Abridged version
1 Introduction
In intraplate areas, landforms are strongly modified by erosion and particularly by cryoclastic processes that operated periodically during Quaternary time. In these zones, as exemplified by NW France, intraplate deformation is characterised by slow uplift rates [10]. However, the various responses of landforms to regional deformation are weakened by the combination of both efficient erosion and moderate uplift rates.
The analysis of fault scarp degradation is presented here. It concerns the Jobourg fault scarp that forms the southern boundary of north Cotentin (Fig. 1). The computation of the volume of rock eroded from the scarp is constrained by fitting (i) digital elevation model data and (ii) field data relative to head and slope deposits.
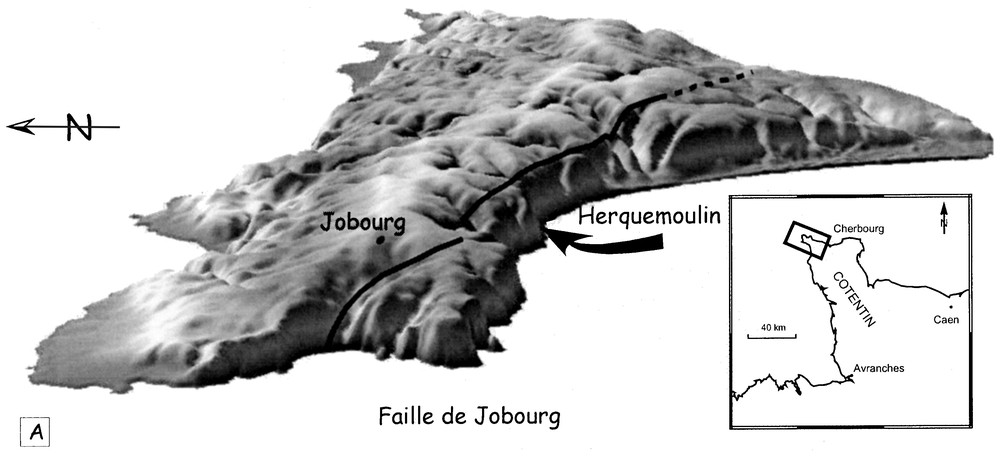

A. Topographie numérisée de la presqu'ı̂le du Nord Cotentin, montrant l'escarpement de la faille de Jobourg. B. Localisation et épaisseur des produits de l'érosion (head). Zone d'alimentation (trait épais), bassin versant d'Herquemoulin (1) et facettes triangulaires (2).
A. Digital Elevation Model from North Cotentin showing the Jobourg fault-scarp. B. Location and thickness of head and its supply range (thick line), Herquemoulin watershed (1), triangular facets (2).
2 Climatic controls on landforms development
Quaternary geomorphic systems in intraplate zones are strongly influenced by the conjugate effects of tectonic and climatic processes. Considering a time interval greater than 105 years, morphodynamics seem to be highly controlled by the tectonic differential uplift. Conversely considering a time interval lower than 105 years morphodynamics and hill slopes are strongly influenced by the landform degradation linked to the climatic input.
2.1 Indicators of the climatic degradation of a fault scarp
In Normandy, where Plio-Quaternary deformation induces landform rejuvenation and river entrenchment [1,11,13], fault scarps are particularly concerned by the climatic degradation. Weathering helps erosion and denudation of the scarp while cumulative effects of vertical uplift and gravitational collapse of the scarp-free face lead to complex shapes, characterised by an upper concave and a lower convex segment.
Evidences of scarp erosion and decay can be found along the Jobourg Fault Zone (Fig. 1). In this area, landforms are strongly influenced by climate-related processes, such as cryoclastic processes that occurred during periglacial periods [15]. These processes have contributed to the cracking of rocks near the surface, then sliding of cryoclasts during thawing periods gives rise to head and slope deposits several tens of metres thick [5,20]. The head is roughly stratified and made of coarse angular metamorphic and sedimentary rocks that come from the Palaeozoic units slope above. The head lies on a raised beach and shows geometrical and lithological characteristics (Fig. 2): (i) horizontal variations of the lithology follows the horizontal variations of the bedrock on the backslope, (ii) the vertical stratification is characterised by an evolution from a very coarse lower head with angular blocks close to 1 m embedded in a sandy clay matrix to a upper head, less coarse and with a loessic matrix, (iii) the bedding dips seaward, (iv) the preferred stone orientation is subparallel to the slope. These characteristics of the head have been considered as evidences in favour of a periglacial origin [5,8,13,20].
