[Hydroxy(tosyloxy)iodo]benzene (HTIB, PhI(OH)OTs) is a versatile hypervalent iodine(III) reagent that has numerous applications in organic synthesis [1]. Important applications of HTIB are: α-functionalization of ketones [2] ring expansion [3] ring contraction [4] ring tosyloxylation [5] α-iodination [6] preparation of iodonium salt [7] synthesis of α,β-tosyloxyketones and their conversion into pyrazoles [8] isoxazoles [9] and synthesis of various other organic compounds [10].
The regeneration of the carbonyl functionality is an important step in organic synthesis. The recovery of parent ketones and aldehydes has classically involved acid hydrolysis [11]. However, non-acidic methods are of special significance while dealing with compounds containing acid-sensitive groups [12]. So, considerable interest has been aroused in the development of mild and non-acidic methods for the cleavage of hydrazones, oximes, semicarbazones, thiosemicarbazones, etc. In this regard, a review of protection and deprotection of functional groups in organic synthesis by heterogeneous catalysis has been published by Sartori et al. [13] Though several methods have been employed for the regeneration of the carbonyl functionality, there is scope for the development of a newer and simpler methodology. The common deprotection protocols involve the use of hazardous heavy metal salts, for example mercury(II) chloride [14] and of toxic reagents such as SeO2, (PhSeO)2O, which besides being costly reagents also add to waste-disposal problems [15]. Similarly, reagents such as lead tetraacetate [16] thallium(III) nitrate [17] manganese dioxide [18] Y [19] and ZSM-5 [20] chlorochromate [21] ammonium chlorochromate adsorbed on alumina [22] iodic acid [23] N-bromo-N-benzoyl-4-toluenesulfonamide [24] vanadyl acetylacetonate [25] aqueous phosphoric acid [26] and clayfen [25] have also been utilized for the regeneration of carbonyl compounds. However, some of these methods have suffered from different drawbacks such as requirements for refluxing temperature, tedious work-up, drastic conditions, long reaction times, undesired chemical yields, and use of toxic reagents. Recently, microwave irradiation has also been developed, which is valuable from the synthetic standpoint. But extreme precautions have to be taken as these reactions are performed under microwave irradiation or ultrasonic irradiation with an oxidant [27].
Moriarty et al. [28] developed an hypervalent iodine(III)-mediated methodology for the regeneration of various carbonyl compounds from oximes using iodobenzene diacetate (IBD). Further, Barton et al. [29] proposed a method involving the iodine(III)-mediated oxidation of various hydrazone derivatives of keto esters using HTIB, IBD, and [bis(trifluoroacetoxy)iodo]benzene (BTIB), which has been reported for the regeneration of parent carbonyl compounds. Parent ketones were also regenerated from semicarbazones using IBD [30].
In connection with our ongoing programme directed towards the use of hypervalent iodine(III) compounds as unique reagents in organic synthesis, we have recently reported the simple and efficient iodine(III)-mediated cleavage of carbonyl derivatives of dehydroacetic acid (DHA) with HTIB and IBD [31]. Various derivatives of carbonyls, such as aryl/heteroaryl hydrazones, oximes, semicarbazones and thiosemicarbazones, gave the parent carbonyl back after reaction with either HTIB or IBD. Both reagents showed a similar behaviour in the cleavage of derivatives of carbonyl compounds. Encouraged by these observations, we further extended our research to study the behaviour of these two reagents towards carbonyl derivatives of other heterocyclic moieties and obtained some interesting results.
First, we carried out the oxidation of pyridylhydrazones of 4-formylpyrazoles (1a–g) with HTIB in dichloromethane (DCM) and we observed that oxidative cleavage1 occurred smoothly in this case, giving back the parent carbonyl compounds (2a–g)2. However, in our previous investigation, we had found that the same substrates when oxidised with IBD gave 1,2,4-triazole derivatives (i.e., oxidative cyclisation occurred) (Scheme 1 and Table 1) [32]. Similar results were obtained with other aromatic aldehydes (Scheme 2 and Table 2) [33].

