Abridged version
Many areas, for which mineral exploitations have been intensively developed, constitute nowadays high-risk zones in urban planning and development. For example, the Seine-et-Marne French department is highly concerned by underground cavities resulting from old gypsum exploitations, since a territory of about 10 km2 is affected around the commune. This problematic was identified by the BRGM, which realised in 1997 a national inventory of underground cavities from different kinds of archives [7]. In order to extend this database when no information was available, we decided to test seismic methods to detect underground cavities from the surface. We focussed our study on the commune of Annet-sur-Marne, for which many old gypsum cavities are present in the first 50 m.
In the last decades, high-resolution seismics have been extensively applied to cavity detection [1,2,5,8]. These studies showed that the cavities could be detected by the lacking effect that they produce on the later reflections [6]. When the cavity size is smaller compared to the wavelength, diffraction processes can also occur and wave diffusion can be invoked [3]. Such features are described and commented in this study.
The underground cavities studied here result from gypsum exploitation. They are located in three Ludian layers between 30 and 80 m deep, each of them being separated from the others by marl layers (Fig. 1a). The seismic profile was set so that its first third was above a non-exploited area, while the other part was above cavities (Fig. 1b). Quite good field conditions were reached: straight seismic line, no topography, although a road noise affected sometimes the records, especially for far offsets. The acquisition system (StrataView RX) consisted of 60 channels – 24 bits coding system, 14 Hz geophones and a SISSY gun source (30 g). Geophones and source spacing was 3 m, and a total of 100 shots was recorded.
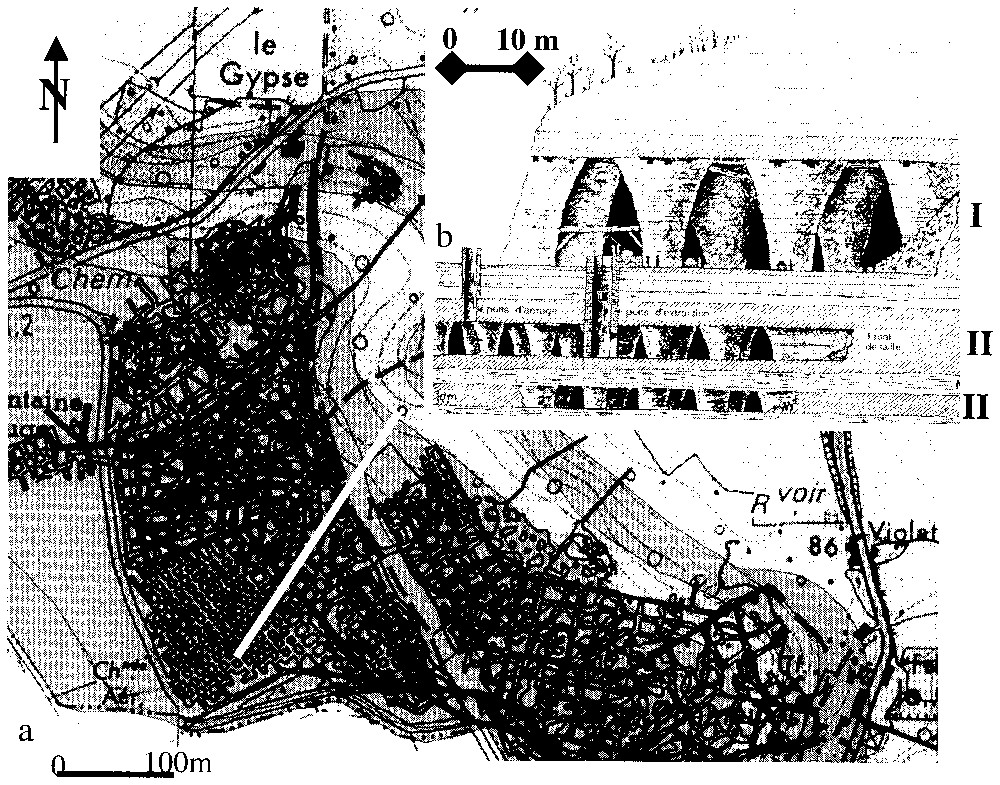
a. Plan de position du profil sismique (trait blanc) par rapport aux réseaux des cavités souterraines. b. Schéma d'une exploitation souterraine dans la région d'Annet-sur-Marne. Les chiffres romains indiquent les trois niveaux d'exploitation.
a. Location of the seismic profile (white line) with respect to the underground quarries network: the first third of the profile is located in an unexploited area, while other parts cover the exploitation. b. Sketch of an underground quarry in the Annet-sur-Marne surroundings. Roman numbers indicate the three exploited levels.
