Version française abrégée
Les enregistrements d'inversions dans des séquences volcaniques procurent sans doute les données les plus fiables sur ces événements rapides. Malheureusement, peu d'études ont pu être effectuées jusqu'à présent avec une haute résolution. Nous présentons une compilation des cinq enregistrements les plus détaillés dont les âges sont compris entre 1 et 15 Ma. Ils ont été obtenus principalement à partir de sites du secteur Amérique–Pacifique ouest. Un seul enregistrement provient d'Islande. Nous reportons d'abord les trajectoires suivies par le pôle géomagnétique virtuel (PGV) lors de chaque inversion ainsi que l'évolution de la latitude du pôle en fonction de l'ordre stratigraphique dans la séquence. Les transitions sont complexes avec des oscillations de grande amplitude, qui suivent un basculement rapide du pôle d'un hémisphère à l'autre. L'allure des courbes rappelle celle des enregistrements de paléovariations séculaires et est compatible avec l'existence d'un champ non-dipôle complexe lors des inversions. On observe également une alternance entre des variations en apparence très rapides et des groupements de PGVs sensiblement dans la même position géographique. Nous discutons pourquoi l'origine de ces amas de PGVs, présents à toutes les longitudes, est probablement liée à la chronologie des éruptions volcaniques (alternances d'activité intense et de quiescence), plutôt qu'à des épisodes de stabilité du champ pendant les inversions. Nous testons également l'hypothèse de bandes de longitudes préférentielles au-dessus de l'Amérique et de l'Asie, suivies par le pôle géomagnétique virtuel (PGV). Les bandes sont présentes malgré une dispersion importante, mais il existe un troisième secteur au-dessus de l'Europe qui invalide la suggestion d'un lien possible entre la structure du manteau profond et la géométrie du champ transitionnel. Dans un deuxième temps, nous avons incorporé des données volcaniques moins détaillées. Les PGVs de l'ensemble de la base présentent une répartition semblable, avec trois bandes de longitude qui rappellent la distribution des anomalies d'inclinaison déduites des modèles d'inversion du champ pour les cinq derniers millions d'années. Toutefois, le faible nombre d'enregistrements d'inversions, associé à la mauvaise répartition géographique des sites dans les deux bases de données (inversions et variation séculaire) rend toute interprétation très hasardeuse. Plusieurs enregistrements de la dernière inversion ont été extraits de la base de données. L'ensemble est caractérisé par une dominance de PGVs dans l'hémisphère sud, qui suggère que la première phase de cette inversion serait plus longue que la seconde. Les enregistrements de paléointensité, qui suggèrent une phase de décroissance très longue du dipôle avant l'inversion par opposition au regain très rapide qui suit, sont évidemment compatibles avec cette observation.
1 Introduction
Paleomagnetic data are the unique source of observations to understand the geomagnetic transitions and hence the dynamo processes involved in field generation. However, because reversals occur over a few thousand years at most, it is a real challenge to acquire detailed paleomagnetic information about the transitional field. The first reversal records were obtained from volcanic sequences, but the development of very sensitive magnetometers favored a quest for transitional directions in sediments rather than in volcanics. A large number of records were acquired within a few years and this indeed was seen as a promising improvement. Several simple concepts such as the dipolar character of the transitional field [10,11] and then a possible dominance of axisymmetrical components [18] could be tested [12,29]. The first compilations [8,24] of sedimentary paleomagnetic data suggested that reversals exhibit some regularity with virtual geomagnetic pole (VGP) positions preferably lying within the American and Asian longitudinal sectors. In the meantime, it was noticed that these two bands coincide with lower mantle heterogeneities [24] and could thus induce persistence pattern in core flow and hence in features of the magnetic field. The ‘preferred paths’ generated some controversy that relied on the site distribution [44], the fact that some high-resolution records dominated over the rest of the database and above all that the VGP paths appeared also to lie 90° away from their respective site longitude [27,44]. This last tendency was difficult to reconcile with geomagnetic arguments and several possibilities [2,25,36] have been proposed which question the reliability of sediments as adequate field recorders during periods of low field intensity [33,34]. No clear answer has been given yet to this difficult question. The response of sediment magnetization to field changes involves a combination of complex physical and magnetic parameters.