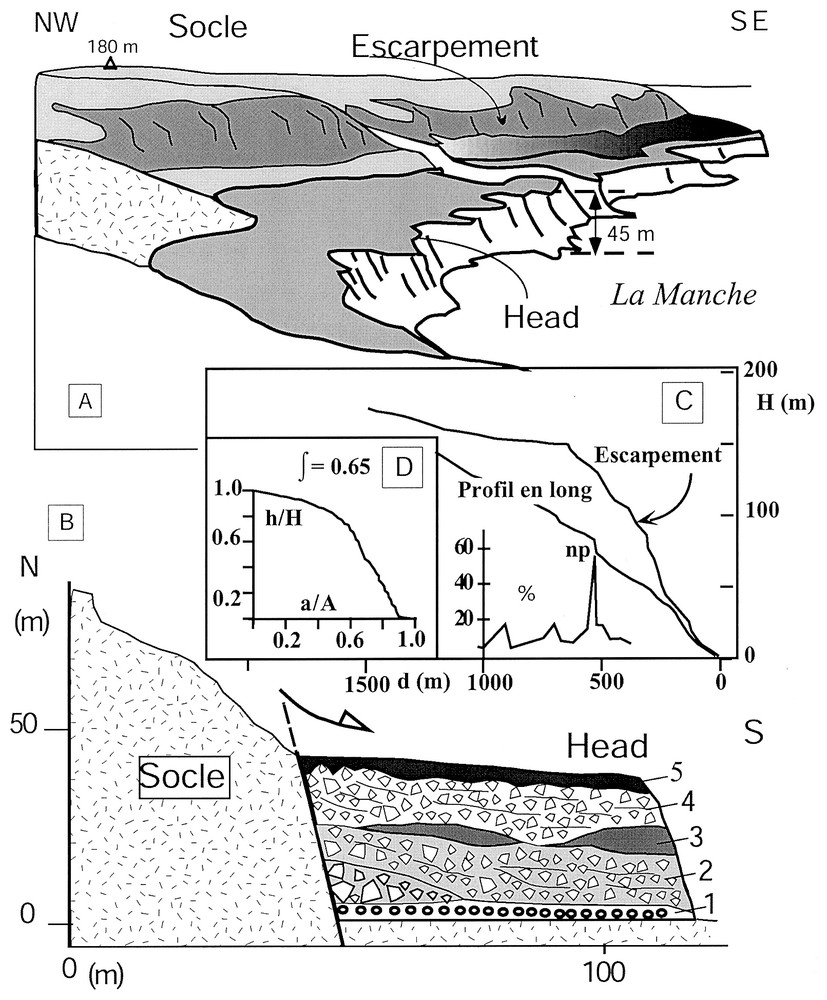
A. Vue de la baie d'Herquemoulin, montrant le prisme de head formant une terrasse au pied de l'escarpement de Jobourg. B. Stratification verticale du head, montrant la succession: 1, paléoplage, 2, head inférieur très grossier, 3, niveau de lœss, 4, head supérieur plus fin à matrice lœ ssique, 5, lœss et colluvions. C. Escarpement de la faille de Jobourg, profil en long du cours d'eau et distribution des pentes (%) montrant une rupture de pente np (nickpoint). D. Courbe hypsométrique, exprimant l'aire des différentes tranches d'altitude du bassin versant et intégrale hypsométrique ∫ exprimant le volume relatif non érodé. Masquer
A. Vue de la baie d'Herquemoulin, montrant le prisme de head formant une terrasse au pied de l'escarpement de Jobourg. B. Stratification verticale du head, montrant la succession: 1, paléoplage, 2, head inférieur très grossier, 3, niveau de ... Lire la suite
A. Herquemoulin beach, characterised by a wide prism of head that makes a terrace at the bottom of the scarp. B. Vertical stratification of the head: 1, raised beach, 2, very coarse lower head, 3, loesses, 4, upper head with a loessic matrix, 5, loess and colluviums. C. Fault scarp profile, longitudinal profile of the river and slope distribution (%) and nickpoint (np). D. Hypsometric curve and integral ∫.
