Version abrégée
1 Introduction
Les minéraux, les substances divisées (argiles) ou ultra-divisées (oxy-hydroxydes) présents dans la partie supérieure de l'écorce terrestre jouent un rôle considérable dans le transport des éléments, à cause de leur capacité de rétention et de leur affinité sélective pour les solutés présents dans les eaux. Leur étude est donc nécessaire pour comprendre de manière approfondie les mécanismes d'échange ou de rétention et pour découvrir les lois qui fixent les relations des substances naturelles avec leur milieu ambiant, afin de donner aux responsables des outils d'aide à la décision pour une meilleure gestion, une meilleure protection de notre environnement, tout particulièrement des eaux et des sols, dans le cadre d'un développement durable.
Le travail est immense, dans la mesure où les milieux naturels sont très complexes et d'une grande hétérogénéité, quelle que soit l'échelle d'observation choisie. La démarche la plus sûre consiste à rechercher, d'une part, les propriétés de la phase solide et, d'autre part, les propriétés des solutions.
Les propriétés physico-chimiques des éléments les plus fréquemment rencontrés dans les eaux naturelles sont connues. En ce qui concerne les molécules organiques, tout particulièrement les acides humiques ou fulviques, qui jouent un rôle clé dans la formation des sols et des colloı̈des organo-minéraux, compte tenu de leur capacité de rétention et d'agrégation, les connaissances sont plus rudimentaires. On a choisi de présenter dans le détail les mécanismes de rétention des tensioactifs sur les solides, compte tenu : (1) de leur composition (une chaı̂ne aliphatique dont on peut régler l'hydrophobicité) et une tête polaire hydrophile de nature très variable (cationique, anionique, neutre) – connaı̂tre leur comportement comme celui des polymères est utile à une meilleure compréhension du comportement des matières organiques naturelles – ; (2) de leur physico-chimie très complexe et variée, mais connue [13,17,23] – ils donnent naissance à des agrégats de formes variables – ; (3) de leur très grande affinité pour les solides – ce sont d'excellents traceurs de l'hétérogénéité énergétique des surfaces [12] ; (4) de leurs usages industriels. Peu d'industries se passent de l'utilisation de ces agents de surface. On décrira plus particulièrement deux applications importantes pour nos économies, touchant aux ressources minérales et énergétiques, respectivement la valorisation des minerais par flottation sélective et la récupération assistée de pétrole par voie tertiaire.
La connaissance des propriétés de la phase solide avant et après immersion dans la phase aqueuse est nécessaire pour comprendre les phénomènes de rétention. Elle passe par plusieurs étapes obligées et l'utilisation de nombreuses techniques expérimentales. La méthode proposée (Fig. 1), mise en œuvre depuis plusieurs années dans notre laboratoire, permet aussi, pas à pas et au fur et à mesure de la caractérisation des propriétés structurales, texturales et superficielles de la phase solide, de mieux valoriser les substances minérales naturelles, afin de les utiliser le plus rationnellement possible dans de nombreuses branches de l'industrie. Il faut noter que chacun des thèmes trouve une application dans le domaine de la gestion de la planète, et tout particulièrement dans les problèmes d'environnement. Cette approche n'est souvent pas celle des chimistes ou des physiciens, pour lesquels l'hétérogénéité des systèmes naturels à différentes échelles peut être sous-estimée, sinon ignorée.
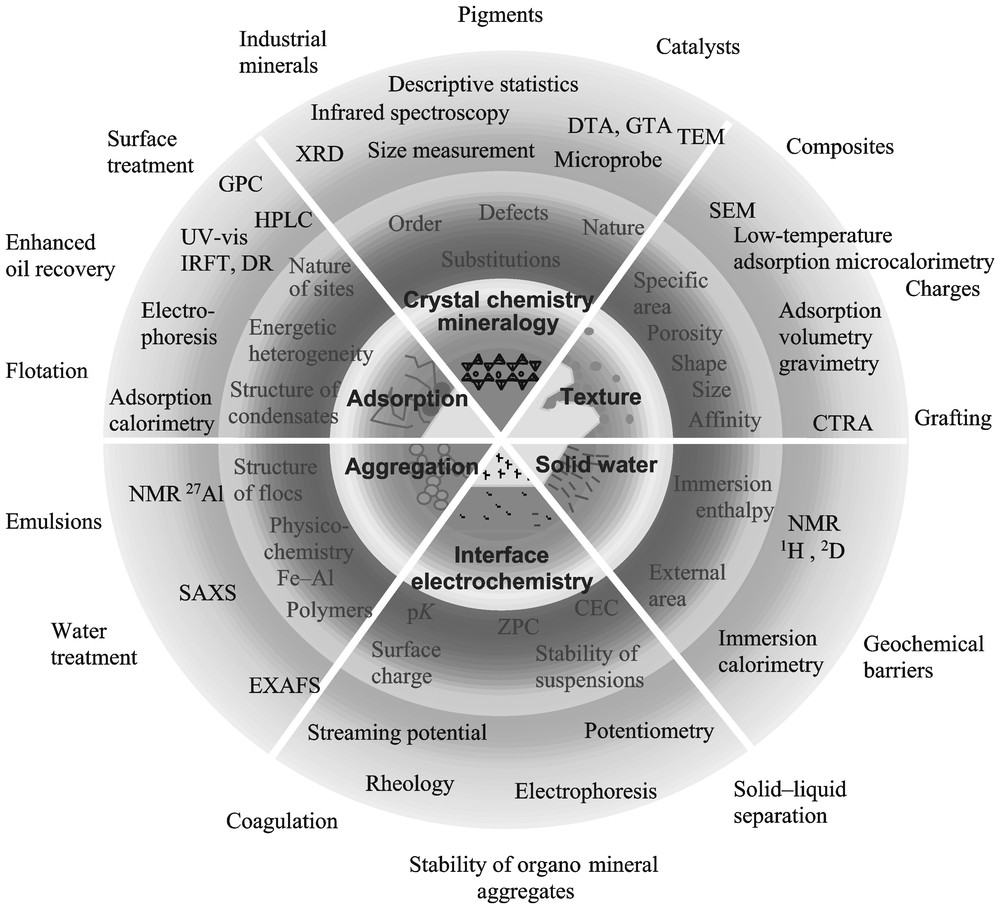
Minerals and divided solids: methodology of studies for beneficiation and the understanding of retention phenomena. The main research topics are presented in the central disk, whereas peripheral rings relate successively to (1) the main parameters measured; (2) the experimental techniques used; (3) the potential applications.
