Version abrégée
1 Introduction
Les monts Apuseni (Roumanie) se situent dans la zone interne de la chaı̂ne des Carpathes. Ils sont caractérisés par une série d'unités tectoniques, qui appartiennent à la marge continentale du bloc de Tisza.
Des indicateurs de l'évolution géodynamique du bloc de Tisza sont bien préservés au sein des unités tectoniques des monts Apuseni, dont la structuration est encore très peu connue.
Cet article a pour objet la reconstruction de l'histoire tectonique et l'estimation du degré métamorphique de la nappe de Fenes, qui affleure dans les monts Apuseni méridionaux.
2 Cadre géologique
Les Carpathes (Fig. 1) représentent une chaı̂ne orogénique d'âge Alpin, liée à la convergence Mésozoı̈que–Cénozoı̈que entre les fragments des plaques africaine et eurasienne. La zone interne de la chaı̂ne des Carpathes, qui correspond au « Bassin pannonien », est caractérisée par un substrat que l'on retrouve dans certaines zones, où affleurent des roches métamorphiques ayant subi plusieurs phases de déformation. Ces roches sont plus anciennes que le Néogène, comme celles des monts Apuseni (Fig. 1).

Tectonic sketch map of the southeastern sector of the Apuseni Mts (a) and schematic cross section of the Trascau Mts (b); the location of the cross section and the study area are indicated. Explanation: 1, main thrusts; 2, secondary thrusts; 3, main faults; 4, Neogene sedimentary cover; 5, Neogene calc-alkaline intrusions and related subvolcanic rocks; 6, Banatitic intrusions and related volcanites; 7, Mures nappe; 8, Cretaceous Flysch units; 9, Biharia nappes complex; 10, Codru nappes complex; 11, Bihor nappes complex; 12, Getic nappe.
Schéma tectonique du secteur sud-oriental des monts Apuseni (a) et coupe schématique des monts Trascau (b) ; position de la coupe schématique et de la zone étudiée. Légendes : 1, principaux chevauchements ; 2, chevauchements secondaires ; 3, failles principales ; 4, couverture sédimentaire du Néogène ; 5, intrusions néogènes calc-alcalines et roches sub-volcaniques associées ; 6, intrusions banatitiques et volcanites associées ; 7, nappe de Mures ; 8, unités flyschoı̈des du Crétacé ; 9, série de nappes de Biharia ; 10, série de nappes de Codru ; 11, série de nappes de Bihor ; 12, nappe gétique.
D'après les données géophysiques, ces roches appartiendraient au bloc Tisza. La partie superficielle de la micro-plaque Tisza [16] est exposée au nord des monts Apuseni (Fig. 1), où affleurent des unités tectoniques cristallines [7,16], chevauchées par des unités flyschoı̈des du Crétacé [4,11] et par la nappe ophiolitique de Mures [14].
Parmi les unités flyschoı̈des, une des plus importantes est la nappe de Fenes, qui affleure dans les monts Apuseni méridionaux et qui est constituée par une succession sédimentaire, en accord avec Bleahu et al. [4].
L'analyse structurale a été menée au sein de l'épaisse succession de turbidites silicoclastiques, qui passent, vers le haut, à des conglomérats polymictes représentant le sommet de la formation de Fenes (cf. carte géologique de la Roumanie, échelle ) [12]. Les déformations de la nappe de Fenes sont scellées par des intrusions banatitiques, associées à des volcanites (Maastrichtien tardif–Paléocène) [5].
3 Histoire de la déformation
L'histoire de la déformation proposée pour la nappe de Fenes se base sur une nouvelle campagne géologique et des analyses microstructurales effectuées dans la zone comprise entre les vallées de Ighiel et de Galda des monts Trascau, au sud des monts Apuseni.
D'après les données recueillies, l'histoire de la déformation de la nappe de Fenes comprendrait deux phases de plissement (D1 et D2). L'âge des formations impliquées et de leurs relations avec les dykes banatitiques, qui n'ont pas subi de déformation, indiquent que les déformations se sont produites dès le début de l'Aptien jusqu'au Maastrichtien tardif.
Plus en détail, les principales caractéristiques des phases de déformation sont les suivantes.