Oxidative cleavage of carbonyl derivatives (1a-g) with HTIB.
Sr. no. | Reactant | Reference | Product | Time (in min) | Melting point (°C) | Yield (%) |
1 | 1a | 35 | 2a | 30 | 140 | 80 |
2 | 1b | 35 | 2b | 30 | 122 | 82 |
3 | 1c | 35 | 2c | 30 | 121 | 83 |
4 | 1d | 35 | 2d | 30 | 148 | 78 |
5 | 1e | 35 | 2e | 30 | 110 | 77 |
6 | 1f | 35 | 2f | 30 | 132 | 82 |
7 | 1g | 35 | 2g | 30 | 165 | 85 |
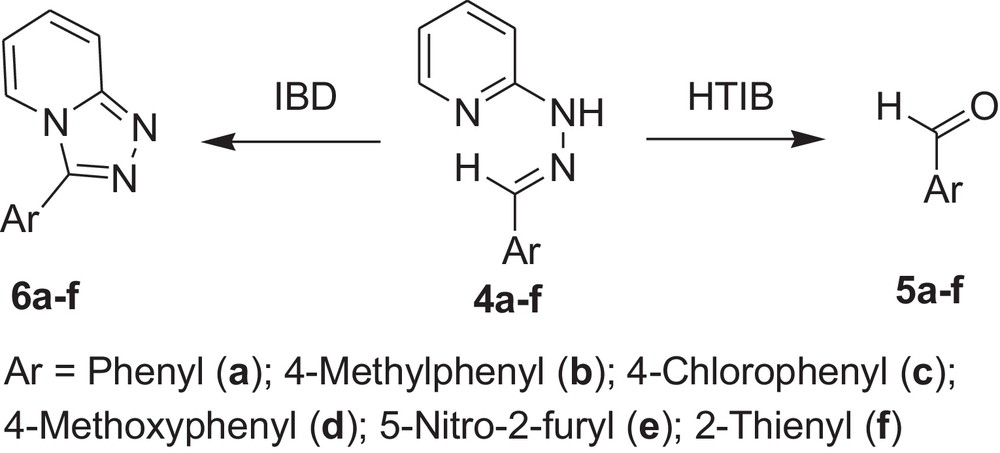
Oxidative cleavage of carbonyl derivatives (4a-f) with HTIB.
Sr. no. | Reactant | Reference | Product | Time (in min) | Melting point (°C) | Yield (%) |
1 | 4a | 36 | 5a | 60 | –2.6/179a | 76 |
2 | 4b | 36 | 5b | 60 | –6/204–205a | 78 |
3 | 4c | 36 | 5c | 60 | 46/60a | 75 |
4 | 4d | 36 | 5d | 60 | 0/248a | 77 |
5 | 4e | 36 | 5e | 60 | 37–39 | 70 |
6 | 4f | 36 | 5f | 60 | 198a | 69 |
a Boiling point in °C.
Encouraged by these observations, we carried out reactions with other hydrazones containing heterocyclic moieties, like quinoline [33] pyrimidine [34] phthalazine [35], etc. Interestingly, similar oxidative cleavage occurred in all cases with HTIB. It is to be mentioned that in our previous investigation oxidative cyclisation occurred with IBD in such cases (Schemes 3–7 and Tables 3–7).