Minéraux naturels et/ou divisés : méthodologie d'étude pour une meilleure compréhension des phénomènes de rétention et une meilleure valorisation. Les thèmes de recherche sont présentés dans le rond central. On trouve successivement, dans les anneaux périphériques : (1) les principaux paramètres mesurés ; (2) les méthodes utilisées ; (3) les applications possibles.
2 Minéralogie systématique et cristallochimie
Il s'agit d'acquérir une connaissance précise de la composition minéralogique, tant qualitative que quantitative, des échantillons, mais aussi des propriétés cristallochimiques des phases associées, indispensables pour l'interprétation des phénomènes de rétention (Fig. 1, secteur 1) [45]. Dans d'autres domaines d'application, tels ceux de la conception et de la formulation des matières composites (destinées aux céramiques, aux cosmétiques et substances d'enduction – peintures, vernis, mastics, enduits –, aux papiers, aux plastiques, etc.), l'utilisation simple des données ainsi recueillies est d'une grande richesse d'application. Certaines propriétés de composition et de structure vont induire les propriétés de comportement, d'autres les conditions de genèse. En effet, en ajoutant des données technologiques à la description obtenue, on peut alors fonder un classement par aptitude, qu'on essaie d'effectuer à l'aide d'un nombre minimal de critères, aussi simple que possible à mesurer. Il est alors possible de déterminer quelle conjonction de grandeurs descriptives et de conditions préparatives engendrent les meilleurs résultats. Il faut alors effectuer des classements, à l'aide de caractères facilement lisibles, afin d'établir des typologies par propriétés d'usage. Il faut noter que les classements par type sont le plus souvent identiques aux classements par conditions de genèse ou, à une échelle plus fine, aux classements par faciès et, dans les cas favorables, au classement stratigraphique dans chaque faciès.
3 Propriétés texturales et superficielles des phases solides
Cette deuxième étape consiste à déterminer l'hétérogénéité des surfaces minérales par l'étude les propriétés extensives et intensives de la phase solide avant immersion dans la phase aqueuse (Fig. 1, secteur 2) [44]. Sur une phase argileuse, il s'agit, par exemple, de caractériser les paramètres indispensables à une bonne interprétation des isothermes d'adsorption. Il s'agit, d'une part, de la surface spécifique, du facteur de forme des particules élémentaires, des différents types de porosité, s'il y a lieu et, d'autre part, de la nature des différentes faces, de leur composition chimique ainsi que de la fonction de distribution énergétique des différents types de sites ou domaines d'adsorption. Outre certaines méthodes spectroscopiques (ESCA par exemple), on utilise principalement les méthodes modernes de volumétrie d'adsorption de gaz très basse pression (argon), dont il est possible de modéliser les résultats. Ceux-ci peuvent être corrélés à ceux obtenus par une méthode plus lourde d'utilisation, la calorimétrie d'adsorption basse température. Les applications sont nombreuses, de la modélisation des phénomènes naturels, comme l'oxydation catalytique des gaz à effet de serre dans la haute atmosphère par les aérosols et les cendres volcaniques, à la mise au point de nouveaux géomatériaux pour un meilleur confinement des pollutions industrielles ou, par exemple, la conception de bétons de poudre réactive (BPR) à haute performance.
4 Structure de l'eau au voisinage des interfaces
L'immersion d'un solide hydrophile dans une phase aqueuse provoque deux types de phénomènes : d'une part, l'adsorption et la condensation des molécules d'eau à l'interface solide–solution aqueuse (Fig. 1, secteur 3) et, d'autre part, la formation d'une charge superficielle variable en fonction du pH des solutions (Fig. 1, secteur 4) [29].
Les recherches (couplage de la microcalorimétrie d'immersion dans l'eau sous pré-recouvrement en eau contrôlé à la RMN du proton et du deutéron) mettent en évidence l'existence, sur tout solide, de deux à trois couches d'eau structurée [9,20,36]. Deux conséquences importantes peuvent en être tirées, rarement prises en compte dans la littérature : (1) aucune méthode ne permet de connaı̂tre, à l'interface solide–solution aqueuse, l'énergie d'adsorption d'un adsorbat, si ce dernier déplace ou modifie la couche d'eau structurée ; (2) l'épaisseur de la couche d'eau liée (environ 10 Å) fixe, sauf dans le cas de l'adsorption de polymères formant des boucles, le plan de coupure hydrodynamique en cas de déplacement de la phase aqueuse par rapport à la phase solide.