La phaseD1 est caractérisée par des plis semblables, très serrés à isoclinaux, avec des charnières épaisses. Les axes A1 sont assez dispersés (Fig. 3a), même s'ils se concentrent autour de la direction sud–sud-ouest. Ces plis sont associés à une foliation de plan axial, représentée par une surface continue et pénétrative, généralement parallèle à la stratification S0. L'orientation des méso-structures D1 et les rapports S0/S1 indiquent une vergence vers l'ouest-nord-ouest. Dans les argiles, la foliation de plan axial S1 (slaty cleavage) (Fig. 2b) observée en lame mince paraı̂t caractérisée par l'association métamorphique suivante : illite + chlorite + albite + quartz + calcite (Fig. 2b). Diverses familles de veines syn-tectoniques ont été remarquées dans les siltites et les argilites ; elles sont représentées par des ombres de pression et des veines en extension, remplies par du quartz, par de la calcite et, plus rarement, par des phyllosilicates. Les caractéristiques microstructurales indiquent une direction de cisaillement vers l'ouest-nord-ouest. Toutes les observations microscopiques indiquent donc que le pic des conditions de métamorphisme a été atteint durant la phase D1.

Equal-area, lower hemisphere stereographic representation of D1 and D2 structural data. a, A1 axes; b, A2 axes; c, S0 bedding planes.
Représentation stéréographique (projection équivalente de Schmidt, hémisphère inférieur) des données structurales de D1 et D2. a, axes A1 ; b, axes A2 ; c, stratification S0.
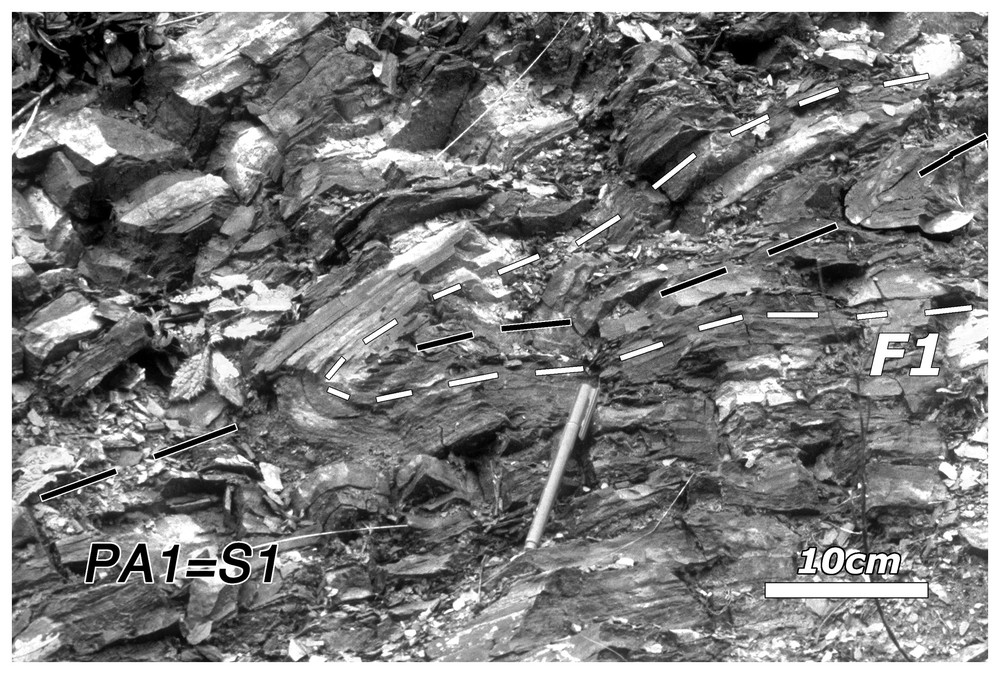
D1 phase, sub-isoclinal fold (F1) recognised in the Fenes Fm. The axial plane (PA1) is indicated.
Phase D1, pli sub-isoclinal (F1) dans la formation de Fenes. Le plan axial (PA1) est indiqué.