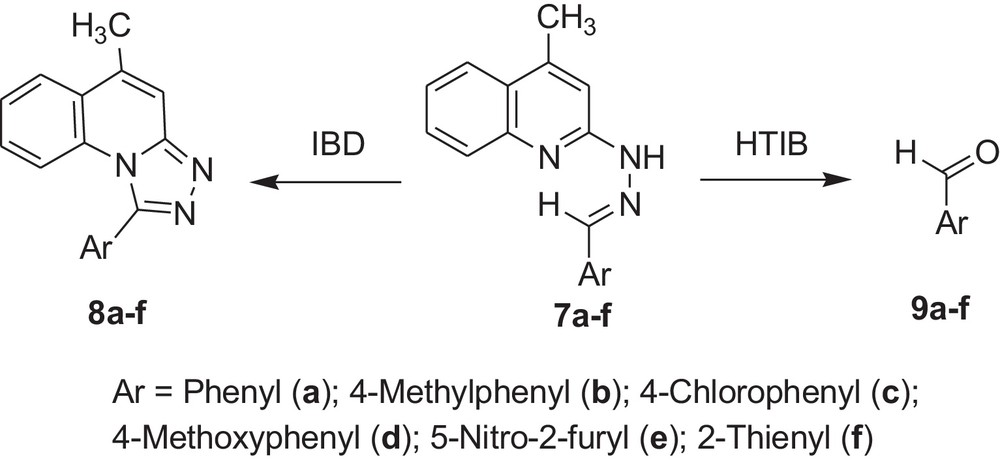
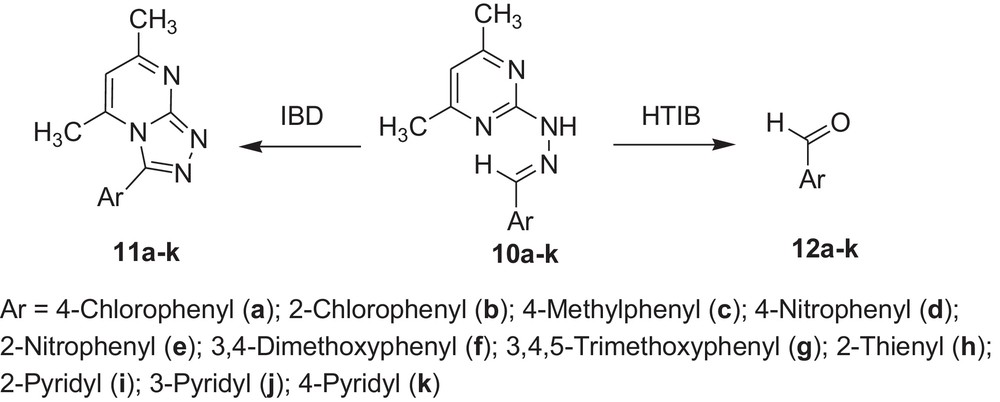



Oxidative cleavage of carbonyl derivatives (7a–f) with HTIB.
Sr. no. | Reactant | Reference | Product | Time (in min) | Melting point (°C) | Yield (%) |
1 | 7a | 37 | 9a | 60 | –2.6/179a | 74 |
2 | 7b | 37 | 9b | 60 | –6/204–205a | 76 |
3 | 7c | 37 | 9c | 60 | 46 | 75 |
4 | 7d | 37 | 9d | 60 | 0/248a | 77 |
5 | 7e | 37 | 9e | 60 | 37–39 | 68 |
6 | 7f | 37 | 9f | 60 | <10/198a | 69 |
a Boiling point in °C.
Oxidative cleavage of carbonyl derivatives (10a–k) with HTIB.
Sr. no. | Reactant | Reference | Product | Time (in min) | Melting point (°C) | Yield (%) |
1 | 10a | 38 | 12a | 60 | 46 | 70 |
2 | 10b | 38 | 12b | 60 | 9–11/209–215a | 72 |
3 | 10c | 38 | 12c | 60 | –6/204–205a | 74 |
4 | 10d | 38 | 12d | 60 | 103–106 | 76 |
5 | 10e | 38 | 12e | 60 | 40–43 | 77 |
6 | 10f | 38 | 12f | 60 | 72–74 | 75 |
7 | 10g | 38 | 12g | 60 | 72–75 | 77 |
8 | 10h | 38 | 12h | 60 | <10/198a | 71 |
9 | 10i | 38 | 12i | 60 | –21/181a | 62 |
10 | 10j | 38 | 12j | 60 | 8/78–81a | 66 |
11 | 10k | 38 | 12k | 60 | 71–73a | 63 |
a Boiling point in °C.