Another important issue regarding the existence of preferred bands of longitude was the assertion [31] that intermediate VGPs derived from volcanic lava flows were randomly distributed. In this case also, disputing arguments were recently opposed by Love [26], who found that volcanic data give intermediate VGPs that tend to fall along the American and Asian longitudes that are roughly consistent with the sedimentary data. The aim of the present paper is to bring up some new views on this question but also to reconsider the current way of interpreting reversal records by statistical approaches, which to some extent do not tell us much about field morphology and mechanisms involved in the transitions.
2 Guidelines and justifications for data selection
As noticed above, potential problems inherent to the use of sediments for reversal records have not found yet any clear answer. Because lava flows do not introduce any significant delay in field recording, they appear to be much more suitable to properly document the reversal process' rapidity. However, it is important to put forward a few consequences of volcanic processes on the paleomagnetic records. Volcanism is sporadic in nature and sequences of lava flows are very irregularly distributed in time. There is no way to control the timing of successive eruptions, as error bars on the ages (in the over-optimistic case of a uniformly dated sequence) exceed the entire duration of the reversal. For these reasons, two conflicting approaches are employed when interpreting the vector changes during reversals and paleosecular variation. The first one considers that similar paleomagnetic directions recorded by successive flows represent a unique picture of the field over a very short period of time and should thus be averaged, while the opposite view defends that each individual lava flow provides a unique and independent record of the local field. Prévot et al. [31] used the first concept, while Love [26] treated each individual data separately, arguing that this could obliterate preferred VGP longitudes. This question is important as it is also directly related to the origin of VGP clusters that Hoffman [20] considered as indications of transitional dipolar states. Indeed, the presence of successive flows with the same direction can either be caused by rapid eruptions or represent an actual standstill in the reversal process. We thus oppose the ‘volcanic’ hypothesis, which assumes that directional clusters result from rapid successive eruptions, to the ‘field’ hypothesis, for which the field remained stable for a long time. The rate of historic field changes varies considerably and depends on location. Declination changes in Europe by about 10° in about 80 years. There are many records for which this value can be considered as a limit of resolution. Thus redundant directions can be recorded over a few hundred years, which is indeed compatible with frequencies of volcanic eruptions.
Detailed stratigraphy of the flows is required, which points out the importance of dealing with long sequences of superimposed lava flows. Alternatively, we would not follow Prévot et al. [31] in rejecting data from different volcanoes that have the same directions, unless there is a direct correlation between the sequences. Love [26] argued that when both directions and intensities of successive flows are available, the direction can be stable, but the intensity can change by tens of microteslas, thereby indicating an actual field evolution over a rather long time period, given the rate of change of the dipole. However, it is difficult to envisage that a predominantly dipolar field (with high intensity) would immediately follow (or precede) a non-dipolar field without significant change in direction. Also, the paleointensity data must be considered with their error bars. For many reasons [40], error in determinations of absolute paleointensity from basaltic rocks is not lower than 20% and sometimes higher. Because paleointensity experiments are so time consuming, published values rely frequently on three samples per lava flow, which is not enough to average out heterogeneities of the rock [42] and experimental uncertainties. In addition, several studies were performed without using proper checks aimed at detecting magnetomineralogical changes produced during heating experiments. In these cases, deviations between consecutive flows with similar directions could simply reflect inaccuracies inherent to paleointensity experiments and/or to complex magnetization of rocks. A typical illustration that stable direction between two flows does not coincide with stable paleointensities can be found in a detailed record of absolute paleointensity across the last reversal at La Palma Island [46].