Au sein de la nappe de Fenes, les structures associées à la phase D2 sont les plus répandues et les mieux développées ; elles se superposent aux structures de D1, produisant des familles d'interférence de type 3 [14]. Ces structures, centimétriques à métriques, sont constituées par des plis asymétriques et renversés. Les surfaces axiales de ces plis sont représentées par des surfaces subhorizontales, tandis que les axes A2 (Figs. 3b et 3c) se concentrent autour de la direction ENE–WNW. Les données structurales suggèrent une vergence des plis F2 vers l'ouest-nord-ouest. Dans les argiles, la foliation S2 est représentée par un clivage de crénulation discret.
Les déformations tardives sont principalement représentées par une famille de failles subverticales avec direction variable du nord-ouest au sud-est.
4 Degré métamorphique
Le degré métamorphique a été déduit par l'analyse de l'association minéralogique et des valeurs de la cristallinité de l'illite et de la chlorite sur 17 échantillons de pélites de la succession turbiditique.
Les roches analysées sont des pélites, constituées principalement d'illite et de chlorite et, en plus petite quantité, par du quartz, de la calcite et de l'albite. Dans la plupart des échantillons, la quantité d'interstratifiés illite–smectite (I–S) est faible à modérée. La serpentine est présente uniquement en faible quantité dans deux échantillons. Le K-feldspath et le plagioclase, bien qu'assez répandus, représentent un composant secondaire. En lame mince, le plagioclase, le K-feldspath, la serpentine, l'apatite, le rutile et le zircon sont des minéraux détritiques, tandis que le mica blanc, la chlorite, le quartz, l'albite et la calcite sont, soit des minéraux détritiques, soit des minéraux métamorphiques, qui se sont cristallisés pendant la phase D1. La paragenèse métamorphique des pélites est donc représentée par : I+Chl+Ab+I–S+Qtz+Calc.
La distribution des données IC10 Å et ChC7 Å est illustrée dans les diagrammes de la Fig. 4, qui ont été obtenus à partir de préparations orientées séchées à l'air. Sur la même figure sont aussi reportées les valeurs moyennes des deux index ; dans le cas de l'illite, on a reporté, soit la valeur moyenne issue des préparations orientées séchées à l'air (AD), soit la valeur moyenne tirée des préparations traitées à l'éthylène glycol (EG).

S1 slaty cleavage with well developed pressure shadows around a detrital quartz grain (qtz) filled in by phyllosilicates (phy) in siltstone from Fenes Fm.
Slaty cleavage S1 avec des zones abritées bien développées autour d'un grain de quartz (qtz) détritique, remplies par des phyllosilicates (phy), dans une siltite de la formation de Fenes.
Les valeurs de IC10 Å des échantillons séchés à l'air (AD) standardisées par rapport aux standards CIS préparés par Warr et Rice [17] sont comprises entre 0,41 et 0,65, avec une moyenne de 0,51±0,05° . En considérant les valeurs IC proposées par Kübler [8,9] et adoptées par l'échelle CIS [18] pour les limites diagenèse/anchizone et anchizone/épizone, les données recueillies suggèrent un degré métamorphique de diagenèse supérieur.
En calibrant les données par rapport aux standards de Kübler, on obtient une valeur moyenne IC10 Å de 0,47° pour les pélites analysées et, en appliquant les limites de la cristallinité de l'illite de Kübler [8,9], les échantillons s'approcheraient encore plus de la limite diagenèse/anchizone, en accord avec les données structurales. Ce degré métamorphique est confirmé par les macles des fibres de calcite des veines en extension, qui appartiennent aux types I et II de Burkhard [6].
Les valeurs de cristallinité de l'illite et les macles de la calcite indiquent donc une déformation D1 syn-métamorphique, à des températures comprises entre 150 et 200 °C.
Tout comme pour la cristallinité de l'illite, l'indice (AD) ChC7 Å présente des valeurs comprises entre 0,35 et 0,59 (°), avec une moyenne de 0,47±0,06 (°). La valeur moyenne de ChC7 Å des échantillons examinés donne des résultats qui coı̈ncident avec ceux donnés par l'indicateur IC10 Å.
5 Conclusions
Les données recueillies indiquent que la nappe de Fenes a été déformée par deux phases principales, D1 et D2. La phase D1 est caractérisée par des plis, très serrés à isoclinaux, associés à une foliation de type slaty cleavage. Le degré métamorphique atteint durant la phase D1 indique une déformation qui se serait développée dans des conditions métamorphiques de diagenèse tardive (limite diagenèse/anchizone), correspondant à un intervalle de température de 150–200 °C.