5 Propriétés électrochimiques des interfaces
Lorsqu'un solide est en contact avec des molécules d'eau sous forme liquide ou gazeuse, le champ généré par la coordinance libre des atomes de surface provoque la dissociation des molécules d'eau et la formation de groupes hydroxiles amphotères (Fig. 1, secteur 4) [43]. Lors de l'immersion dans une phase aqueuse, ces groupes se dissocient suivant les conditions de pH du milieu. Une charge électrique superficielle variable apparaı̂t saturée par des ions de signes contraires, fixés sur la surface ou situés à proximité immédiate de celle-ci. Ces phénomènes, présents à la surface des minéraux, que les charges soient permanentes, c'est-à-dire dues à des substitutions isomorphiques dans le réseau (cas principalement de certaines argiles et des micas par exemple), ou variables, comme on l'a présenté ci-dessus, sont responsables de l'adsorption des espèces ioniques de signes contraires lorsque la liaison normale adsorbat–adsorbant est de nature entièrement électrostatique (le cas le plus fréquent) [8]. Au voisinage du point de charge nulle, les molécules organiques riches en radicaux OH, susceptibles de former des liaisons hydrogène avec les groupes oxo ou hydroxo superficiels, s'adsorbent préférentiellement [13,18,19,23].
6 Les phénomènes de coagulation et floculation
La charge superficielle des minéraux divisés est aussi à l'origine des phénomènes qui conditionnent l'état dispersé ou coagulé (au point de charge nulle) des suspensions (Fig. 1, secteur 5). Les états ultra-divisés se comportent comme des adsorbants dont l'affinité avec les métaux lourds est très importante [24,25]. Les oxy-hydroxydes d'aluminium [3,4] et de fer [22] sont aussi très utilisés comme floculants dans le traitement des eaux de distribution et résiduaires. Leur efficacité dépend des espèces formées au sein des solutions mères, juste avant le seuil de gélation [41].
7 Les phénomènes d'adsorption, d'échange et de rétention
Les propriétés superficielles du solide étant connues, il est possible d'aborder avec efficacité l'étude des phénomènes de rétention. Les adsorbats pris comme exemple sont des tensioactifs ioniques et non ioniques, utilisés respectivement dans les domaines de la valorisation des minerais non métalliques par flottation sélective et la récupération assistée du pétrole (RAP) par voie chimique.
Les tensioactifs ioniques présentent une forte affinité pour les surfaces minérales dont la charge superficielle est opposée à celle de leur tête polaire. Ils se fixent donc sur les surfaces, à très faible concentration, par les têtes polaires, les chaı̂nes aliphatiques étant rejetées vers la solution, ce qui représente pour la molécule une forte contrainte. Sous l'influence de forces latérales importantes entre les chaı̂nes, ils se condensent sur la surface pour former des agrégats lamellaires à une couche dont la taille est fonction de l'étendue des différents domaines énergétiques de la surface. La surface devient de plus en plus hydrophobe lorsque la concentration croı̂t et que la phase minérale devient flottable. À plus forte valeur de la concentration, la condensation bidimensionnelle d'une deuxième couche sous l'influence des liaisons normales queue–queue rend de nouveau la surface hydrophile [13].
Lorsque la liaison normale adsorbat–adsorbant est faible (cas des tensioactifs ioniques utilisés en RAP dans les milieux gréseux ou des tensioactifs non ioniques), le processus d'agrégation est différent, la surface assure la fixation du tensioactif sans imposer de sévères restrictions conformationnelles. La surface du solide hydrophile joue alors le rôle de précurseur des agrégats micellaires qui apparaissent en solution au-delà de la concentration micellaire critique. La surface reste hydrophile, les têtes polaires dans les micelles étant orientées vers la solution [13,23].
Les performances de trois méthodes d'étude in situ de l'adsorption des tensioactifs sont présentées. Il s'agit de la microcalorimétrie de titration ou d'écoulement, qui permet la mesure des enthalpies de déplacement, l'ellipsométrie [18] et la spectroscopie infrarouge à transformée de Fourier couplée à la réflexion externe, qui donnent accès, sur surface lisse, à des mesures en temps réel, c'est-à-dire à l'étude de la cinétique d'adsorption. La réflexion externe a permis récemment de venir à bout d'un problème extrêmement complexe, la compréhension des mécanismes de rétention des sels d'acides gras sur un minéral à solubilité non négligeable – la fluorine [35] –, ouvrant ainsi la voie à une meilleure appréhension du comportement des acides humiques dans les eaux et les sols.