A direct way of testing the ‘volcanic’ with respect to the ‘field’ hypothesis is to deal with parallel records of the same reversal. The Waianae sequence of the Oahu Island (Hawaii) offers an exceptional opportunity to compare three adjacent records of the Lower Mammoth reversal from sections that are separated by a few kilometers at most [16]. The stratigraphy and geology of the flows has been extensively described [39]. The changes in VGP latitudes (Fig. 1) depict the same pattern of variations in each section, but the successions of the individual VGPs are different. Clusters can be recorded in one or two sections but may be completely absent in the third section. Similarly, the absence of VGP in two sections could be interpreted as indicative of rapid field changes but this hypothesis is discarded by a regular evolution of the vector in the third section. This suggests that alternation of apparent steadiness with episodes of apparent rapidity results from the timing of eruptions and therefore that clusters of directions are most likely explained by the ‘volcanic’ hypothesis. This interpretation implies that there is no reason to find a dominance of clusters within the preferred longitudinal bands. It is thus important to determine whether clusters are dominantly found within the American and Asian preferred longitudes.

Latitude of the virtual geomagnetic pole (VGP) during the Lower Mammoth transition recorded in three parallel sections of the Waianae sequence (Oahu Island, Hawaii) (redrawn from [46] with VGPs instead of inclination). Each record displays the same pattern of variations, but different successions of directions have been recorded in each section. This demonstrates that the timing of eruptions is the dominant factor that controls the appearance of clusters or the absence of transitional data.
Latitude des pôles géomagnétiques virtuels (PGV) lors de la transition « Mammoth » inférieure, enregistrée dans trois sections parallèles de la séquence Waianae (ı̂le d'Oahu, Hawaii).
An implicit statement is that reversal records should not be considered if they do not display long records of full normal and reverse directions. The reversal test is a basic test in paleomagnetism and consequently it should be even more specifically applied in the present situation, otherwise we would be dealing with directions that cannot be identified as suitable transitional directions. In addition, the instantaneous character of the magnetization process provides records of the full vector and thus important information about paleosecular variation. It is also crucial to ascertain the coherency of the sequence and the actual origin of the transitional directions. Several effects including overlapping of non-antipodal directions [28] resulting, e.g., from unremoved viscous components [13], residual remagnetization caused by partially removed lightning components and/or chemical remagnetization [45] could produce artificial ‘intermediate’ directions. For all these reasons, we consider that single or even multiple ‘intermediate’ directions recorded in geographically sparse lava flows cannot be validated. This, in a way, is more restrictive than the previous analyses that were dealing with stratigraphic sections, but not necessarily with clear records of the periods that preceded and followed transitions. It is surprising that this requirement, which is obvious when dealing with sediments, is not applied for volcanics. Lastly, we note that for the same reasons, complexity and inaccuracies are introduced when dealing with excursions, especially when they are not recorded within volcanic sequences that are long enough to show records of paleosecular variation. Note that excursions actually represent the large majority of records in Love's database [26]. Including excursions means a priori postulating that they are linked to the same processes as field reversals. This is probably correct but not proved.
The number of transitional VGPs can vary considerably between reversals. For this reason, it is convenient to weight the records by the total number of transitional VGP positions. This approach was applied to sedimentary records [44], because detailed records can incorporate hundreds of transitional directions whereas low-resolution records are characterized by no more than a few data points. The situation is different for volcanics, which so far never exceeded 15–20 independent transitional directions. Very little information can be obtained from records with a few transitional directions. We will see below that the most detailed records do not show longitudinal concentrations of VGPs but rather a complex structure. Thus it is not justified to give the same weight to poor-resolution records as to detailed records that contain important information. The matter is also complicated by the fact that directional clusters can be given a very different weight depending on their interpretation. Despite these considerations, we will check the stability of the VGP distributions before and after weighting.
For all these reasons, we feel that it is essential to primarily rely on the most detailed records of reversals. One can consider this choice as too restrictive, because it imposes strict rejection criteria that reduce the database to a few records. However this is, to our eyes, the most reliable approach to extract global characteristics of the reversing field from volcanics.
3 Category-A volcanic records of reversals
We have selected records obtained from unambiguous and clearly established stratigraphic successions of lava flows with at least eight intermediate and different VGP positions between 60°S and 60°N (this number was selected because it really marks the lower limit for this category of records with respect to all others that do not incorporate more than three different VGP positions) and with full normal and reverse directions. Only five detailed reversal records [7,17,30] satisfy these criteria that we consider as basic requirements to extract reliable information. We restrained the Icelandic dataset to the record from profile C [47], which was recently revisited [17] and which to our knowledge is the best-documented record obtained so far from a single sequence.