Data processing was realised with the Promax software and consisted in several steps. First, a severe trace rejection was used to reject noisy traces, particularly for far offsets. To attenuate surface waves and high-frequency noise, a band-pass filter between 90–110–250–350 Hz was applied. As results, several hyperbolas features can be observed on the records between 0 and 150 ms (Fig. 2a). Aerial wave (Figs. 2a and 2b) was removed by using an air blast mute (Figs. 2c and 2d). Because the reflectors are supposed to be superficial, no AGC was used, but a trace normalisation of amplitudes was performed to compensate the attenuation with respect to the offset. A deconvolution was also applied, and a particular attention was accorded to first arrival muting. Hyperbolas observed on Fig. 2a were used to estimate velocities (1200 to 1600 m s−1) for NMO corrections. Finally, 320 CDPs were gathered with a maximum folder of 24 because of the trace rejection.
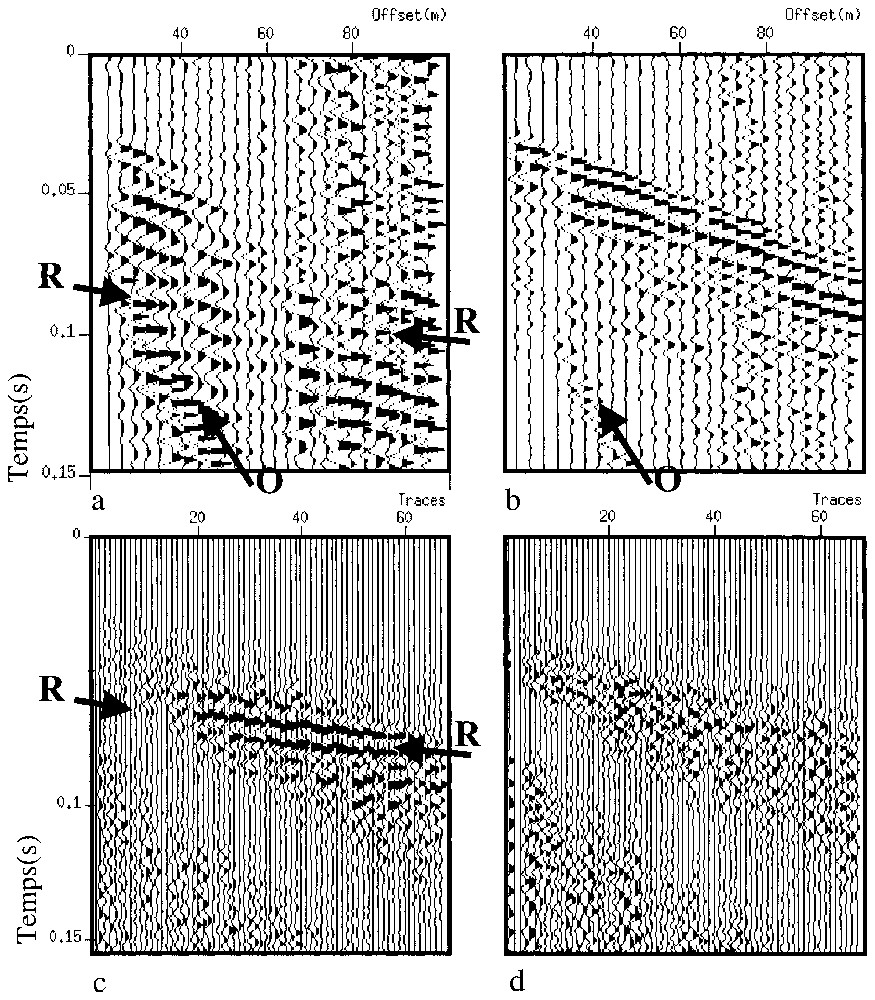
Tirs sismiques (a, b) et super CDP (c, d) dans le premier tiers (a, c) et dans les deux derniers tiers (b, d) du profil. Des réflexions (R) sont visibles en (a) et (c). (O) correspond à l'onde aérienne.