1 Introduction
Knowing the structural, textural and surface properties of natural minerals is of prime importance for understanding their genesis and equilibrium properties as well as their mechanical behaviour and alteration mechanisms. They also control to a large extent transport phenomena of dissolved species in the environment. In addition, basic research in the field of geosciences devoted to the study of the physicochemical properties of minerals whatever their size and crystallinity provides new information that can be used for answering some technological questions encountered in various sectors of the socio-economical world. Indeed, such research has proven extremely relevant in (i) the management and protection of continental water and soils, (ii) the development of enhanced oil recovery, (iii) the improvement of geochemical prospecting methods, (iv) the efficient management of natural mineral resources, (v) waste management and recycling technologies, (vi) civil engineering practice, (vii) pollutant removal procedures. It then appears clearly that this type of fundamental research can provide, in numerous fields, relevant answers for protecting the environment while promoting sustainable development strategies.
In most of the above-cited applications, a prominent role is played by retention, adsorption and exchange phenomena between natural solids and their ambient environment, which are directly linked to the surface properties of mineral phases. This is particularly true for high surface area solids such as poorly crystallised oxides or clay minerals. These mineral phases play a key role in the transport and immobilisation of chemical elements in sub-surface environments and can even be considered as ‘the living fraction’ of inorganic materials. In that context, significant progress on the characterisation of retention mechanism at the molecular level are currently obtained through the use of local spectroscopic techniques such as NMR, XANES (X-ray Absorption Near-Edge Spectroscopy), EXAFS (Extended X-ray Absorption Fine Structure) or EELS (Electron Energy Loss Spectroscopy), sometimes associated with high resolution electronic microscopy measurements. The results obtained concern the characterisation of either the adsorbing phases obtained at different hydrolytic stages, leading to the formation of oxi-hydroxides (of iron [7,26,37]) manganese [27], aluminium [4–6] or its chemical analogue, gallium [28], etc.), or the adsorbate/adsorbent couples [15,16,24,25]. The diversity of retention modes is not infinite, but strongly depends on the adsorbate/adsorbent system. In the case of poorly crystallised hydrated oxide phases, three main elementary mechanisms resulting in the immobilisation of trace elements can be observed: adsorption at the solid aqueous solution interface, formation of intimately associated phases and substitution in the crystalline network of the solid phase. For instance, it was shown that: (1) in estuarine environments, approximately 90% of lead is immobilised on hydrated manganese oxides; (2) the fixation of uranyl complexes on ferric gels is due to the formation of a bidented binuclear complex involving two UOFe chemical bonds with two distinct iron octahedra [25], the partition coefficient between the liquid and solid phase thus resulting from a perfect structural match between adsorbate and adsorbent. The high retention efficiency of such hydrated oxides is then due to two main properties: (i) a high affinity of the adsorbate for the adsorbent, (ii) elevated values of specific surface area (). At the present time, local spectroscopic experiments based on X-ray absorption techniques require the use of rather concentrated solutions ⩾10−3 M. Taking into account these constraints, EXAFS experiments have recently been successfully used to determine univocally the fixation mechanism of cobalt ions on the edge faces of hectorite [39,40] as well as the various types of chemical bonds linking Zn and Pb ions with humic acids and cell walls of fungi and lichens [38]. It then appears clearly that such research at the molecular scale can provide reliable predictions on the fixation mode and fate of heavy metal compounds in the biosphere. In opposition, they cannot be used to predict the retention of organic species at solid surfaces. In that latter case, other concepts and other investigation methods sometimes far from the molecular level have to be used. The aim of the present monograph is to describe and precise such concepts.
2 Understanding retention mechanisms on solids
The determination of experimental adsorption isotherms is the first step required for studying fixation mechanisms of adsorbates on solids. Often, the interpretation is limited by a quick analysis of isotherm shape. In the plane equilibrium concentration–adsorbed amount, most isotherms exhibit a Langmuirian shape, which does not imply that the basic hypotheses proposed by Langmuir, i.e., energetically homogeneous surface, no lateral bonds in the adsorbed layer, adsorption limited to the monolayer and non-dissociative adsorption, are fulfilled. Indeed, if the last assumption is often verified, the first one is nearly almost wrong, energetically homogeneous solids being an absolute rarity in nature. Furthermore, when the adsorbate is an organic species, lateral bonds are often present. Therefore, the observed shape (adsorbed quantity = 0 for equilibrium concentration = 0 and existence of a plateau at higher equilibrium concentration) is only related to the fact that there is no adsorption for the point of zero concentration and that there is a progressive saturation of adsorption sites for increasing equilibrium concentration, adsorption being limited to one monolayer [14]. The only relevant information is then the maximal retention capacity of the adsorbate by the solid. Such a rudimentary approach based on isotherm shape analysis therefore does not allow any detailed analysis of retention mechanisms. Indeed, a full analysis of retention mechanisms necessitates to distinguish between adsorption, exchange and three-dimensional condensation of the adsorbate on the solid support, and also to know the size and shape of the various surface aggregates that can be formed. In the case of natural substances, such a detailed analysis clearly requires to take into account the mineralogical composition of the solid phase. In addition, a complete characterisation of the surface properties of the various mineral components in the system before and after immersion in the aqueous phase must also be carried out together with a full analysis of adsorbate speciation in the aqueous phase. Such requirements can only be fulfilled through extensive research work that step-by-step provides a detailed knowledge of the solid phase and of its interaction with its environment.