2.2 Fault scarp degradation processes
Detailed analyses of the morphological and sedimentary properties of the slope deposits along the Jobourg fault have allowed accurate identification of periglacial processes [5,13]. The head of Jobourg is attributed to solifluction processes [17]. In these processes, downward displacement of the cryoclastic material occurs mainly by frost creep and gelifluction. It implies specific climatic conditions with a high number of freeze-thaw cycles and seasonal alternation with (i) cold and dry seasons responsible for slow frost creep and (ii) more humid seasons where gelifluction predominates [8]. Other processes may interfere to accumulate the observed deposits. Very coarse lower deposits, near cliff foot, suggest a moderate rockfall activity. Local flows of more or less cohesive debris can be inferred from the alternation of fine material concentrated at the base of the flow and imbricated coarse gravels and blocks concentrated both at the top and at the front of the flow.
3 Quantification of fault scarp degradation
The computation of the volume of rock eroded from the scarp is constrained by fitting (i) digital elevation model data and (ii) field data relative to head and slope deposits.
Jobourg fault scarp morphologies have been investigated using digital elevation model data, which allow a precise description of the profile of the slope. Several profiles have been extracted (Fig. 3). For each profile, we compute the vertical offset, the slope distribution, the maximum scarp slope and the thickness of slope deposits accumulated along the scarp.
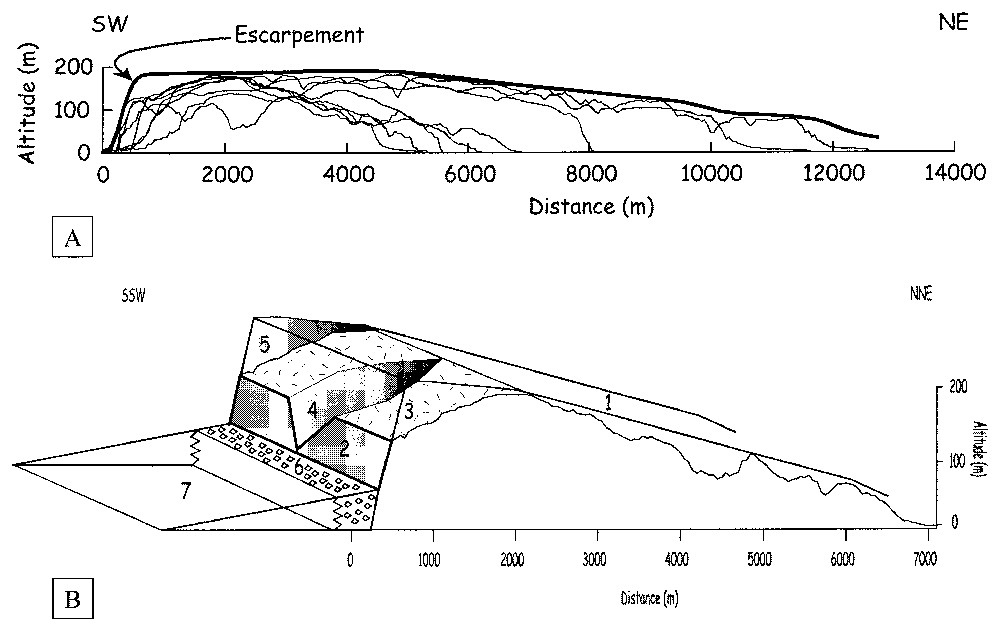
A. Profils sériés de la presqu'ı̂le du Nord Cotentin (traits fins), illustrant la forte asymétrie du relief ; surface enveloppe comportant le coin sud érodé. B. Principaux volumes et surfaces quantifiés: flanc nord (1), escarpement de la faille de Jobourg (2), zone d'alimentation des heads (3), incision de la vallée d'Herquemoulin (4), coin manquant (5), dépôt de pente (6) et prisme de head (7).
A. Topographic profiles of North Cotentin (thin lines) showing landscape asymmetry. Surface envelope with missing corner. B. Main quantified volumes and surfaces: north side (1), Jobourg fault-scarp (2), head feeding zone (3), Herquemoulin erosion (4), missing corner (5), slope deposit (6) and head prism (7).