Seismic shots (a, b) and super CDPs (c, d) in the first third (a, c) and in the two last thirds (b, d) of the profile. Note the reflection (R) in (a) and (c). (O) is the aerial wave.
As shown in Fig. 3, strong reflections are clearly visible between 50 and 100 ms in the first 150 m of the profile. These signals are attributed to gypsum–marl interfaces located between 30 and 70 m in depth. For distances larger than 150 m along the profile, no reflections occur, this limit corresponding to the presence of cavities. This feature is interpreted as interferences between reflections on interfaces and diffractions on cavity structures leading to incoherent diffusion of the wave front.
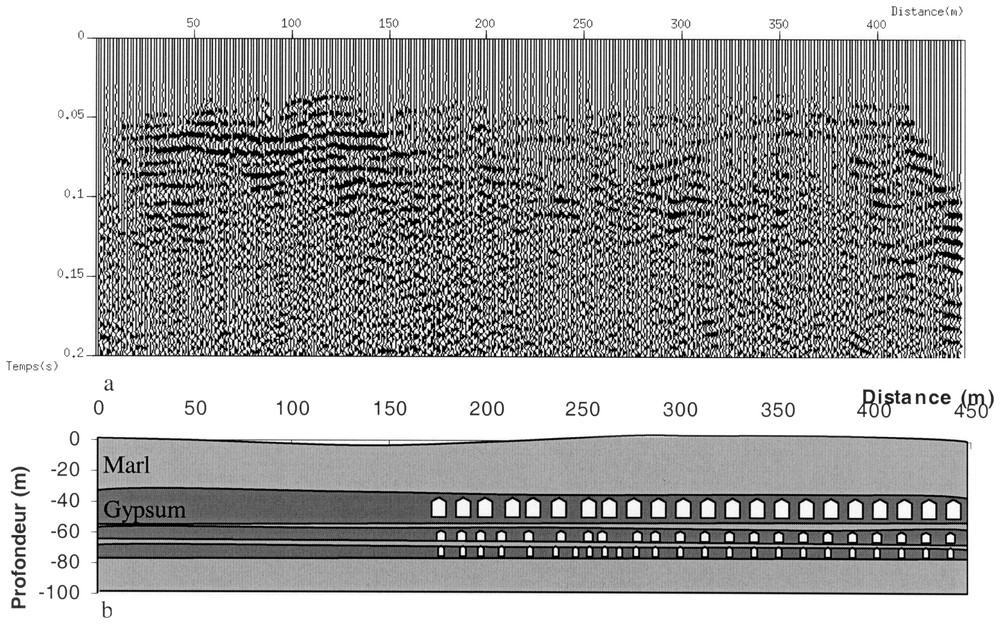
a. Section sommée montrant l'arrêt des réflexions situées entre 40 et 100 ms à partir de la distance 150 m. b. Coupe schématique.
a. Stack section showing the interruption of reflections occurring between 40 and 100 ms from the distance of 150 m. b. Schematic cross-section.
To illustrate this hypothesis, we computed several synthetic seismograms using a 2D FDTD code [9], in which an additional parameterisation was introduced to take into account the interaction between elastic waves and free surface boundaries of a cavity [4]. The reference model is indicated in Table 1. Fig. 4 shows three synthetic seismograms computed respectively with no cavity (a), one cavity (b) and three cavities (c). Each cavity has dimensions of 10 m×10 m, and is located at a depth of 30 m. The first synthetic (Fig. 4a) shows a strong reflection (R) coming from the main interface of the model. When a cavity is present in the model (Fig. 4b), a lacking effect (M) occurs, so that (R) locally disappears. This effect is stronger on the third synthetic (Fig. 4c), where the reflection (R) has almost disappeared for the benefit of diffraction patterns. This simulation shows that the lacking effect is mainly related to the presence of diffraction effects on each small cavity, so that diffusion processes makes incoherent the reflected wave front [3].
Caractéristiques du modèle numérique.
Numerical model characteristics.