En considérant un gradient géothermique de 20 °C km−1, valeur généralement utilisée dans un contexte de collision continentale (ex. [13]), la profondeur de déformation de la nappe de Fenes peut être évaluée à 8–10 km.
La phase de déformation D2, moins intense, a produit des plis parallèles renversés. La phase D2 se serait donc développée durant l'exhumation de la nappe de Fenes. La remontée qui a eu lieu durant le Crétacé tardif se caractérise par une translation de la vergence, qui passe d'ouest-nord-ouest durant D1 à nord-nord-ouest durant D2.
1 Introduction
The Apuseni Mts (Romania) are located in the inner zone of the Carpathian belt. They are characterised by a complex stack of tectonic units belonging to the continental margin of the Tisza block. Some of these units include thick sedimentary successions of Cretaceous age, which represent orogenic-related deposits involved in the Late Cretaceous tectonic phases. These phases, which are regarded as the results of the most important orogenic events that affected the Carpathian area, are still poorly known.
The aim of this paper is to reconstruct the deformation history and to evaluate the metamorphic grade of the Fenes Nappe, from the southern Apuseni Mts.
2 Geological setting
The Carpathians (Fig. 1) are an orogenic belt of Alpine age, originated from the Mesozoic–Cainozoic convergence of the continental fragments derived from the African plate's margin into the Eurasian plate (e.g., [16]). The related crustal shortening was coeval to the extension in the inner zone of the Carpathian belt, i.e., the Pannonian basin, where Neogene deposits and calc-alkaline volcanites largely occur (Fig. 1). The substrate of the Pannonian basin is represented by polydeformed and metamorphosed rocks older than Neogene. The geophysical data suggest that these rocks are representative of different continental microplates, referred to as Alcapa, Tisza (also known as Tisia) and Dacia blocks.
The lowermost levels of the Tisza microplate are exposed in the Northern Apuseni Mts, where a stack of tectonic units has been recognised (Fig. 1). These units are represented, from the bottom to the top, by the Bihor, Codru, and Biharia complexes [16]. These complexes, also known as Internal Dacides, were deformed during two main Cretaceous tectonic events. The first one (‘Austrian’ phase) occurred in the time spanning from Aptian to Albian, whereas the second event (‘Laramian’ phase) occurred during Senonian [7]. The second event was marked by the development of sedimentary basins filled by ‘Gosau’ deposits of Santonian to Early Maastrichtian age.
In the Southern Apuseni Mts, the Biharia complex is overlain by a stack of tectonic units, mainly represented by Fenes, Grosi, Cris, Bucium, Valea Mica Galda and Bozes nappes [4,11], in turn topped by the ophiolite-bearing Mures nappe [15]. These units, reported all together as Cretaceous Flysch units in Fig. 1, are basically made up of a Cretaceous sedimentary succession, consisting of coarse-grained siliciclastic deposits. Among the Cretaceous Flysch units one of the most important is the Fenes Nappe, which crops out in the southern Apuseni Mts. According to Bleahu et al. [4], this unit consists of a sedimentary succession, whose oldest formation, referred to as Fenes Formation (Barremian–Early Aptian), is mostly made up of turbiditic deposits. The Fenes Formation grades up into the Valea Dosului Formation, consisting of Aptian calcareous coarse-grained turbidites. These formations are unconformably overlain by the Metes Formation, composed of Late Aptian to Middle Albian conglomerates. The youngest formation of the Fenes Nappe succession is represented by the Valea Lui Paul Formation, consisting of Albian–Cenomanian siliciclastic turbidites that unconformably cover all the underlying deposits. Bleahu et al. [4] suggest a Jurassic ophiolitic basement for the succession of the Fenes Nappe.
In the study area, the Metes and Valea Lui Paul Formations are lacking and the structural analyses have been performed in the upper part of the Fenes Formation, according to the 1:200 000 scale geological map of Romania [12]. All the deformations recognised in the Fenes Nappe are sealed by undeformed Late Maastrichtian to Palaeocene Banatitic intrusions and related volcanites [5].