Oxidative cleavage of carbonyl derivatives (13a–k) with HTIB.
Sr. no. | Reactant | Reference | Product | Time (in min) | Melting point (°C) | Yield (%) |
1 | 13a | 38 | 15a | 60 | –10/181a | 72 |
2 | 13b | 38 | 15b | 60 | 46 | 71 |
3 | 13c | 38 | 15c | 60 | 55–58 | 73 |
4 | 13d | 38 | 15d | 60 | –26/179a | 74 |
5 | 13e | 38 | 15e | 60 | –6/204–205a | 74 |
6 | 13f | 38 | 15f | 60 | 0/248a | 76 |
7 | 13g | 38 | 15g | 60 | 103–106 | 77 |
8 | 13h | 38 | 15h | 60 | <10/198a | 69 |
9 | 13i | 38 | 15i | 60 | 8/78–81a | 50 |
10 | 13j | 38 | 15j | 60 | 64–66 | 77 |
11 | 13k | 38 | 15k | 60 | 46–48 | 78 |
a Boiling point in °C.
Oxidative cleavage of carbonyl derivatives (16a–i) with HTIB.
Sr. no. | Reactant | Reference | Product | Time (in min) | Melting point (°C) | Yield (%) |
1 | 16a | 38 | 18a | 60 | –6/204–205a | 73 |
2 | 16b | 38 | 18b | 60 | 0/248e | 75 |
3 | 16c | 38 | 18c | 60 | 46–48 | 76 |
4 | 16d | 38 | 18d | 60 | 46–47 | 75 |
5 | 16e | 38 | 18e | 60 | 55–58 | 76 |
6 | 16f | 38 | 18f | 60 | –10/181a | 72 |
7 | 16g | 38 | 18g | 60 | 103–106 | 76 |
8 | 16h | 38 | 18h | 60 | –36/54–56a | 70 |
9 | 16i | 38 | 18i | 60 | 37–39 | 68 |
a Boiling point in °C.
Oxidative cleavage of carbonyl derivatives (19a–l) with HTIB.
Sr. no. | Reactant | Reference | Product | Time (in min) | Melting point (°C) | Yield (%) |
1 | 19a | 39 | 21a | 60 | –26/179a | 73 |
2 | 19b | 39 | 21b | 60 | –6/204–205a | 74 |
3 | 19c | 39 | 21c | 60 | 46 | 75 |
4 | 19d | 39 | 21d | 60 | 55–58 | 74 |
5 | 19e | 39 | 21e | 60 | 0/248a | 75 |
6 | 19f | 39 | 21f | 60 | –10/181a | 72 |
7 | 19g | 39 | 21g | 60 | <10/198a | 68 |
8 | 19h | 39 | 21h | 60 | 103–106 | 76 |
9 | 19i | 39 | 21i | 60 | 34–37 | 72 |
10 | 19j | 39 | 21j | 60 | 64–69 | 74 |
11 | 19k | 39 | 21k | 60 | 9–11/209–211 | 73 |
12 | 19l | 39 | 21l | 60 | 9–12/213–14 | 74 |
a Boiling point in °C.
We also carried out the reaction with pyrazolylaldehyde N-acylhydrazones (22a-g) with HTIB and it was found that in these cases also oxidative cleavage occurred with HTIB, while our previous investigation showed that oxidative cyclisation occurred with IBD (Scheme 8 and Table 8) [36].