Couche 1 | Milieu semi-infini | |
Épaisseur (m) | 50 | – |
Masse volumique (kg m−3) | 1300 | 1500 |
Vitesse des ondes P (m s−1) | 1300 | 3500 |
Vitesse des ondes S (m s−1) | 800 | 2100 |
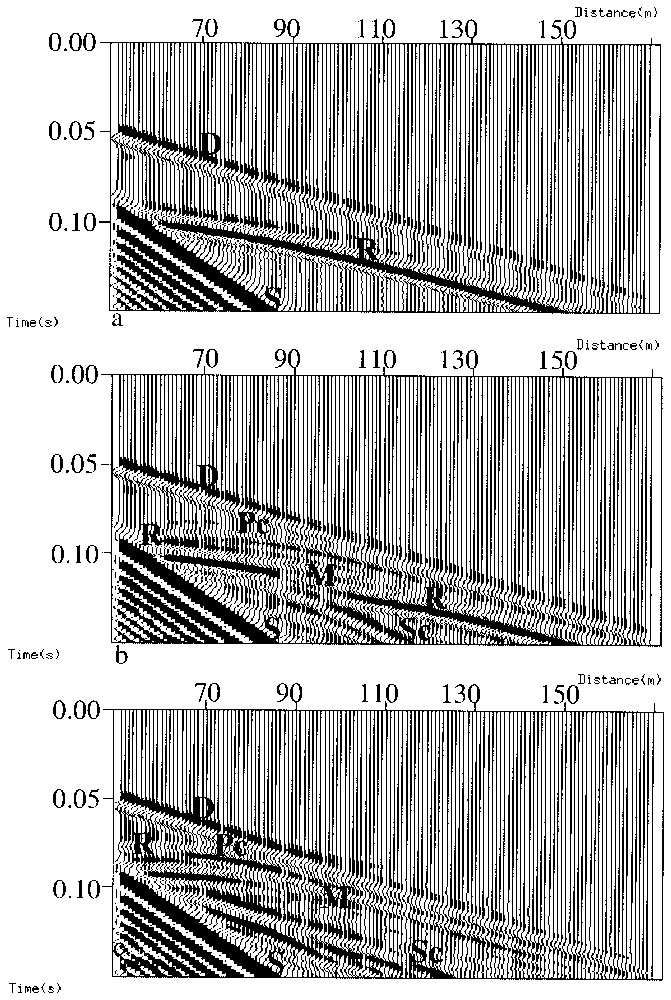
Tirs synthétiques (a) sans, (b) avec une et (c) avec trois cavités. D : onde P directe ; R : onde P réfléchie ; S : onde de surface ; Pc : onde P diffractée sur la cavité ; Sc : onde de surface diffractée sur la cavité ; M : effet de masquage.
Synthetic seismograms (a) without, (b) with one and (c) with three caves. D: direct P wave; R: reflected P wave; S: surface wave; Pc: P wave diffracted on the cave; Sc: surface wave diffracted on the cave; M: lacking effect.
1 Introduction
Dans de nombreuses régions soumises à l'exploitation industrielle ou artisanale des ressources minérales, l'existence d'anciennes carrières souterraines abandonnées constitue un risque majeur pour les aménagements et une contrainte importante dans l'occupation du sol et la gestion du sous-sol. Par exemple, la Seine-et-Marne (France) est un département français pour lequel l'impact des carrières souterraines sur l'environnement est considérable, puisque ces anciennes exploitations de gypse couvrent un domaine de plus de 10 km2, dans lequel six communes sont implantées et en voie d'expansion.
Un inventaire des carrières souterraines réalisé en 1997 par le BRGM, à la demande des ministères de l'Industrie et de l'Environnement, a permis de mettre en évidence les zones affectées par les anciennes exploitations du gypse sur un certain nombre de communes dont Annet-sur-Marne [7]. Cet inventaire, qui recense les exploitations de gypse situées dans différents niveaux exploités du Ludien, ainsi que les désordres superficiels, a été réalisé à partir des données disponibles, telles que les archives des anciennes exploitations, les informations des administrations et des associations locales. Des cartes ont donc été établies, de façon à localiser les zones à fort aléa, sachant que certaines d'entre elles n'ont pu être caractérisées, par manque d'informations. Afin de pouvoir compléter cette base de données, nous avons choisi de tester les méthodes sismiques comme outil d'auscultation non destructif. Un profil sismique a donc été réalisé au-dessus d'anciennes exploitations abandonnées, afin de connaı̂tre les performances des méthodes sismiques appliquées à la détection des cavités souterraines situées dans les 50 premiers mètres.