Scarp measurements yield height values that range from a few tens of metres to over 100 m (Fig. 1). Slope profiles are characterised by a steep central zone bounded by an upper concave and a lower convex segment. This latter segment corresponds to head and slope deposits that form a terrace sloping moderately seaward. The head width ranges between 30 and 40 m, whereas the surface slope of the head above the cliff edge is between 5° and 10°. The height of the backslope reaches 180 m and the altitude of the maximum scarp slope is around 85 m (Fig. 1B).
The quantification of the scarp erosion is based on the following methodology (Fig. 3).
(1) Taking elevations from the ridgelines and interpolating a surface from these points [4] give us the envelope surface of the topography (Fig. 3A). This computed envelope surface is expected to be close of the Late Tertiary erosional surface [7]. The envelope surface shows (i) an asymmetry and a northward tilting of the area consistent with differential uplift along the Jobourg fault [11], (ii) a significant cutting of the top of the slope that modifies strongly the scarp profile.
(2) The difference between the topography and the envelope surface corresponds to the volume of erosion (Fig. 3). Computation of this volume takes into account (i) the volume of the missing corner near the top of the slope, (ii) the volume corresponding to the incision of the hydrographic network (Fig. 3B). The computed volume of erosion (0.22 km3) is then changed to volume of slope deposits (0.28 km3) according to the change of the volumic mass (2.4 to 1.9 g cm−3).
(3) Slope deposits form a sedimentary prism dipping moderately seaward (Fig. 3B). Mapping these deposits gives us an assessment of the volume of rock eroded from the scarp and stored downslope. The surface slope of the head has been scanned to obtain a network of points used to compute (i) the three-dimensional shape of the prism and its volume, (ii) the net volume of the head actually observed. The results indicate that the volume of the head is near 0.026 km3. The volume of corresponding eroded rocks is near 0.03 km3, i.e. 4.6 m3 m−2. Finally, the quantification of the scarp degradation indicates a bulk erosion of 0.22 km3, which corresponds to 39 m3 m−2, while the volume of head accumulated and stored at the bottom of the fault scarp only represents 4.6 m3 m−2, i.e. 1/8 of the bulk scarp erosion.
(4) Computation of the erosion rate during a climatic cycle of the Quaternary implies a precise knowledge of the number of climatic cycles registered within the head of Jobourg. Although it was previously hypothesized that at least two climatic cycles were registered in the head of the Cotentin [13], the lack of marked stratigraphic discontinuities and the faint resistance of the head to marine erosion argue for one slope deposit Weichselian in age.
This assumption leads to an estimation of the erosion rate of the scarp near 4.6 m3 m−2 during a climatic cycle. According to the bulk scarp erosion that reaches 39 m3 m−2, the value of 4.6 m3 m−2 implies eight climatic cycles and therefore gives an assessment of the age of the scarp fault near one million years. These values are consistent with data obtained from measurements in actual periglacial deposits [6].
4 Conclusion
In Normandy, fault scarps are particularly relevant to the climatic degradation that operated periodically through the Quaternary period [5,7,13,18]. The degradation of the Jobourg fault scarp (north Cotentin) leads to head and slope deposits that form a sedimentary prism. Morphological and sedimentary properties of slope deposits suggest frost creep and gelifluction processes under periglacial conditions.
The quantification of the scarp degradation, using digital elevation model data, indicates a bulk erosion of 0.22 km3, which corresponds to 39 m3 m−2, while the volume of head accumulated and stored at the bottom of the fault scarp only represents 4.6 m3 m−2, i.e. 1/8 of the bulk scarp erosion. Field data suggest that the head of Jobourg can be ascribed to the Weichselian cycle. Following this assumption, the computed erosion implies that the scarp degradation started about one million years ago.
1 Introduction
L'Ouest de la France constitue un exemple de domaine intraplaque qui a été soumis, au Quaternaire, depuis au moins 1 Ma, à de profondes modifications climatiques, avec apparition de manière périodique de conditions périglaciaires [13,18]. Une étude de la dégradation des formes du relief sous l'effet du climat y a été entreprise dans le cadre du Programme national des risques naturels.