3 Investigation methodology
In order to identify, understand and model the interaction mechanisms and equilibrium conditions of natural divided solids with their environment, the methodology presented in Fig. 1 can be applied. This research methodology applied in our laboratory for the past two decades takes into account the heterogeneity of natural systems and the dynamical character of interfacial systems. The main research topics are presented in the central disk, whereas peripheral rings relate successively to (1) the main parameters measured, (2) the experimental techniques used, (3) the potential applications. It is worth noting that each one of the six themes independently leads to an application in the field of geosciences and particularly in environment related problems. The presented approach is somehow different from those developed by physicists and chemists who may tend to underestimate or even sometimes neglect the multiscale heterogeneity of natural systems.
4 Quantitative mineralogy and crystal chemistry
A precise characterization, both qualitative and quantitative, of the mineralogical composition of natural substances and of the bulk properties of the various components represents a first compulsory step (Fig. 1, sector 1) [45]. Some of the mineralogical and structural properties thus determined are closely linked to some usage properties, whereas others are related to genetic conditions. For each mineralogical species, both structure and composition vary. Such variations, the study of which is the main object of crystal chemistry, obviously induce changes of all the other properties. Crystal chemistry studies are particularly relevant to the case of clay minerals where structure and composition must be studied at various scales from the atomic one (distribution of chemical elements in the layer) to the particle one (extension of the various faces) going through the mesoscopic organisation related to layer stacking. Depending on the investigated scale, multiple experimental tools (Fig. 1) can be used to determine structure and composition. The combination of chemical analyses at various scales, X-ray diffraction techniques, spectroscopic methods and statistical data treatment provides a precise knowledge of both mineralogical composition of natural samples and crystal chemistry characteristics of the various components in the system, which is essential for interpreting retention phenomena. Furthermore, in other application fields such as those related to the design and formulation of composites for ceramics, cosmetics, paints, paper, plastics etc., a simple use of the obtained data can provide very fruitful information. Indeed, by adding technological information to the set of data derived from mineralogical analyses, it is possible to define a classification scheme related to potential applications. For usefulness, such a classification scheme must be based on a few simple easily measurable parameters, which allow determining the combination of descriptive characteristics and preparative conditions leading to optimal results for a given application. Such an approach then yields a typological framework related to applications. It must be pointed out that such typological classifications are often identical to typological classifications based on genetic conditions or facies and even, in favourable cases, to stratigraphical classification inside each facies.
5 Textural properties
Studying the textural and surface properties of natural solids (Fig. 1, sector 2) represents a second compulsory step, as for analysing retention mechanisms at the solid/aqueous solution interface, it is necessary to have a proper description of the surface heterogeneity of the minerals under study before their immersion in solution [44]. Such a description includes (i) measurement of some extensive properties of the solid (total specific surface area, internal or external surface area), (ii) determination of the various types of porosity (if any) present in the sample, (iii) characterisation of some intensive properties (nature of surface sites, adsorption energy distribution). The nature of surface sites is often determined by using surface sensitive spectroscopic techniques such as XPS (X-ray Photoelectron Spectroscopy), AES (Auger Electrons Spectroscopy), FTIR (Fourier Transform Infrared Spectroscopy) associated with diffuse reflectance, etc., whereas the determination of porosity, surface area and adsorption energy distribution can be carried out using gas adsorption techniques. In that regard, high-resolution low-pressure gas adsorption associated with the DIS (Derivative Isotherm Summation) method and sometimes with low-temperature adsorption microcalorimetry allows quantifying the different faces in lamellar materials, and thus to determine the aspect ratio of particles. In the case of kaolinite, such methods have provided conclusive answers concerning the origin of the Cationic Exchange Capacity (CEC). Indeed, they have shown that, except in rare cases where dioctahedral substitutions of Al3+ by Fe2+ are present, the CEC of kaolinites is exclusively due to the edge faces of elementary particles [12]. When associated with Controlled Transformation Rate Thermal Analysis (CTRTA), high-resolution gas adsorption techniques can also be used to select the most suitable activated carbons for adsorbing organic pollutant molecules such as phenol [12]. Finally, the determination of intensive and extensive surface properties allows reaching a better understanding of mineral fillers/polymer interactions and therefore helps in the rational choice of the suitable grafting agent to provide the solid phase with desired wettability properties.
6 Water organisation at the solid/aqueous solution interface
When a hydrophilic solid is immersed in an aqueous solution, two phenomena occur [29]. On the one hand, water molecules adsorb and condense at the interface (Fig. 1, sector 3) and on the other hand, for some solids, a pH-dependent surface charge arises (Fig. 1, sector 4). By combining water adsorption gravimetric measurements and immersion enthalpy determination at increasing water precoverage, it was clearly revealed that at most 2 to 4 water layers are structured by the surface field of the solid, i.e., an approximate thickness around 10 Å [9,20,36]. This has three immediate consequences, sometimes neglected in the literature. (1) At the solid–aqueous solution interface, no experimental method yields the adsorption energy of an adsorbate if the latter displaces or modifies structured water layers. Notably, the adsorption enthalpies measured by calorimetric techniques correspond in fact to enthalpies of displacement of water by the adsorbate. (2) Except in the case of polymer adsorption when loops can extend into the solution, the thickness of structured water layers fixes the position of the shearing (hydrodynamical) plane, at which electrokinetic phenomena are measured. Structured water layers remain tightly bound to solid surfaces. (3) The presence in the solution of structure breaking or structure making ions can thoroughly modify the organisation of water layers at the interface. The structure and dynamical properties of interfacial water layers can be approached using various spectroscopic techniques (IR, Raman, NMR...) as well as scattering techniques. Numerous applications where interfacial water plays a prominent role can be found in civil engineering, geochemical barrier design, waste disposal, oil engineering, pharmaceutical and cosmetic industries. It is also crucial for understanding the swelling behaviour of homoionic swelling clays [1,10,11] and the mechanisms underlying gel formation [2]. Such a research work is rather difficult, due to the complexity of natural clay minerals that are heterogeneous and polydisperse. A combination of rheological, thermodynamical, X-ray scattering or even sometimes X-ray microscopy experiments is then necessary.