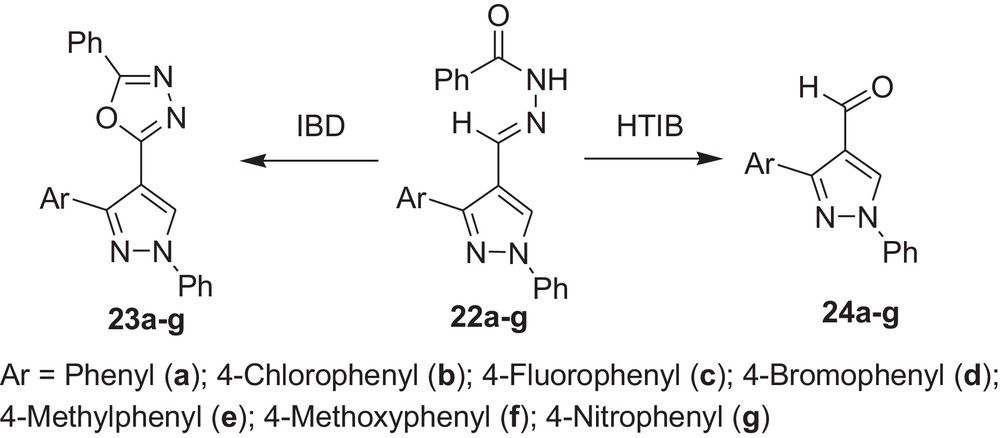
Oxidative cleavage of carbonyl derivatives (22a–g) with HTIB.
Sr. no. | Reactant | Reference | Product | Time (in min) | Melting point (°C) | Yield (%) |
1 | 22a | 40 | 24a | 30 | 140 | 78 |
2 | 22b | 40 | 24b | 30 | 110 | 80 |
3 | 22c | 40 | 24c | 30 | 148 | 77 |
4 | 22d | 40 | 24d | 30 | 132 | 83 |
5 | 22e | 40 | 24e | 30 | 122 | 79 |
6 | 22f | 40 | 24f | 30 | 121 | 80 |
7 | 22g | 40 | 24g | 30 | 165 | 81 |
As expected, in the case of semicarbazones (25a–f), thiosemicarbazones (26a–f) of 4-formylpyrazoles, we observed the oxidative cleavage with both reagents i.e., HTIB and IBD. But with IBD in addition to oxidative cleavage, the formation of several products (as evident by TLC) was observed (Scheme 9 and Table 9). Similarly, in case of phenylhydrazones of 4-formylpyrazoles, oxidative cleavage occurred with both HTIB and IBD (Scheme 10 and Table 10) [37].

Oxidative cleavage of carbonyl derivatives (25a–f and 26a–f) with HTIB.
Sr. no. | Reactant | Reference | Product | Time (in min) | Melting point (°C) | Yield (%) |
1 | 25a | 41 | 27a | 30 | 140 | 82 |
2 | 25b | 41 | 27b | 30 | 121 | 81 |
3 | 25c | 41 | 27c | 30 | 122 | 83 |
4 | 25d | 41 | 27d | 30 | 110 | 84 |
5 | 25e | 41 | 27e | 30 | 132 | 80 |
6 | 25f | 41 | 27f | 30 | 148 | 85 |
7 | 26a | 41 | 27a | 30 | 140 | 82 |
8 | 25b | 41 | 27b | 30 | 121 | 86 |
9 | 26c | 41 | 27c | 30 | 122 | 87 |
10 | 26d | 41 | 27d | 30 | 110 | 86 |
11 | 26e | 41 | 27e | 30 | 132 | 82 |
12 | 26f | 41 | 27f | 30 | 148 | 84 |

Oxidative cleavage of carbonyl derivatives (28a–f) with HTIB.