La sismique haute résolution (SHR) a largement été utilisée ces dernières années pour la détection des cavités souterraines [1,2,5,8]. Ces études ont montré que la présence d'une cavité pouvait être mise en évidence par l'effet de masquage qu'elle produit sur les réflexions sous-jacentes. Ce phénomène, largement décrit et démontré par Léonard [6], est d'autant plus important que les dimensions de la cavité sont grandes par rapport à la longueur d'onde et que le réflecteur est proche de la base de la cavité. Lorsque les cavités ont des géométries en chambres et piliers et que leurs dimensions sont inférieures à la longueur d'onde, le phénomène de diffraction multiple peut aussi être évoqué pour expliquer les atténuations de certaines réflexions, liées alors à de la diffusion d'onde [3]. Nous montrons dans cette étude que ce type de cavités peut être détecté par SHR, que les signatures observées sur le profil sismique sont en accord avec celles décrites par les études précédentes et qu'elles peuvent être expliquées par un effet de masquage, aggravé par de la diffraction multiple se produisant au niveau des zones exploitées en chambres et piliers.
2 Mise en œuvre
Les carrières souterraines de Seine-et-Marne résultent de l'exploitation du gypse dans les niveaux du Bartonien supérieur et du Ludien moyen et inférieur. Ceux-ci sont surmontés par les séries marneuses du Bartonien et du Ludien supérieur, puis par les séries argileuses et à meulières du Stampien. Ces séries sont horizontales ou à faible pendage vers le sud. Les carrières souterraines se situent dans le niveau gypsifère du Ludien moyen et inférieur, lui-même subdivisé en plusieurs masses situées entre 30 et 80 m de profondeur. Les niveaux intercalaires sont constitués de bancs marneux de 4 à 8 m d'épaisseur (Fig. 1a).
La première masse, qui est la plus épaisse, a une puissance qui varie de 13 à 20 m. Ce niveau a été intensément exploité, en comparaison des seconde et troisième masses, dont les épaisseurs ne dépassent pas 10 m. Les exploitations dans les différentes masses sont généralement en chambres et piliers, ceux-ci étant disposés de façon à ce que les piliers situés dans les différentes masses soient alignés et garantissent une stabilité maximum.
Le profil sismique, orienté N30, a été placé à cheval sur un domaine exploité et un domaine vierge de toute exploitation, le premier tiers du profil se situant au nord-est, en dehors de la zone d'exploitation (Fig. 1b). Sur la totalité de sa longueur (450 m), le profil traverse un champ non cultivé, ce qui nous a permis de suivre un tracé parfaitement rectiligne. Le levé topographique montre une légère variation d'altitude, dont l'amplitude maximum sur l'ensemble du profil n'excède pas 9 m.
L'acquisition fut réalisée à l'aide d'un sismomètre Geometrics de type StrataView RX 24 ayant une dynamique de 24 bits. Le dispositif était constitué de 60 géophones verticaux, de fréquence propre 14 Hz, placés à 3 m de distance les uns des autres. La source utilisée était un fusil de type SISSY à déclenchement électrique, tirant des cartouches de Dynergit (30 g), le tir se faisant au fond d'un trou dont la profondeur était en général de 70 cm. Nous avons commencé par une géométrie de type « tir en bout » en début de profil, afin de bien couvrir la partie nord. Puis, la source avançant dans le dispositif, une géométrie de type « tir au centre » a été utilisée pour la seconde moitié du profil. En conséquence, les offsets minimums ont varié suivant les tirs entre 1,5 et 3 m et les offsets maximums entre 106 et 211 m. La distance inter-tir fut fixée à 3 m et l'ensemble du profil a totalisé une centaine de tirs. L'avancement du profil a consisté à déplacer le dispositif de 36 m tous les 12 tirs, de façon à retrouver régulièrement la même géométrie d'acquisition. Le dispositif a progressé du nord-est vers le sud-ouest. Durant l'acquisition, nous avons effectué des écoutes de 1 s, enregistrées avec un pas d'échantillonnage de 0,25 ms. Compte tenu de la dynamique disponible, nous n'avons appliqué aucun filtre en fréquence au moment de l'enregistrement. Les gains furent tous fixés à 48 dB, de manière à optimiser la détection des faibles signaux.