7 Electrochemical properties of interfaces
When an oxide surface is put into contact with liquid or gaseous water molecules, the field due to the free coordinence of surface atoms provokes the dissociation of water molecules and the formation of amphoteric hydroxyl groups (Fig. 1, sector 4) [43]. When the solid is immersed in an aqueous solution, these hydroxyl groups dissociate depending on the pH conditions. A variable surface electrical charge then appears. Titration potentiometric techniques and electrokinetic methods (streaming potential for coarse particles and electrophoresis for fine particles <2 μm) can be used to study the evolution of this charge with pH. For pH values lower than the PCN, the surface charge is positive and it is the negative in the opposite case. At the PCN, the surface is mainly covered with hydroxyl groups. The surface charge is compensated by ions of the opposite sign fixed at the surface or located in the vicinity of the surface. The study of the distribution of ions near the interface yields various theoretical multi-layer models. The presence of electrical surface charges either due to substitutions in the crystalline network (case of charged clay minerals and micas for instance) or to hydroxyl groups resulting from water dissociation, as presented above, explains the adsorption of ionic species of opposite charge when the normal adsorbate–adsorbent bond is mainly electrostatic in nature [8]. Around the PCN, organic molecules with numerous OH radicals that can form hydrogen bonds with the oxo or hydroxo surface groups are preferentially adsorbed [13,18,19,23]. In that pH range, colloidal suspensions coagulate, whereas they are stable for pH values corresponding to high charges (⩾15 mV). Coagulation can also be reached by surface charge neutralization. These few last sentences clearly reveal the crucial role played in natural environments by electrochemical phenomena at interfaces.
8 Coagulation–flocculation phenomena
As shown in the previous paragraph, the surface charge of finely divided solids controls to a large extent the dispersed or coagulated state of suspensions. In parallel, as discussed in the introduction section, ultradivided states such as those encountered for poorly crystallised oxi-hydroxides are very favourable for heavy metals adsorption [24,25]. For these two reasons, aluminium and iron oxi-hydroxydes are very often used as flocculants in wastewater and drinking water treatment. Indeed, in special conditions (the system being placed before the gelation threshold), the hydrolysis and condensation behaviour or Al and Fe(III) in aqueous solutions favours the formation of ultra divided non-crystalline solids that are very active flocculants. The flocculating properties of these products clearly depend on their multiscale structures [3–7,22]. These aspects will not be detailed in the present issue as a specific theme (Fig. 1, sector 5), as a synthesis of such studies was recently published in the same series [41]. The structure of flocs formed during water treatment operations is another key parameter, as it controls the water content of the sludge and, therefore, their filterability and rheological behaviour. It can be studied efficiently using scattering techniques based on light, X-ray or neutron beams. In addition, a new method was recently developed to study floc structure in situ [46]. In this approach, digitised microtome sections of an activated sludge floc were obtained and the 3-D structure of the object was reconstructed using a new interpolation method, called discrete smooth interpolation. Floc size, surface area and volume were then directly measured on the 3-D reconstructed object. Both contour and surface area exhibited a fractal nature with fractal dimensions of 1.4 and 2.4, respectively. Such a method presents numerous advantages (rapidity, automation, accuracy...) and can certainly be further developed to reach a better understanding of floc structure and of its consequences on the efficiency of water treatment procedures.
9 Adsorption at the solid–aqueous solution interface
On the basis of the information gathered in the five preceding sections, the adsorption mechanisms at the solid–solution interface can be examined in detail (Fig. 1, sector 6). In the present monograph, adsorption mechanisms will be detailed in the case of surfactant molecules, which were the main focus of the five last chapters. The reasons for such a choice are the widespread use of surfactants, their relevance to geosciences and their complexity, which clearly illustrates the need for multiple researches for understanding adsorption mechanisms.