Sr. no. | Reactant | Reference | Product | Time (in min) | Melting point (°C) | Yield (%) |
1 | 28a | 41 | 27a | 30 | 140 | 82 |
2 | 28b | 41 | 27b | 30 | 121 | 84 |
3 | 28c | 41 | 27c | 30 | 122 | 83 |
4 | 28d | 41 | 27d | 30 | 110 | 85 |
5 | 28e | 41 | 27e | 30 | 132 | 86 |
6 | 28f | 41 | 27f | 30 | 148 | 84 |
Although mechanisms offering such oxidative cyclisations and oxidative cleavage with IBD and HTIB have been described in previous reports [38], there is still a need for further work to explain the different reactivity pattern of IBD and HTIB, especially the uniqueness of HTIB for CN cleavage. Therefore, on the basis of the products formed and consumption of reagent, a plausible mechanism is shown in Schemes 11–12. The first step involves an electrophilic attack of HTIB on the NH group of hydrazone with loss of p-toluenesulphonic acid, giving an unstable iodine(III) species (29d and 32c), which subsequently undergoes rearrangement, in which the –OH group attached to iodine attacks intra-molecularly to form an α-hydroxyazo intermediate (29e and 32d) and iodobenzene (it is this –OH group of HTIB (PhI(OH)OTs) that is responsible for cleavage). Another molecule of HTIB attacks the azo group to initiate the decomposition of the α-hydroxyazo intermediate (29f and 32e), giving back carbonyl compounds (31 and 34) with formation of iodobenzene, water, and salt.
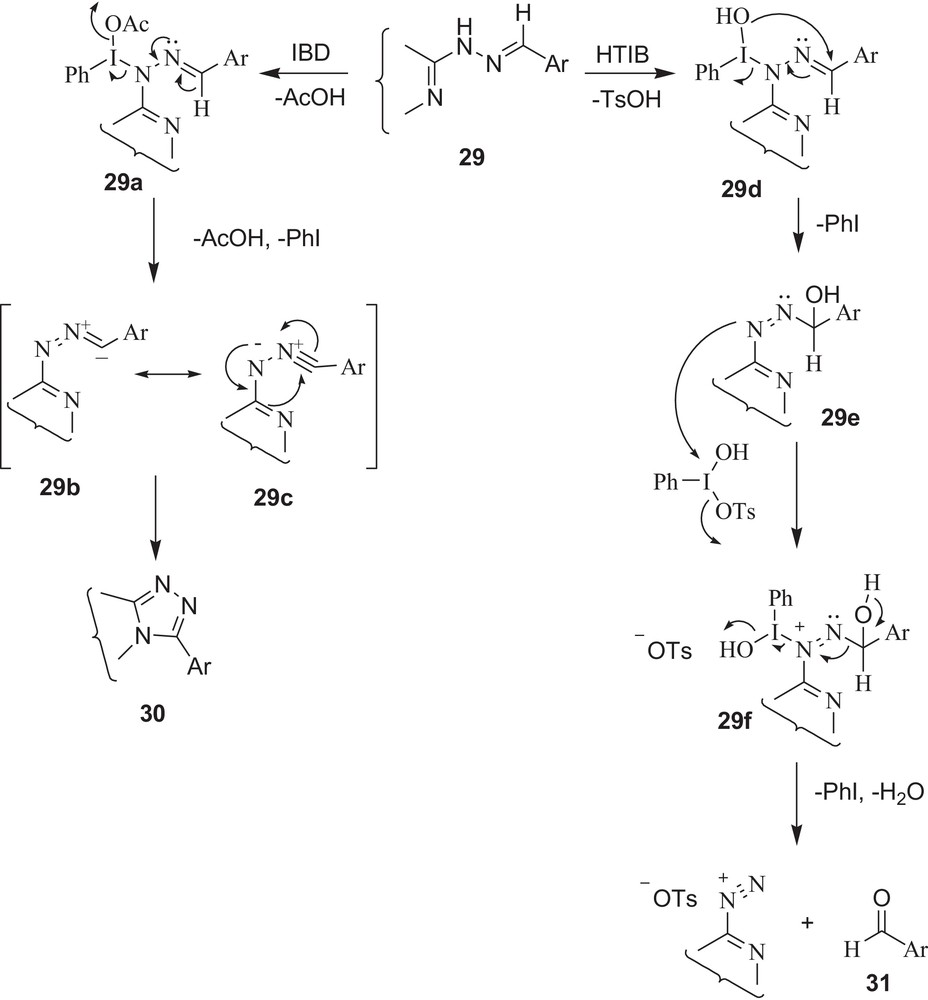

In summary, the present study offers a significant application of HTIB in an efficient and convenient regeneration of carbonyl compounds from derivatives of carbonyl having N-containing heterocyclic systems that are known to undergo oxidative cyclisations with IBD. Semicarbazones, thiosemicarbazones and phenylhydrazones of formyl pyrazoles and other simple carbonyl compounds that do not have adjacent nitrogen atoms give carbonyl compounds back with both reagents i.e., HTIB and IBD1 [30–31]. Furthermore, the reagent HTIB is significant for the following reasons:
- • it tolerates a variety of substrates;
- • overoxidation of aldehydes to their carboxylic acids do not take place;
- • the oxidative approach is ecofriendly in nature;
- • the method only needs a very simple set-up and mild conditions.