The five VGP paths are shown in Fig. 2. The first overall characteristic is the apparent complex structure of the trajectories with large longitudinal and also latitudinal crossings. Some trajectories depict appearance of loops. Another picture of the records is shown in Fig. 3 by plotting the VGP latitudes as a function of their stratigraphic order in the sequence. The apparent first stages of the transitions are frequently characterized by latitudinal crossing of VGPs from initial polarity to very high or mid-high latitudes of the opposite hemisphere. This episode is either relatively well documented or appears as a rapid transit. Since a significant period of time can be missing in each record (due to absence of eruptions), we cannot discern whether the opposite polarity has been reached. The latitudinal crossing of VGPs is frequently followed by an oscillation with amplitude depending on the temporal resolution, i.e. the timing of the eruptions. The most pronounced oscillations mark a return towards low latitudes, but without restoration of initial polarity. Their frequent presence indicates that they are characteristic of the transitional process, in contrast with a previous suggestion [43]. The succession of these oscillations reminds records of paleosecular variations with the amplitude of the waveforms being considerably amplified in the absence of strong (or any) dipole component.

Evolution of VGP latitudes as a function of stratigraphic order for the five best documented volcanic records of reversals: Upper Jaramillo from Tahiti [45], Lower Mammoth and Gilbert–Gauss from Oahu, Hawaii [46], 12.9-Ma-old record from Iceland [30] and 15.3 Ma old from Steens Mountain, Oregon [16].
Trajectoire des pôles géomagnétiques virtuels des cinq enregistrements volcaniques d'inversions les plus détaillés.

Evolution of VGP latitude as a function of stratigraphic order for the flows of the five category-A reversals. The gray curves have been obtained after smoothing by polynomial approximation. This technique can be discussed, as there is no linear distribution in time. It was only performed to compare the global patterns of large amplitude variations in all records.
Évolution de la latitude des PGV en fonction de l'ordre stratigraphique des coulées correspondant aux cinq enregistrements de la catégorie A (cf. texte).
There is no longitudinal confinement except for the Lower Mammoth [16], but the absence of VGPs in the southern hemisphere suggests that an important part of the process was not recorded. All records exhibit clusters of VGPs at various longitudes without preference for the American and Asian sectors. There are also episodes of apparent fast changes at different time intervals during the transition. Following the results of the Waianae sequences [16], it seems clear that these alternations rather reflect the chronology of eruptions. In other words, major features of the reversal process can be observed, but it is impossible to reconstruct the entire structure of the transitions. The site distribution (which is dominated by the Pacific) and all individual VGPs are shown in Fig. 4a and b, respectively. The longitudinal distribution of the VGPs (Fig. 4c) shows abundance of data in three broad longitudinal bands that roughly correspond to the eastern Pacific, Europe–Africa and western Pacific sectors. In contrast, there is almost no pole within the Atlantic and the Eurasian longitudinal sectors. Thus the two preferred bands over America and Asia are not exclusive. As expected, the distribution does not change (Fig. 4d) after weighting each data by the number of transitional VGPs inherent to the record and the latitude of the corresponding VGP. The significance of the distribution is not straightforward if we take into account the longitudinal dispersion inherent to each individual record.