3 Deformation history
The proposed deformation history of the Fenes Nappe is based on new field structural analyses, mostly performed within the turbiditic sequences cropping out in the area between the Ighiel and Galda valleys of the Trascau Mts, Southern Apuseni Mts. These analyses have been accompanied by microstructural studies on selected, oriented samples, in order to assess the relationships between deformation and metamorphism.
The collected data suggest that the Fenes Nappe experienced a deformation history consisting of two distinct folding phases (D1 and D2), followed by late, brittle events of Tertiary age. Owing to the lack of radiometric dating, the age of the deformation phases is only constrained by the age of the formations involved in the structures and by their relationships with undeformed banatitic dykes. The Early Aptian age of the siliciclastic turbidites and polymict conglomerates can be assumed as the onset of the deformation phases. These deformations are, in turn, older than Late Maastrichtian, according to their relationships with the banatitic dykes [5]. Consequently, the D1 and D2 phases developed in the time spanning from Early Aptian to Late Maastrichtian.
More in detail, the main characters of the deformation phases are the following:
3.1 D1 phase
It is characterised by very tight to isoclinal F1 folds, with thickened hinge zones; these folds (Fig. 2), distinguished by an approximately similar geometry, are very common in the siliciclastic turbidites. The boudinage along the limbs is poorly developed, but the necking of the more competent layers can be observed. The distribution of the axes A1 shows a cluster around NNE–SSW (Fig. 3a). These folds are associated with an axial plane foliation (S1), recognised as a continuous and penetrative surface, generally parallel to the bedding (S0). The LS0–S1 intersection lineations are mainly represented by an axial-plane foliation-bedding intersection and mullion structures. The L1 mineral lineations occur as calcite and/or quartz fibres filling in pressure shadows around pyrite crystals on S1 surfaces. The L1 and LS0–S1 lineations are mostly sub-perpendicular and sub-parallel, respectively, to the cluster of the A1 axes. The facing of the D1 meso-scale structures, as well as the S0/S1 relationships, indicates a WNW vergence.
In the shales, the axial-plane foliation S1 can be classified as a slaty cleavage (Fig. 4). In thin section, this slaty cleavage, developed only in the finest lithologies, is characterised by the following metamorphic mineral assemblage: illite + chlorite + albite + quartz + calcite; it appears defined by aligned illite and chlorite flakes and elongated quartz–albite–mica aggregates showing the effects of pressure-solution processes (Fig. 4).
In the shales and siltstones, various sets of syntectonic veins, represented by pressure shadows and extension veins, have been recognised. These veins were developed during the D1 phase, as can be deduced by their relationships with D1 and D2 structures and by their increase in the most deformed areas. The extension veins exhibit both mosaic and fibrous textures. In thin section, the veins with a fibrous texture are characterised by antitaxial quartz and/or calcite fibres. The pressure shadows closely associated with S1 slaty cleavage are generally observed around detrital minerals, such as quartz, feldspar and chlorite, as well as around framboidal pyrite. The pressure shadows, generally filled by calcite, phyllosilicates and/or quartz fibers, show asymmetrically re-crystallised tails that indicate a non-coaxial deformation history, coherent with a top-to-WNW sense of shear.
In summary, all the microscopic observations indicate that the peak metamorphic conditions were attained during the D1 phase.
3.2 D2 phase
In the Fenes Nappe, the structures of the D2 phase are the most widespread and the best developed. They are everywhere superimposed on the D1 structures, producing a type 3 interference pattern [14]. These structures consist in overturned and asymmetric F2 folds, developed at a centimetre- to a metre-scale, having an approximately parallel geometry and interlimb angles ranging from 50° to 120°. The axial planes of these folds occur as low-angle planes, whereas the A2 axes (Fig. 3b) cluster around ENE–WNW, as suggested also by the S0 density diagram of Fig. 3c. The S0/S1/S2 relationships and ‘s’ and ‘z’ sense of fold asymmetry criteria consistently suggest a NNW vergence for the F2 folds. The S2 axial-plane foliation developed in the shales can be recognised as a discrete crenulation cleavage, ranging from spaced to zonal types. On the contrary, in siltstones and sandstones, the S2 foliation can be classified as a disjunctive cleavage. In thin sections, no mineralogical recrystallisation along the S2 foliation has been observed.
