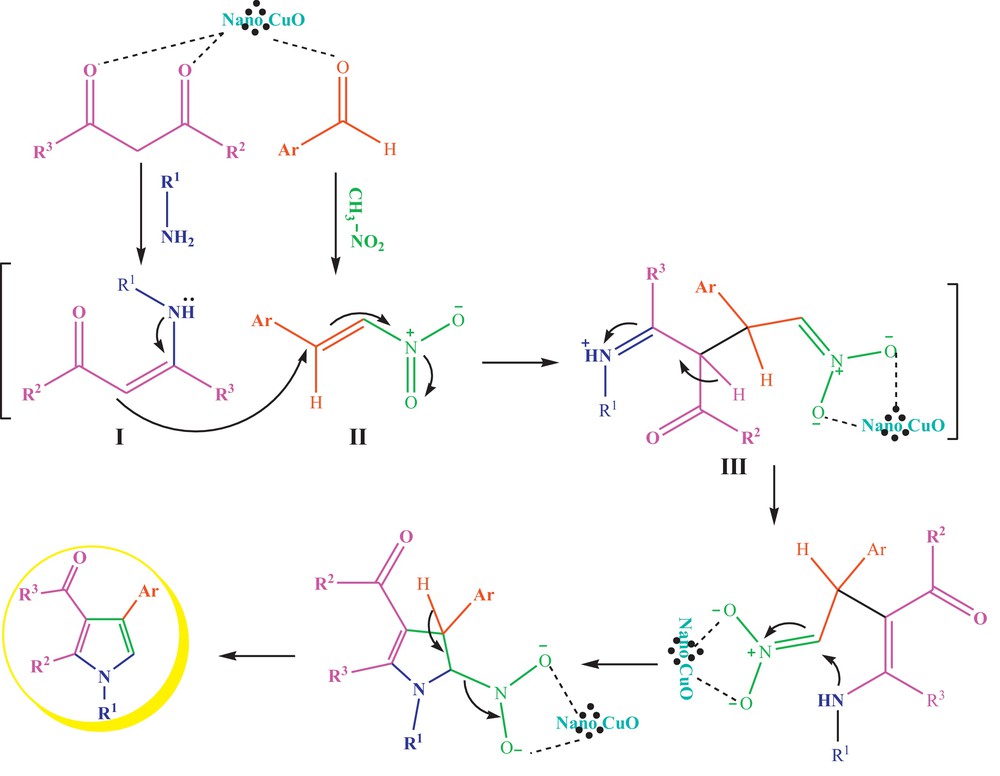
Suggested reaction mechanism for the formation of desired highly substituted pyrroles.
The applicability of the present methodology is further extended by performing the reaction with 4-chlorobenzaldyde, benzoylacetone, benzyl amine and nitromethane in the presence of CuO nanoparticles (Scheme 3).
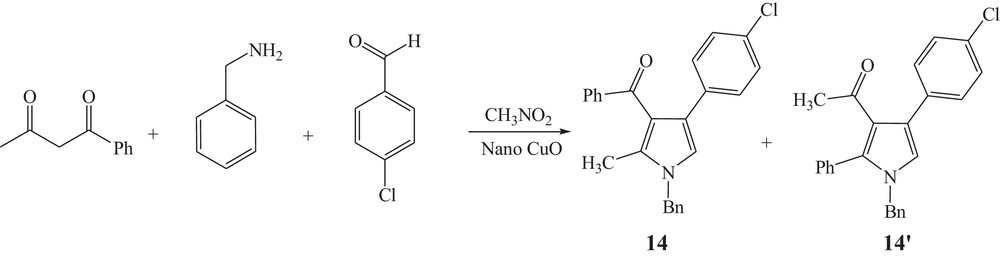
CuO nanoparticles catalyzed reaction of 4-chlorobenzaldyde with benzoylacetone.
This reaction can lead to two structural isomeric products; hence, their NMR spectra are very similar together. In order to provide an assignment of NMR spectra of the studied compounds, the NMR spectra of isomers were coupled with quantum chemical calculations in the DFT approach of hybrid B3LYP exchange-correlation functional to confirm the structures of the synthesized compounds. The optimized structures of 14(14′), are given in Fig. 2 with atom numbers. In order to express the chemical shifts in ppm, the geometry of the TMS molecule had been optimized and then, its NMR spectrum was calculated by using the same method and basis set as in the case of the calculations on the compounds 14(14′).

Numbering optimized structures of 14 (14′).
The calculated isotropic shielding constants were then transformed to chemical shifts relative to TMS. The calculated spectra are compared with the experimental data. Theoretical and experimental chemical shifts of NMR of 14(14′) are given in Table 2.
Experimental and calculated 1H-NMR and 13C-NMR chemical shifts (ppm) for 14(14′).
Atom | 14 | 14′ | ||
δCalcd. | δExp. | δCalcd. | δExp. | |
HCH3 | 1.95 | 2.35 | 1.84 | 2.33 |
HBn | 5.27 | 5.15 | 5.07 | 5.14 |
Hpyrrole | 7.14 | 6.76 | 6.73 | 6.74 |
CCH3 | 11.94 | 11.36 | 31.11 | 11.41 |
CBn | 54.03 | 50.60 | 52.97 | 50.56 |
CCO | 198.81 | 194.16 | 198.15 | 193.95 |
As it can be seen from Table 2, the NMR shifts obtained by the DFT method at the B3LYP/6-311++G (2d,2p) level are in reasonable agreement with the experimental values of the 1H-NMR and 13C-NMR spectra.