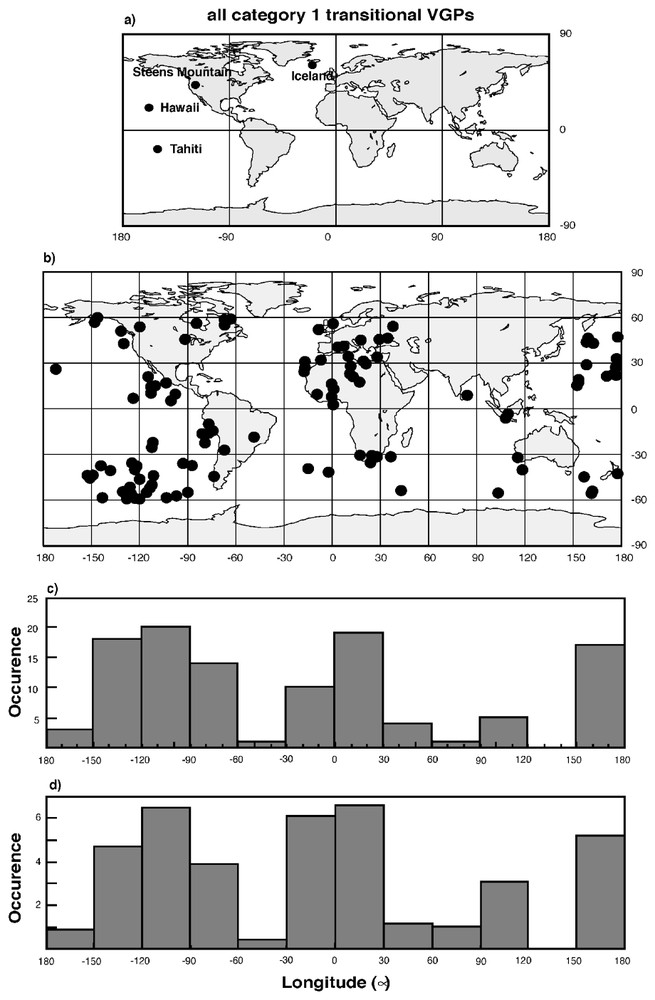
Category-A records. (a) Sites locations of the five category-A volcanic records shown in Fig. 2. (b) Transitional VGPs with latitudes lower than 60°. (c) Distribution of the VGP longitudes with latitudes <60°. (d) Same as (c) after weighing the data to take into account the latitude of the pole (λ) and the number (N) of transitional VGPs in the record (cosλ/N).
Enregistrements de catégorie A. (a) Localisation des cinq enregistrements de la catégorie A. (b) PGV transitionnels dont les latitudes sont inférieures à 60°. (c) Distribution des longitudes des PGV dont les latitudes sont inférieures à 60°. (d) Fig. 4c obtenue en normalisant les données pour tenir compte de la latitude des PGV et du nombre de données transitionnelles dans chaque enregistrement.
4 Category-B volcanic records
We have attempted to go one step further by enlarging the database to ‘category-B’ records from stratigraphic sequences with normal and reverse polarity lava flows and a minimum of five intermediate directions (but including also transitional directions that are similar). Because there are too few VGPs, it is meaningless to plot each individual path. The site distribution (Fig. 5a) incorporates records of the last reversal from Chile [5], Tahiti [7], Canary [38], and Hawaii, of lower Jaramillo from Tahiti [7], of the upper Olduvai [20] from Hawaii, Pliocene records from Hawaii [4,21] and a 8.5-Ma old Miocene record from New Zealand [19]. The distribution of VGP longitudes (Fig. 5b) is not very different from the previous distribution. However interpretation is even more delicate due to the weak number of very transitional VGPs (in fact many positions have latitudes close to 60°) and the fact that some records are characterized by directional clusters such as the last reversal (Brunhes–Matuyama) from Chile [5], which shows a unique spot of transitional VGPs over Australia (Fig. 5c).