L'idée qui sous-tend cette étude est que, au cours d'un cycle climatique du Quaternaire, les principaux changements de forme des versants, y compris des escarpements de faille, sont liés à des processus d'érosion cryoclastique en contexte périglaciaire humide. Ces processus génèrent d'importants volumes de formations de surface (dépôts de pente). Leur mise en place, principalement par cryoreptation, gélifluxion et coulées de débris se produirait sur un pas de temps relativement court et suppose des vitesses d'érosion momentanément élevées [15]. Ces processus, qui contribuent à la dégradation des formes du relief, altèrent également la signature morphologique des déformations récentes, surtout en domaine intraplaque actif, à déformation supposée lente, de l'ordre de 0.1 mm an−1 [10–12].
Le problème est abordé ici sur un escarpement attribué à la faille de Jobourg, qui constitue la limite sud de la presqu'ı̂le du Nord Cotentin (Normandie) [11]. Une méthode de quantification de la dégradation de l'escarpement est présentée. L'utilisation d'un modèle numérique de terrain a permis de calculer le volume total de roche enlevé à l'escarpement et le volume des produits de l'érosion encore stockés au pied de la faille. Une estimation du taux d'érosion de l'escarpement au cours d'un cycle climatique du Quaternaire est ensuite présentée.
2 Effets des conditions climatiques périglaciaires sur les formes du relief
En domaine intraplaque, sur un pas de temps court, inférieur ou égal à 105 ans, les reliefs issus de la déformation de la lithosphère peuvent subir de profondes transformations sous l'effet des changements climatiques haute fréquence du Quaternaire. Les facteurs climatiques affectent les processus d'érosion des versants. Ils conditionnent l'altération, c'est-à-dire la capacité des roches à produire des matières transportables. Ils conditionnent la cohésion interne et la résistance à l'érosion des assemblages granulaires [9]. Les facteurs climatiques contrôlent également la capacité de transport, en intervenant sur la quantité d'eau disponible à la surface et sur son mode de drainage, qui varie fortement entre les périodes froides et les périodes plus tempérées de plus basse énergie.
2.1 Les marqueurs de la dégradation d'un escarpement de faille sous l'effet du climat
En Normandie, la faille de Jobourg forme la limite sud de la presqu'ı̂le du Nord Cotentin (Fig. 1A). Elle appartient à la zone de failles varisques N110 « Les Casquets–Aurigny–Jobourg ». Cette faille présente localement des escarpements de plusieurs dizaines de mètres de haut, avec des pentes moyennes de l'ordre de 25 à 30° vers le haut, mais pouvant atteindre 70° en bas de versant, où ils forment des falaises (Fig. 2). Un rejeu de cette faille au Plio-Quaternaire a été envisagé sur la base d'arguments géomorphométriques et sismotectoniques [1,11,13]. Ce rejeu est suggéré (i) par les valeurs élevées des intégrales hypsométriques, indiquant des reliefs immatures et rajeunis (Fig. 2D), (ii) par la forte incision et les ruptures de pente (nickpoints) des cours d'eau, indiquant des instabilités morphologiques liées à des mouvements verticaux différentiels, avec basculement vers le nord (Fig. 3A), (iii) par les linéaments sismiques associés [1].
Dans le secteur d'Herquemoulin (Fig. 1A), l'escarpement est jalonné de produits d'érosion, empilés sur une épaisseur de 30 à 40 m, sous forme de dépôts de pente type head [20] (Fig. 2).
Ces dépôts de head sont constitués de fragments anguleux (gélifracts), fortement hétérométriques, emballés dans une matrice sablo-limoneuse. La zone d'alimentation est composée du substratum gréseux ou schisteux du versant sus-jacent, tandis que l'aspect des gélifracts atteste l'absence de transport sur une longue distance. Leur présence suggère une origine cryoclastique des matériaux et une mise en place en contexte climatique périglaciaire par gélifluxions avec des phases de ruissellement et de coulées de débris [17].