4 Conclusions
In conclusion, we have reported an efficient procedure for the synthesis of highly substituted pyrroles using CuO nanoparticles as a non-toxic and inexpensive heterogeneous nanocatalyst. The method also offers some other advantages, such as clean reaction, low loading of catalyst and use of various substrates, which make it a useful and attractive strategy for the synthesis of highly substituted pyrroles. A joint of experimental and DFT calculations was also used to confirm the structure of (1-benzyl-4-(4-chlorophenyl)-2-methyl-1H-pyrrol-3-yl)(phenyl)methanone 14 and 1-(1-benzyl-4-(4-chlorophenyl)-2-phenyl-1H-pyrrol-3-yl)ethanone 14′.
Acknowledgements
We would like to acknowledge the financial support from Payame Noor University (PNU). We also are thankful to Mr. Biglari for NMR spectral support.
1 Representative spectroscopic data: Table 1, Entry 1: 1H-NMR (400 MHz, CDCl3): d 2.51 (s, 3H), 3.71 (s, 3H), 5.10 (s, 2H), 6.63 (s, 1H), 7.11 (d, J = 6.8 Hz, 2H), 7.30–7.43 (m, 8H); 13C-NMR (100 MHz, CDCl3): d 11.56, 50.57 (2 C), 110.76, 120.59, 126.18, 126.21, 126.57, 127.65, 127.84, 128.96, 129.11, 135.80, 136.60, 136.73, 166.33. Table 1, Entry 2: 1H-NMR (400 MHz, CDCl3): d 2.52 (s, 3H), 3.73 (s, 3H), 5.09 (s, 2H), 6.62 (s, 1H), 7.12 (d, J = 8.4 Hz, 2H), 7.32–7.43 (m, 7H); 13C-NMR (100 MHz, CDCl3): d 11.64, 50.61, 50.63, 110.68, 120.67, 125.09, 126.57, 127.78, 127.92, 128.98, 130.47, 132.02, 134.34, 136.61, 136.90, 166.10. Table 1, Entry 5: 1H-NMR (400 MHz, CDCl3): d 2.52 (s, 3H), 3.64 (s, 3H), 5.12 (s, 2H), 6.60 (s, 1H), 7.11 (d, J = 8.4 Hz, 2H), 7.23–740 (m, 7H); 13C-NMR (100 MHz, CDCl3): d 11.39, 50.57, 50.65, 112.13, 120.57, 120.93, 122.91, 126.03, 126.57, 127.64, 127.82, 128.98, 129.11, 131.61, 134.35, 136.01, 136.76, 166.01; Table 1, Entry 7: 1H-NMR (400 MHz, CDCl3): d 1.16 (t, J = 7.2 Hz, 3H), 2.51 (s, 3H), 4.20 (q, J = 7.2 Hz, 2H), 5.10 (s, 2H), 6.63 (s, 1H), 7.11 (d, J = 6.8 Hz, 2H), 7.30–7.44 (m, 8H); 13C-NMR (100 MHz, CDCl3): d 11.51, 14.08, 50.55, 59.41, 111.07, 120.44, 126.14, 126.22, 126.58, 127.53, 127.81, 128.95, 129.30, 135.88, 136.45, 136.78, 165.91. Table 1, Entry 8: 1H-NMR (400 MHz, CDCl3): d 1.21 (t, J = 7.2 Hz, 3H), 2.53 (s, 3H), 4.22 (q, J = 7.2 Hz, 2H), 5.10 (s, 2H), 6.63 (s, 1H), 7.12 (d, J = 7.2 Hz, 2H), 7.32–7.46 (m, 7H); 13C-NMR (100 MHz, CDCl3): d 11.61, 14.22, 50.58, 59.52, 110.96, 120.57, 125.08, 127.56, 127.66, 127.90, 128.97, 129.01, 129.34, 130.65, 131.98, 134.44, 165.68. Table 1, Entry 12: 1H-NMR (400 MHz, CDCl3): d 1.18 (t, J = 7.2 Hz, 3H), 1.86 (d, J = 6.8 Hz, 3H), 2.48 (s, 3H), 4.19 (q, J = 7.2, 2H), 5.40 (q, J = 6.8 Hz, 1H), 6.74 (s, 1H), 7.12 (d, J = 8.4 Hz, 2H), 7.3–7.35 (m, 7H); 13C-NMR (100 MHz, CDCl3): d 11.49, 14.16, 22.18, 55.17, 59.48, 110.83, 116.92, 124.79, 125.83, 127.63, 127.69, 128.92, 130.58, 131.91, 134.69, 136.70, 141.98, 165.81.
Entry | β-Keto esters | Amines | Aldehydes | Products | Yield (%) |
1 | 75 | ||||
2 | 78 | ||||
3 | 69 | ||||
4 | 65 | ||||
5 | 73 | ||||
6 | 76 | ||||
7 | 77 | ||||
8 | 81 | ||||
9 | 70 | ||||
10 | 74 | ||||
11 | 75 | ||||
12 | 68 | ||||
13 | 66 |