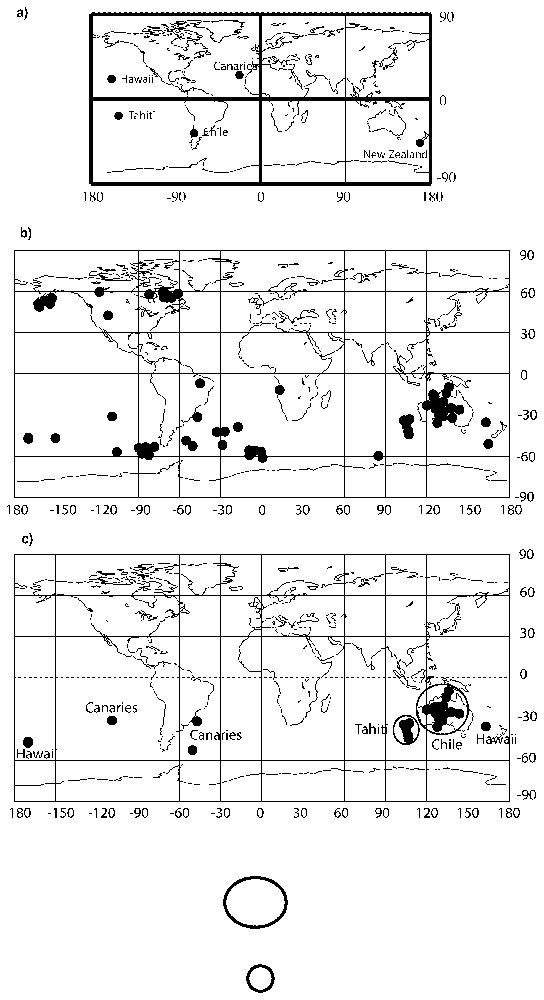
Category-B records. (a) Site locations of records. (b) Transitional VGPs with latitudes lower than 60°. (c) Transitional VGPs corresponding to volcanic records of the last reversal. The circle surrounding the VGP positions in the vicinity of Australia indicates the two clusters recorded in Chile and Tahiti.
Enregistrements de catégorie B. (a) Localisation des sites d'enregistrement. (b) Localisation des PGV transitionnels dont les latitudes sont inférieures à 60°. (c) Localisation des PGV transitionnels correspondant aux enregistrements volcaniques de la dernière inversion.
The published volcanic records of Brunhes–Matuyama were obtained from four distinct locations in Chile [5], Canary Islands [38], Tahiti [7] and Hawaii. The records from Iceland [23] were not considered as identification of the reversals and stratigraphy of the sequences is not clear. Unfortunately, the Maui record from Hawaii was published for dating purposes [1,37] without report of paleomagnetic study and therefore cannot be properly validated. Considering that the two records of this transition are characterized by different transitional directions and in the absence of detailed information, we selected the most recent dataset [37] from the Heleakala section at Maui. Two studies were recently carried out at Los Sauces in La Palma (Canary Islands). The first one [35] revealed that intermediate directions, previously not clearly related to the transition [32], belong in fact to another geomagnetic event that occurred 200 kyr earlier. The second one [38] reported three new transitional directions from another section of the Los Sauces sequence. In Fig. 5c are shown the transitional VGPs associated with these records (for comparison with other studies, we have included the results of Maui in Fig. 5b and c). The two records from Tahiti and Chile are characterized by clusters in the vicinity of Australia and no other transitional VGP. The ‘field’ hypothesis implies that the pole should span most of the transitional period in this position. However, clusters or similar VGPs should be systematically present in the other records of this transition if this episode was long enough to be associated with an inclined dipolar state. Thus we rather defend the ‘volcanic’ interpretation, which implies that the vicinity of these two clusters may well be a coincidence. Additional data are required to conclude. Interestingly, transitional VGPs are found only in the southern hemisphere, which may suggest a long duration for this phase of the reversal. All VGPs seem effectively to belong to the ‘initial’ phase of the transition, with the exception of the Maui record which would incorporate a normal direction before the intermediate VGPs of the southern hemisphere. It is tempting to link the preponderance of VGPs in the southern hemisphere with records of absolute paleointensity which revealed an asymmetry between a slow pre-transitional decaying phase of the dipole followed by a very fast recovery [3,41]. If the decay of the dipole persisted for a very long time, it can be expected to see more transitional directions with VGPs close to the original pole position than close to the new polarity.
5 Field geometry of the transitional field
As a final step we have plotted the A and B datasets together in Fig. 6a. As expected, three large longitudinal bands remain prominent at 100 °E, 0° and 130 °W (Fig. 6b). Interestingly, these three bands coincide with persistent inclination anomalies in the time-averaged field models [6,22], which could suggest some recurring influence of non-dipole components during reversals. It is certainly too early to go further on this matter and the stability of these bands must be tested with more data and better site distributions. In Fig. 6c we removed the effect of clusters (which were considered as not ‘field’ significant) by averaging directions that differ by less than 10° in angular distance. The distribution (Fig. 6d) does not change strikingly, except for the peak over Australia, which is mainly constrained by the clusters and consequently may not be very significant if one relies on the ‘volcanic’ interpretation. The absence of VGPs within the Atlantic, middle west and central Eurasia sectors remains intriguing. Because these sectors are rather depleted of observation sites, we have examined whether the distribution would not be governed by some dependence between the VGPs and their site longitudes. The histogram of the distance between the VGPs longitudes and their site (or antipodal site) meridian (Fig. 6e) is flat.