3 Traitement
Le traitement des données a été effectué au laboratoire de géophysique de l'université de Pau et des pays de l'Adour (UMR 5831), à l'aide du logiciel Promax. Compte tenu des conditions de terrain (bruit lié au vent, bruit d'automobiles, avions, etc.), nous avons tout d'abord effectué une sévère sélection des traces : les traces issues d'un géophone défectueux ou ayant un rapport signal/bruit trop faible ont été systématiquement rejetées, surtout pour les grands offsets. Ensuite, un filtre passe-bande de 90–110–250–350 Hz a été appliqué, afin d'atténuer les ondes de surface et les signaux hautes fréquences relatifs à du bruit. Ce filtre met clairement en évidence les hyperboles de réflexion situées entre 0 et 150 ms (Fig. 2a) dans la première partie du profil. L'onde sonore, visible sur les Figs. 2a et 2b, a été atténuée par filtrage des pentes proches de 340 m s−1 (Figs. 2c et 2d). La compensation des amplitudes par égalisation trace à trace a corrigé les effets d'atténuation en fonction des offsets et du temps. Aucun AGC n'a été appliqué, en raison de la nature superficielle des cibles recherchées (moins de 100 ms). Comme le montrent les Figs. 2a et 2b, les hyperboles ainsi que la dromochronique présentent un certain nombre de rebonds, probablement dus à des effets de multiple origine superficielle. Une déconvolution a été appliquée pour réduire ces effets. Une attention particulière fut apportée au mute des réfractées, de manière à ne pas sommer des amplitudes liées à ces phases. De même, une seconde fonction mute fut automatiquement appliquée lors des corrections dynamiques, afin d'annuler les amplitudes ayant subi une trop forte distorsion. Les Figs. 2c et 2d montrent respectivement des super CDPs – combinaison de trois CDP consécutifs – utilisés lors de la sommation dans la zone vierge et exploitée.
Les hyperboles observées sur les tirs (Fig. 2a) permettent d'estimer les lois de vitesse du milieu, variant de 1200 m s−1 pour la partie superficielle jusqu'à 1600 m s−1 pour la partie inférieure. Les corrections statiques furent calculées à partir du pointé des premières arrivées, en intégrant les variations topographiques mesurées lors de l'acquisition. Nous avons ainsi calculé un modèle de vitesse pour la zone altérées, ainsi que la variation de l'épaisseur de cette couche.
La mise en collection nous a conduits à définir 320 CDP, le taux de couverture variant entre 18 et 24. Les corrections dynamiques ont été déterminées en se basant sur des sections sommées à vitesse constante, tout en contrôlant l'origine des signaux sommés. Le modèle de vitesse final est un modèle 1D, variant entre 1200 m s−1 en surface et 1600 m s−1 à 80 ms. Enfin, les corrections statiques résiduelles ont été calculées après application de ces corrections dynamiques. La section finale la plus satisfaisante (Fig. 3) a été obtenue en ne sommant que les traces aux offsets compris entre 20 et 90 m. Compte tenu de la géométrie quasi horizontale des réflecteurs, aucune migration n'a été effectuée.
La section présentée sur la Fig. 3 montre nettement une série de signaux très énergétiques, localisés dans les 150 premiers mètres du profil. Ces signaux, situés entre 50 et 100 ms, peuvent être associés à des réflexions qui, compte tenu des vitesses de propagation des ondes, se situeraient approximativement entre 30 et 70 m de profondeur. Nous pouvons raisonnablement supposer qu'il s'agit là de réflexions ayant eu lieu sur les diverses interfaces marnes/gypse qui constituent l'ensemble de la formation exploitée. Pour des distances le long du profil supérieures à 150 m, on n'observe plus ces signaux. D'un point de vue géographique, on aborde, à partir de ce point, le secteur des niveaux exploités. Il semble donc que la présence des cavités provoque une perturbation dans la propagation des fronts d'onde, les réflexions sur les interfaces marnes/gypse étant soumises à des interférences, du fait des diffractions des fronts d'onde sur les cavités.