Surfactant molecules are surface-active agents that are used in an extremely wide range of applications due to their fundamental properties: wetting power, emulsifying power, frothing, and detergency. These applications can be listed according to these fundamental properties and the order classically chosen follows the increasing complexity of the system under consideration [21]. (1) In the case of simple solutions of the surface-active agent, the micellar and solubilisation properties are exploited. Solubilisation can be either internal (inside the micelle for non polar compounds such as oil for instance) or external, outside micelles. Such situation is applied for instance to plastosoluble colouring agents for polyester dyeing and to the emulsion polymerisation of vinyl monomers. (2) Surfactant-based froths are used in froth drilling, extinguishers, low-weight plasters and cements, shampoos and foam bathes. In that case, the mechanical properties of froth depend on the properties of the surface films forming the walls of the bubbles. (3) Thanks to their emulsifying power, surfactant molecules are able to stabilise suspensions of a liquid in a non-miscible liquid. The emulsions obtained can be either oil in water (the dispersed phase is oil) or water in oil and are used in agriculture (emulsions of phytosanitary agents), food industries (creams, mayonnaise), building industries (paints), cosmetics (ointments and creams), textile industries (colouration), civil engineering (bitumen). (4) Surfactant molecules also present strong adhesion power, wetting power and dispersant properties. The adhesion properties are used in froth flotation of mineral ores, anticaking treatments of fertilizers, lubrification and crystal growth applications, where surfactant molecules are added to selectively inhibit the growth of certain crystalline faces. The wetting power of surfactant molecules, defined as the easiness with which a solution spreads on a surface is regularly used when a treatment solution is contacted with a smooth surface (phytosanitary treatment of leaves, metal surfacing, cleaning of windows etc.), or when a porous support must be treated by a solution (leather treatment, textile dyeing). The dispersing ability of surfactant solutions are used for dispersing sulphur, pigments in paints etc. (5) The most well known property of surfactants is certainly detergency. Surfactant molecules are able to detach ‘dirt’ particles from a surface and maintain them dispersed in suspension. They are then used in metal panels deoiling, and in the washing of cloth (highest consumption of surfactants), dishes, soils, bottles etc. The detergency of surfactants is also used in enhanced oil recovery and has recently been applied to soil remediation, as surfactant molecules are able to displace and remove oily and metallic contaminants from polluted soils.
As evidenced by the preceding list of applications, nearly all industrial branches use surfactants somewhere in their process. In that context, tightening environmental regulation and increasing awareness for the need to protect ecosystems have recently lead to an increasing interest in biosurfactants as possible alternatives for petroleum-based chemical surfactants. Indeed, due to their intensive use, surfactant molecules are also polluting agents that can be transported easily in the environment despite bacterial biodegradation, which is rather efficient for linear chain surfactants. Studying their adsorption mechanisms is then of prime importance.
However, before carrying out such a work, it is first necessary to know in detail the speciation and phase behaviour of the adsorbate in the aqueous solution. Such a statement is particularly well illustrated for surfactant molecules, whose phase behaviour in aqueous suspensions is very complex.
9.1 Physical chemistry of surfactants in aqueous solution
Surfactants are amphiphilic molecules and are formed with two parts, whose chemical nature is significantly different [13,17,42]. The first part, called aliphatic tail, is apolar, hydrophobic and is formed by one or two hydrocarbon chains either saturated or weakly unsaturated, either linear or branched, whose length can vary between 4 and 20 carbon atoms. The second part is polar and can be cationic (formed with NH3+ or N+(CH3)3 groups for instance), anionic (formed with COO−, SO3−, OSO3−, PO32−, OCS2− for instance) or non ionic (when the polar group is an OH group, the link with the aliphatic tail occurs through ethylene oxide units (CH2OCH2)). Some amphoteric surfactants are zwitterions: for most of them, the molecule includes an amine function bearing the positive charge and an organic or inorganic acid function bearing the negative charge.
The properties of surfactant in aqueous solutions result from a compromise between the properties of the polar and apolar parts of the molecule. Being hydrophobic in nature, the aliphatic chain will tend to concentrate towards the liquid–air interface to lower its surface tension.
Phase diagrams of surfactant molecules are always complex due to the important polymorphism of such systems. In a temperature/concentration diagram, ionic surfactants often present three main domains. (1) In the low-temperature domain, when the temperature is less than that corresponding to the Krafft point, TK, molecules are organised above a certain concentration as small crystals dispersed in water (coagel) or as extended lamellae separated by water layers and regularly stacked along one direction (gel). The chains are fully stretched and nearly all in the trans-configuration. The system is then ‘crystallised’ as the chains present a single conformation. (2) For intermediate temperatures higher than the Krafft point, and for high water contents gauche forms start appearing, which results in a rapid fluidification of the chains due to their high conformational diversity. Above a certain concentration value called Critical Micellar Concentration (CMC), surfactant monomers are in equilibrium with aggregates that are spherical at low concentration and cylindrical for higher concentrations. These aggregates, called micelles, are dispersed in the aqueous solution. The polar heads are oriented towards the solution and the aliphatic chains are located inside the aggregates, linked together by strong bonds between hydrocarbon chains. (3) For even higher temperatures and for low water contents, chains agglomerate to form infinite aggregates linked together by long distance interactions (lyotropic phases) in one direction (lamellar phases of bilayers), two directions (compact hexagonal phase formed by the association of infinitely long cylinders) or three directions (cubic phase) [17]. The solubilisation behaviour of surfactant molecules depends on their phase behaviour and the relationships between those two aspects will be examined in detail in the present monograph [42]. It is also clear that the complex phase behaviour briefly described here must be taken into account when trying to unravel surfactant adsorption mechanisms on a solid surface.
9.2 Surfactant adsorption at the solid/aqueous solution interface
Surfactant adsorption at solid surface will be analysed for both ionic species [13] and non-ionic ones [23]. In the presence of a hydrophilic solid, the polar head of ionic surfactants will form strong bonds, mainly electrostatic, with the surface (systems with strong normal adsorbate–adsorbent bonds). In that case, the aliphatic chains are extended towards the solution, thus rendering the solid hydrophobic [13]. In the case of non-ionic surfactants, the bond with the surface is weak, which leads to the formation of surface aggregates, with their polar parts oriented towards the solution. In that case, the solid surface remains hydrophilic [23]. Two main applications are considered in that case: froth flotation where ionic surfactants are used and enhanced oil recovery where both ionic and non-ionic surfactants can be utilised.