A set of syntectonic shear veins associated with D2 structures has been recognised. The more developed of such veins are marked by sheets of calcite fibers on slip surfaces usually coinciding with bedding surfaces. These fibers are oriented in a direction sub-perpendicular to the A2 axis, a character that supports the association of the veins with the D2 folds. The sense of shear deduced from some small, step-like features observed on calcite fibers of the slip surfaces, is reversed along the F2 fold limbs.
The latest deformations are mainly represented by a set of high angle, strike-slip faults, showing a clear NW–SE trend.
4 Metamorphic grade
The metamorphic grade of the Fenes Nappe has been investigated through mineral assemblages and ‘crystallinity’ of illite and chlorite on 17 samples from the pelites of the turbidite succession.
Whole-rock samples were analysed as normal powders, while <2 μm fraction samples were investigated through oriented aggregates, using standard X-ray powder diffraction techniques on a Philips PW 1710 automatic diffractometer equipped with a long fine-focus Cu tube. The illite (IC) and chlorite (ChC) ‘crystallinity’ indices (half-height peak width expressed as °) were measured on experimental XRD patterns collected on the <2 μm fraction samples sedimented on glass slides. Care was taken to avoid thin slides: the amount of clay on each slide was at least 3 mg cm−2. For mica, the measurements were performed on the (001) basal reflection both on air-dried (AD) and ethylene glycol-solvated (EG) specimens; for chlorite, the measurements were effected on the (002) peak only on air-dried specimens, after that a preliminary survey showed that no change occurred in this reflection in response to glycolation treatment. Hereafter these data will be referred to as IC10 Å and ChC7 Å, respectively.
The (002) reflection of chlorite was selected because: (a) it is the strongest peak of the mineral; (b) at odds with (001) reflection, it is not partially overlapped by the large diffraction band due to the illite/smectite mixed-layer minerals, which are present in most samples; (c) it is substantially free from any overlapping by the (001) reflection of kaolinite, since this mineral never is present in detectable amounts. Each sample was run at the following instrumental conditions: Cu Kα Ni-filtered radiation; 40 kV; 20 mA; slits: (1/2)° divergence and scatter, 0.2 mm receiving; continuous scanning; scan speed: 0.25° ; time constant 4. Experimental profiles were analysed using Krumm's WINFIT programme [8], which determines the ‘crystallinity’ by first subtracting the background from the raw data, then operating the peak fitting through an asymmetrical Pearson VII function. Single-peak fits have been carried out for both illite 10 Å- and chlorite 7 Å-reflection. Experimental data were then converted to calibrated CIS data using the procedure and standards (SW1, SW2, SW4, SW6 and MF1) of Warr and Rice [17].
The investigated rocks are pelites chiefly consisting of illite-muscovite and chlorite, with subordinate amounts of quartz, calcite and albite. Mixed-layer illite/smectite minerals (I–S) occur in small to moderate amounts in most of the examined samples. Minor amounts of serpentine have been observed only in two samples. K-feldspar and plagioclase occur as widespread but minor components. Accessory minerals are: apatite, rutile, zircon, and pyrite.
Optical observation of thin sections shows that plagioclase, K-feldspar, serpentine, apatite, rutile, and zircon occur only as detrital minerals, whereas illite–muscovite (I), chlorite, quartz, albite and calcite are present as both detrital and metamorphic minerals, crystallised during the D1 phase. The metamorphic paragenesis of pelites resulting from optical and X-ray data is then: I+Chl+Ab+I–S+Qtz+Calc.
The distribution of IC10 Å and ChC7 Å data is reported in histograms of Fig. 5, which refer to measures on air-dried specimens. In this figure, the average ‘crystallinity’ index values are also given; for illite, they have been calculated both from measures on air-dried (AD) and ethylene glycol-solvated (EG) specimens, for chlorite only AD data have been considered.


Distribution of illite (IC) and chlorite (ChC) ‘crystallinity’ indexes evaluated in the Fenes Nappe. IC boundaries between metamorphic zones from Kübler [9,10]. x̄ = average value; σ = standard deviation; AD = values from air-dried slides; EG = values from ethylene glycol-solvated slides; N = number of examined samples.