2.2 Les processus de dégradation des versants
Les dépôts de head sont classiquement attribués à des processus de transfert de matière, de type cryoreptation et gélifluxion, quasi généralisés sur les versants en conditions périglaciaires [5,6,8,15]. L'intervention des cycles gel–dégel se traduit par un déplacement vers l'aval, dû à la fois (i) à la cryoreptation potentielle [19], résultat du gonflement du sol pendant le gel, et (ii) à la gélifluxion, fluage du sol, qui perd sa cohésion, pendant le dégel, lors de la libération d'eau consécutive à la fusion de la glace. Les zones de mélange de blocs hétérométriques et de matrice plus fine peuvent correspondre, quant à elles, à des coulées de débris intercalées à l'intérieur des dépôts. Ces coulées sont issues d'instabilités gravitaires sur les pentes lors d'une chute de viscosité et d'une perte de cohésion lors des périodes de fort apport d'eau [16].
L'étude d'analogues actuels en milieu périglaciaire d'altitude dans les Alpes [6] et dans les Andes [8,17] a permis d'établir les conditions climatiques (température, humidité) propices à l'élaboration de tels dépôts : (i) grande fréquence de cycles gel–dégel efficaces (plus de 200 par an) affectant le sol sur une dizaine de centimètres, (ii) apports hydriques répétés, consécutifs à de petites chutes de neige fondant rapidement. Le gel des roches se produit par des températures peu rigoureuses (−5 à −6 °C) et n'entretient une cryoclastie active que dans les groupes lithologiques dont la porosité dépasse 10 %. La mobilisation et la mise en place des dépôts s'effectue alors par gélifluxion, cryoreptation et ruissellement diffus.
2.3 La dégradation des versants au cours d'un cycle climatique du Quaternaire
Dans l'Ouest de la France, les phases de dégradation des versants avec formation des head impliquent des conditions climatiques très spécifiques, froides mais humides, qui pouvaient être réunies de manière périodique, au Quaternaire, lors des passages d'un interglaciaire à une période froide. Pendant ces périodes de transition, dont la durée est estimée entre 20 000 et 30 000 ans au maximum [15], les systèmes géomorphologiques sont fortement déséquilibrés et passent en dynamique érosive. Les cycles gel–dégel journaliers favorisent la fracturation cryoclastique des roches et la mise en mouvement des formations de surface sur les versants par cryoreptation et gélifluxion. Les flux d'eau importants, notamment lors des crues saisonnières associées à la fonte des neiges, sont à l'origine de processus catastrophiques, de type coulées de débris, qui se superposent à la cryoreptation. La végétation de type steppe froide ne peut s'opposer à l'érosion. La quantité de formations superficielles enlevées est alors importante, mais le développement d'un pergélisol en profondeur limite l'incision verticale. À titre d'exemple, lors du dernier cycle climatique, de telles conditions peuvent avoir été réunies au Weichsélien ancien entre les stades isotopiques 5a et 4 (environ
3 Approche quantitative de la dégradation d'un escarpement de faille
Pour quantifier la dégradation des escarpements de failles, il faut connaı̂tre (i) la surface de la zone d'alimentation du dépôt sur laquelle le matériau s'est préparé par gélifraction, (ii) le volume de roche érodée, (iii) le volume de matériau accumulé au pied des escarpements, (iv) la durée des processus d'érosion, le but final étant de préciser l'évolution des profils des escarpements de failles au cours des cycles climatiques du Quaternaire et, si possible, de quantifier les taux d'érosion.
La quantification de l'érosion engendrée par la combinaison des processus de cryoclastie, gélifluxion et coulées de débris a été effectuée dans l'aire drainée vers la zone de failles de Jobourg (Fig. 1B). La procédure est la suivante.
(1) La première étape consiste à calculer la surface enveloppe de la topographie de la zone d'étude, à partir d'un MNT (Modèle numérique de terrain de l'IGN : BD ALTI®, 50 m de résolution), par extraction de l'altitude des points constituant les principaux replats et lignes de crêtes. Après interpolation de ces points, la surface virtuelle obtenue correspond à la surface enveloppe de la topographie (Fig. 3A). Ce calcul est effectué avec une méthode d'interpolation locale (spline) qui repose sur deux postulats : (i) la surface doit passer le plus près possible des points à interpoler et (ii) la surface doit avoir une courbure minimale [4]. Les surfaces topographiques présentent en général un large spectre de longueurs d'onde. Pour obtenir la meilleure approximation de la forme du plateau du Nord Cotentin avant érosion, il est important de reconstituer une surface enveloppe lissée au maximum. Les pixels extraits pour reconstituer les lignes de crêtes correspondant à un seuil d'aire drainée amont nul, on s'affranchit ainsi des dépressions de petites et moyennes longueurs d'onde. L'approximation issue d'une telle reconstruction est fortement dépendante de la quantité de matière qui aurait pu exister au-dessus des lignes de crête actuelles et qui, étant érodée, ne serait pas prise en compte dans le calcul. Dans le cas présent, les lignes de crêtes portent des restes de la surface d'érosion polygénique fini-tertiaire [7] et sont considérées comme raisonnablement représentatives de la surface ante érosion.