Without the development of froth flotation, there would be no mining industry as we know it today. Nearly the whole world supply of various metals (copper, lead, zinc, silver, manganese, molybdenum, chromium, cobalt...) directly depends on froth flotation operations. In that regard, the development of flotation processes is of the same importance as that of smelting, both processes explaining the past increase in mineral production. In addition, improvements in flotation technology have allowed the mining and beneficiation of low-grade complex ores. Nowadays, whereas the mining industry for metallic ores faces a rather severe crisis due to declining ore grades, tighter environmental regulations and development of substitution materials, the development of froth flotation processes for non-metallic and industrial minerals is spectacular. Enormous amounts of phosphate minerals (used for fertilizers and detergent productions) and of other industrial minerals (muscovite, fluorite, feldspar, beryl, baryte, spodumene, sylvinite, fine coal...) are indeed recovered using flotation techniques (in fact more than one hundred metallic and non metallic minerals). Regarding the importance of these applications and the use of flotation techniques in the chemical, paper and food industry, improvement in surfactant design and flotation processing still represents a major industrial issue.
In January 2001, the proven world reserves of crude oil were estimated at 141 billion tons, i.e. the equivalent of a 42-years supply, considering the present oil consumption. Every year, this figure tends to increase as: (1) new oilfields are discovered; (2) when discovered, the reserves of a given field are often underestimated as they do not take into account future enhancement in extraction procedures; (3) previously unprofitable oilfields can now be exploited (for instance deep off-shore, arctic zones, asphalt sands, bitumen, ultra-heavy oils etc...); (4) technological progress in both exploration (3-D seismic for instance) and exploitation (horizontal drilling, fracture drilling, enhanced oil recovery...) procedures are constantly implemented. In fact, primary recovery rates are on average less than 30%. One can foresee that rates around 40–50% should be reached in the near future, especially for oil with high density and low viscosity. Among the currently investigated procedures, the use of surfactant (either chemically-based or bio surfactant) for increasing recovery rates is one of the most extensively studied. When oil is present mainly in sandstones, and when the composition of solution is compatible (medium ionic strength and low content in divalent species), anionic surfactants are the first choice as the negative surface charge of quartz particles minimizes surfactant loss through adsorption on the reservoir rocks. The retention mechanisms in that case have been described by Cases et al. [13]. For other types of reservoir rocks, the use of non-ionic surfactants [23] is generally preferred.
9.3 Specific investigation techniques
Retention mechanisms can be approached by combining (i) a thorough analysis of adsorption isotherms obtained from either the rest methods or, for low specific surface area samples, inverse chromatography techniques and (ii) spectroscopic investigations allowing to study the organisation of adsorbed molecules. In recent years, three in situ analytical methods that will be presented in this monograph [18,35] have provided new information, increasing our understanding of surfactant adsorption at solid surfaces. (i) The measurement of displacement enthalpies accompanying surfactant adsorption can now be obtained using microcalorimetic methods (either titration calorimetry or flux calorimetry). It provides the building step of the surfactant-adsorbed layer [18]. (ii) In real time, the evolution upon increasing surfactant adsorption of the thickness of the adsorbed layers can be approached in the case of flat smooth surfaces using ellipsometric techniques [18]. (iii) The organisation of the adsorbed layers and its evolution with surfactant concentration and/or time can be analysed using Fourier transform infrared spectroscopy coupled with external reflection techniques [35]. That latter method has provided a precise description of retention mechanisms of fatty acid salts at fluorine surfaces and kinetic studies on such soluble minerals have given rational explanations for the long conditioning times classically used in fluorine flotation processes [35]. In addition, using such a spectroscopic approach, spectacular results concerning the selective flotation of complex sulphide ores of the South-Iberian peninsula (Neves–Corvo orebodies) by xanthate collectors have been obtained. In particular, chalcopyrite concentrates have been purified by removing sulfoarsenides (tennantite) and sulfoantimonide (tetraedrite) compounds, whose environmental impact was detrimental to the use of the concentrates during pyrometallurgical operations [30–34].
10 Conclusions
At a time of increasing environmental awareness, Earth Sciences disciplines are to play a major role as they study several key issues for environmental protection (climate change, natural risk assessment, mineral and energy resources exploitation, soil remediation, water treatment). As will be shown in the present monograph, in order to help in decision-making processes, much fundamental research has to be implemented to reach a clear understanding of natural processes such as the transport and retention of chemical elements in subsurface environments. The following publications try to present a rigorous scientific approach using multiple concepts and experimental techniques, that can be applied to understand and sometimes solve some of the complex problems encountered in environmental science. They also reveal that the field of applications of such research is not necessarily limited to the case of geosciences only. Finally, in all the presented work, the multi-scale heterogeneity of natural systems appears as a crucial parameter that must always be considered for obtaining significant scientific advances.
Acknowledgements
The author wishes to thank Laurent J. Michot for helpful suggestions and additions to this manuscript. The researchs described in this issue were performed with the help of the Centre National de la Recherche Scientifique (especially SDU department). This special issue is dedicated to Professor D.W. Fuerstenau (Berkeley University) for his outstanding contribution to mineral processing and flotation and his for 75th birthday.