Distribution des valeurs de « cristallinité» de l'illite (IC) et de la chlorite (ChC) dans la nappe de Fenes. Limites IC des zones métamorphiques d'après Kübler [9,10]. x̄ = valeur moyenne ; σ = dévation standard ; AD = valeurs mesurées sur préparations sechées à l'air ; EG = valeurs mesurées sur préparations traitées à l'éthylène glycol ; N = nombre d'échantillons examinés.
IC10 Å values from air-dried (AD) specimens range from 0.41 to 0.65, with an average value of 0.51 ±0.05° . Glycol treatment appears to produce only a small decrease of the index value (on average about 8%), a feature most likely related to the presence of moderate amounts of illite/smectite mixed layers minerals. Assuming the IC values proposed by Kübler [9,10] and adopted by the CIS scale [18] as characteristic of the diagenetic-zone/anchizone and anchizone/epizone boundaries (0.42 and 0.25 as °, respectively), the data collected in the present study suggest a late-diagenetic grade for the studied samples. When the calibration is performed with respect to Kübler's standards, an average IC10 Å value of 0.47° is obtained. This value would shift the investigated pelites in a zone even more close to the diagenetic-zone/anchizone boundary, which is more consistent with the structural evidence given by the development of a slaty cleavage and a pervasive isoclinal folding in the turbidites. This metamorphic grade is also supported by the intracrystalline deformation in the extensional veins, where the twinning in the calcite fibers belongs to the type I and type II of Burkhard [6]. On the whole, the illite ‘crystallinity’ data and the evidence from calcite twinning indicate a D1 syn-metamorphic deformation under thermal conditions in the range 150–200 °C.
As to chlorite ‘crystallinity’, the (AD) ChC7 Å index present values varying from 0.35 to 0.59 (as ), with an average value of 0.47±0.06 (as °). Although in low and very-low grade metamorphism ChC results to be, in general, positively correlated with the IC index, up to date no widely accepted ChC scale is available. The most detailed studies on the subject have been carried out by Árkai [1–3], who attempted at defining anchizonal limits for both ChC(001) and ChC(002) [2]. In the present study, application of these limits to the average ChC7 Å value found in the studied samples produces results largely coincident with those given by IC scale.
5 Conclusions
The data collected in the present work indicate that the Fenes Nappe, until now regarded as an unmetamorphosed and weakly deformed sequence, experienced a polyphase deformation history. Such a history includes two main deformation phases, D1 and D2.
The D1 phase is characterised by isoclinal to very tight folds, associated to a slaty cleavage. The collected data indicate that D1 deformation phase developed under late diagenetic to diagenetic-zone/anchizone boundary conditions, consistent with temperatures in the range 150–200 °C. Assuming a geothermal gradient of about 20 °C km−1, as generally recognised in the continental collision setting (e.g., [13]), the depth of deformation of the Fenes Nappe during the D1 phase can be roughly estimated as corresponding to 8–10 km.
The later D2 phase is characterised by a less intense deformation, which mainly produced overturned, parallel folds without a synkinematic recrystallisation. This implies that the D2 phase was developed at shallower crustal levels, most likely during the exhumation of the Fenes Nappe. This exhumation, developed during the Late Cretaceous time, was characterised by a vergence shift, from the WNW-vergence dominating during the D1 phase to a NNW-vergence prevailing during the D2 phase.
To sum up, the D1 and D2 folding phases experienced by the Fenes Nappe during the Early Aptian to the Late Maastrichtian time span may be regarded as the record of a structural path, which includes a burial at a depth of 8–10 km and a later exhumation at shallower structural levels. Moreover, the D1 and D2 phases identified in the Fenes Nappe can be tentatively correlated with the ‘Austrian’ and ‘Laramian’ phases recognised in the Bihor, Codru, and Biharia complexes.
Acknowledgements
This research was supported by the ‘Institutul Geologic al Romaniei’, by the Italian National Research Council (CNR, ‘Centro di Studio per la Geologia Strutturale e Dinamica dell'Appennino’) and by the Italian Ministry for University and Scientific and Technological Research (MURST – Projects ‘Layer silicates: crystal-chemical and physical properties; petrological, microstructural and technological applications’ and ‘Geodynamic significance of the ophiolite sequences in the collisional orogens’).