La surface enveloppe obtenue souligne deux caractéristiques du relief local : le plateau Nord Cotentin est basculé vers le nord ; ce plateau est écorné au Sud, à l'aplomb de la faille. Cette érosion du coin supérieur sud du plateau modifie profondément le profil de l'escarpement de faille à cet endroit (Fig. 3A). Il est donc important de reconstituer la partie écornée de manière à l'intégrer dans la surface enveloppe. Pour cela, l'escarpement sud et le flanc nord du plateau (1 et 2 sur la Fig. 3B) ont été extrapolés sur une série de profils parallèles, permettant ainsi d'obtenir les points d'altitude maximale du coin manquant, (Fig. 3A). La pente de l'escarpement varie entre 19 et 50° selon le profil, avec une moyenne de 32,5°. À titre de comparaison, les valeurs de pentes d'escarpements actifs, mesurées sur le terrain, oscillent entre 23,5° [2] et 30–40° [3], tandis que les valeurs utilisées dans des modélisations sont comprises entre 32° et 41° [14].
(2) Le volume de roche érodée est ensuite calculé. Il correspond à la différence de valeur entre la surface enveloppe et la topographie, multipliée par la superficie de la zone érodée. Dans ce calcul, on distingue le volume représenté par le coin manquant (5 sur la Fig. 3B) et le volume représenté par les creux des vallées (4 sur la Fig. 3B). Le volume total de roche érodé est ensuite transformé en quantité de produit d'érosion, en tenant compte des masses volumiques respectives des roches sources (2,4 g cm−3) et des dépôts de pente (1,9 g cm−3). Ainsi, nous obtenons 0,21 km3 de produits d'érosion issus du creusement au niveau du réseau hydrographique et 0,068 km3 de produits issus de l'escarpement (Tableau 1).
Quantification des volumes d'érosion de l'escarpement de la faille de Jobourg.
Quantification of the volume of rock eroded from the Jobourg fault scarp.
Superficie | Volume érodé | Volume équivalent | Érosion moyenne | |
(km2) | (km3) | head (km3) | (m3 m−2) | |
Escarpement | 0,054 ± 0,005 | 0,068 ± 0,005 | ||
Réseau | 0,166 ± 0,005 | 0,210 ± 0,005 | ||
Total | 5,62 ± 0,14 | 0,220 ± 0,005 | 0,278 ± 0,005 | 39,0 ± 1,0 |
Head
|
1,78 ± 0,02 | 0,026 ± 0,002 | 0,033 ± 0,002 | 4,6 ± 1,0 |
(3) Le volume du prisme de matériau accumulé au pied des escarpements est calculé à partir de la surface cartographique, de coupes verticales et d'une estimation du volume manquant (6 et 7 sur la Fig. 3B). Le volume de matériau accumulé au pied des escarpements s'appuie sur la cartographie des dépôts de pente [20]. Ces dépôts sont à l'aplomb d'une zone d'alimentation constituée du bassin versant d'Herquemoulin (1 sur la Fig. 1B) et de deux facettes triangulaires présentant des écoulements de moindre importance (2 sur la Fig. 1B). Les dépôts cartographiés ont été géoréférencés et convertis en semis de points affectés de leurs altitudes. La partie distale du prisme a été extrapolée à partir de ces points. Le prisme s'étend ainsi sur près de 500 m de large et 3250 m de long, avec une pente moyenne de 7° vers la mer à son sommet. Il repose sur un platier rocheux très faiblement penté (0,3°).