1 Initially the reaction of hydrazone was attempted with 1.1 equiv of HTIB in dichloromethane at room temperature. The following observations were made: (a) HTIB started dissolving; (b) the colour of the reaction medium changed from yellow to reddish brown; (c) a characteristic smell of iodobenzene was observed after evaporating the solvent from the reaction mixture. All these changes indicated the occurrence of the reaction, which was supported by the monitoring the TLC of the reaction mixture. The reaction was completed in 4 h. The product obtained was found to be the parent 4-formyl pyrazole (by comparison of TLC, melting point and NMR and IR data with authentic sample) in 50% yield. To optimize the results of oxidative cleavage, the reaction of hydrazone was attempted by increasing the molar ratio of the reagent, i.e. with 2.2 equiv of HTIB in DCM at room temperature. The colour of the reaction mixture changed immediately from yellow to brown black. Usual work-up of the reaction afforded the starting carbonyl compound in 80% yield. Thus, it was found that increasing the molar ratio of HTIB not only increases the yield, but also improves the neatness of the reaction. Encouraged by these successful results, we studied the scope of the HTIB mediated oxidative cleavage with other derivatives. The effect of the solvent was also tested by using different solvents, i.e. methanol, ethanol, and acetonitrile. The reaction proceeded with equal efficacy in acetonitrile, but with poor yield in ethanol and methanol. Therefore, acetonitrile and DCM are found to be suitable solvents for the oxidative cleavage of carbonyl derivatives of various aromatic aldehydes. As expected, this procedure involving HTIB was also successful for effective cleavage of semicarbazones and thiosemicarbazones derived from simple ketones such as cyclohexanone and acetophenone etc.
2 General experimental conditions: HTIB was prepared from IBD and p-toluenesulphonic acid monohydrate in acetonitrile. IBD and all other chemicals used were purchased from commercial sources and were used without further purification. 1H NMR was recorded on a Bruker 300 MHz instrument using tetramethyl silane (TMS) as an internal standard. IR spectra were recorded with a PerkinElmer 1800 FT-IR spectrophotometer. General cleavage procedure: To a stirred suspension of hydrazone (0.000589 mol) in DCM (15 mL) was added HTIB (0.00129 mol) in portion in 10 min at room temperature in open air atmosphere. The colour of the reaction mixture changed from red/yellow to brown. The progress of the reaction mixture was monitored by TLC. Stirring was continued for 10–60 min. After completion of the reaction, the solvent was distilled off and the resulting residue was triturated with petroleum ether (boiling range 60–80 °C) to remove the iodobenzene. The product obtained was purified by recrystallization and column chromatography using petroleum ether and ethyl acetate as eluents in 60–80% yield.