Pour illustrer cette interprétation, une simulation de ce phénomène a été calculée. Celle-ci est basée sur la propagation des ondes sismiques dans un milieu élastique bidimensionnel [9]. Les cavités sont entrées dans la grille numérique par l'adjonction de limites de type surface libre, en utilisant un algorithme de décomposition de domaines [4]. Ce modèle prend en compte les interactions entre une onde élastique et la surface libre des cavités. La source, de type Ricker et de fréquence centrale 70 Hz, est placée à la cote zéro, et le dispositif d'écoute s'étale de 50 à 170 m, afin de se placer dans la fenêtre optimum du signal réfléchi. Le modèle de référence, constitué d'une couche superposée au milieu semi-infini, est décrit dans le Tableau 1. La Fig. 4 montre trois tirs synthétiques, calculés à partir du modèle de référence sans cavité (a), avec une cavité (10 m×10 m) placée de x=85 à 95 m et de z=30 à 40 m (b), et avec trois cavités (10 m×10 m), respectivement placées de x=70 à 80 m, x=85 à 95 m, x=100 à 110 m, et z=30 à 40 m (c). Avec les paramètres choisis, les cavités introduites dans le modèle de référence ont des dimensions de l'ordre d'une demi-longueur d'onde.
Le tir (a) montre différentes arrivées sismiques : le train d'onde direct (D), une réflexion (R) provenant du réflecteur situé à z=50 m, et le train d'onde de surface (S). Le tir (b) fait apparaı̂tre le masquage (M) de l'onde réfléchie (R), ainsi que les diffractions de l'onde P (Pc) et de surface (Sc) sur la cavité. On notera que ces diffractions sont rarement mises en évidence sur les tirs terrain, car leur amplitude est souvent en deçà du rapport signal sur bruit. Le tir (c), pour lequel trois cavités sont placées dans le modèle de référence, montre une accentuation des phénomènes de masquage et de diffraction, de telle sorte que la réflexion (R) est presque invisible.
Cette simulation montre que l'effet de masquage est typiquement lié, dans ce cas, à l'apparition de diffractions des ondes de volume sur les cavités. Ces phénomènes permettent une désorganisation du train d'onde incident – ou diffusion de volume [3] – qui rend les réflexions sur les réflecteurs plus profonds incohérentes et marque ainsi la présence des cavités.
4 Conclusion
Une expérience de sismique a été réalisée à Annet-sur-Marne, en vue de connaı̂tre les performances de cette méthode appliquée à la détection des cavités souterraines et à la caractérisation du sous-sol en présence de cavités. Dans le cas d'Annet-sur-Marne, ces cavités sont issues de l'exploitation du gypse et s'organisent en chambre et piliers, sur trois niveaux situés entre 30 et 70 m de profondeur. Une campagne de sismique haute résolution a été mise en œuvre, de façon à ce que le profil couvre partiellement la zone exploitée. Un traitement conventionnel de sismique réflexion a ensuite été appliqué aux données. La sismique réflexion nous apporte une information sur la localisation des réseaux de cavités, dans la mesure où celles-ci, de par leur effet diffractant, masquent les réflecteurs situés au-dessous d'elles et présents dans les couches de gypse exploitées. Cette interprétation a été validée par une simulation numérique pour laquelle des cavités ont été introduites dans un modèle de sous-sol. En analysant un tir synthétique, il apparaı̂t que ce sont les interactions ondes–cavités qui altèrent les réflexions se produisant sur les couches plus profondes. Cette altération, minime en présence d'une cavité, participe par construction à l'effet de masquage plus important observé sur la section sommée, lorsqu'une d'une série de cavités est considérée.
En conclusion, nous confirmons que dans le contexte d'Annet-sur-Marne, la sismique réflexion haute résolution peut être un outil géophysique adapté à la détection des réseaux de cavités depuis la surface, bien que le manque de résolution ne permette pas à cette méthode de distinguer le type d'exploitation et l'état des zones exploitées, suivant qu'elles sont en place ou dépilées.
Remerciements
Les auteurs remercient les communes et les propriétaires du lieu où les mesures ont été effectuées, J. Virieux pour avoir mis à leur disposition son code numérique de différences finies. Ce travail constitue la contribution BRGM n∘53.