Cette quantification donne une valeur de 39 m3 m−2 pour le volume total érodé, ramené à la surface de l'escarpement drainé, soit une tranche totale érodée de 39 m d'épaisseur. Les produits de l'érosion de l'escarpement, encore stockés au pied de la faille, représentent un volume de 4,6 m3 m−2, soit une tranche érodée de 4,6 m d'épaisseur (Tableau 1). Les produits de l'érosion observables ne représentent donc que 1/8 de l'érosion totale de l'escarpement. Dans ce calcul, la barre d'erreur de ±1 m3 m−2 (Tableau 1) est liée, entre autres, à la précision des documents topographiques utilisés, à la densité du semis de points interpolés, aux approximations et artefacts liés à l'interpolation.
(4) L'étape suivante concerne le calcul du taux d'érosion de l'escarpement au cours d'un cycle climatique du Quaternaire. Ce calcul est fortement dépendant du nombre de cycles climatiques enregistrés dans les dépôts stratifiés en pied d'escarpement. Les travaux précédents [13] ont conclu à l'enregistrement de deux cycles climatiques (saalien et weichsélien) en se basant sur la présence d'un niveau de lœss discontinu au sein des heads. Si tel était le cas, le taux d'érosion serait de 2,3 m3 m−2 par cycle. Dans l'attente des datations en cours, on peut aussi penser que les dépôts de head observables à Jobourg ont pu se former uniquement au cours du dernier cycle climatique (Weichsélien). Les taux d'érosion de l'escarpement de faille seraient alors d'environ 4,6 m3 m−2 par cycle. Deux arguments au moins militent en faveur de cette deuxième hypothèse. Le premier argument est l'absence de discontinuité et de coupure majeure dans la série de head, à l'exutoire des thalwegs, qui refléteraient les incisions successives. Le deuxième argument est lié à la très faible résistance des heads à l'érosion marine actuelle. Or, il est admis que le niveau marin de l'interglaciaire précédent (Éémien) était plus haut que l'actuel. Si tel a été le cas, les heads du cycle saalien ont du être totalement déblayés en quelques siècles à l'Éémien. À titre de comparaison, les mesures effectuées en milieu de haute altitude, analogue actuel du contexte périglaciaire, mais sur des pentes plus faibles, indiquent une érosion des versants rocheux de 2 à 3 m par cycle climatique [6]. Les résultats obtenus sur l'escarpement de Jobourg sont donc cohérents en ordre de grandeur.
Cette quantification permet aussi une première estimation de l'âge du début de l'érosion de l'escarpement. Si l'on admet une érosion par cycle de 4,6 m3 m−2, le volume total érodé de 39 m3 m−2 impliquerait 8 cycles climatiques, soit environ 1 Ma. Cet âge de début de l'érosion peut correspondre à la mise à jour de l'escarpement de l'ancienne faille varisque de Jobourg. Il est à rapprocher du fort rajeunissement du relief mis en évidence par la géomorphométrie [11], même si les premiers mouvements verticaux enregistrés dans la région sont plus anciens, d'âge Pléistocène inférieur [11,13].
4 Conclusion
Dans l'Ouest de la France, les formes du relief sont fortement influencées par les processus climatiques cryoclastiques, qui opèrent de manière périodique en contexte périglaciaire au Quaternaire. Ces processus donnent naissance à des dépôts de pente grossiers accumulés au pied des failles et jouent un rôle prépondérant dans la dégradation des versants. Ils contribuent, de ce fait, à occulter la signature morphologique des déformations plio-quaternaires.
La méthode présentée de quantification de la dégradation d'un escarpement de faille combine les mesures de terrain avec le traitement d'une topographie numérisée. Cette quantification donne, dans le cas de la faille de Jobourg, des valeurs de 39 m3 m−2 pour le volume total érodé, alors que les produits de l'érosion, encore stockés au pied de la faille, ne représentent qu'un volume de 4,6 m3 m−2, soit 1/8 de l'érosion totale de l'escarpement. Dans l'hypothèse où les produits de l'érosion stockés sont à rattacher à un seul cycle climatique, cette quantification suggèrerait qu'au moins huit cycles climatiques, soit environ 1 Ma, ont été nécessaires pour la dégradation de l'escarpement. Cet âge, relativement jeune, est compatible avec l'immaturité du relief et les perturbations du réseau hydrographique associées [11].
Pour compléter cette étude, une simulation expérimentale de la dégradation des escarpements sous l'effet des cycles gel–dégel est en cours.
Vous devez vous connecter pour continuer.
S'authentifier