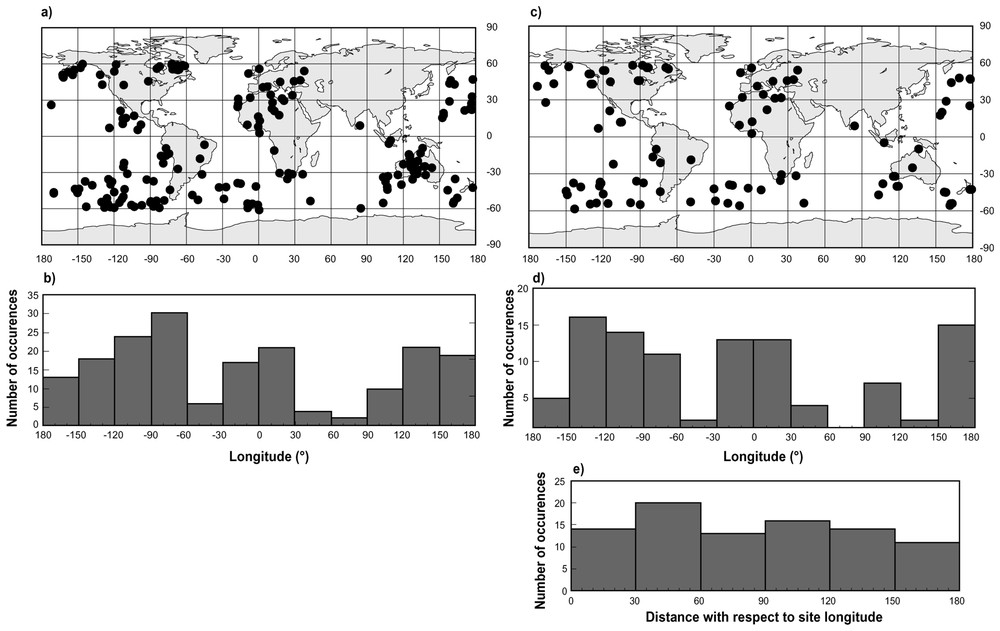
(a) All VGPs from categories A and B. (b) Distribution of the VGP longitudes for all VGPs. (c) All VGPs from categories A and B after averaging VGPs from the same sequence with angular deviations that do not exceed 10°. (d) Same as in (b) after averaging directions that differ by less than 10° in angular distance. (e) Distribution of the distances between the VGPs longitudes and their site meridian.
(a) Tous les PGV des catégories A and B. (b) Histogramme des longitudes des PGV. (c) Représentation des PGV après qu'on ait moyenné ceux des mêmes séquences dont les variations angulaires n'excèdent pas 10°. (d) Histogramme des longitudes après le traitement appliqué en (c). (e) Histogramme des distances entre les longitudes des PGV et le méridien de leur site d'enregistrement.
It is evidently impossible to extract field geometry from the present results. Gubbins and Sarson proposed a model [14] derived from persistent flux patches over long enough a period to produce recurring features during successive field reversals. The transition is controlled by poleward or equatorward migration of patches of reversed flux, similar to those seen in today's field. Because the patches remain concentrated on the same longitudes throughout the transition the VGP paths depend on site position, reversal sense and sense of flux migration. Gubbins and Love [15] found that poleward migration of flux is broadly consistent with sedimentary and volcanic data of the last reversal. We feel that the last reversal is too poorly documented by volcanic records to conclude on this matter.
If we assume that the configuration of the flux patches remained stable over a few million years, we should observe similar transitional characteristics for distinct reversals. In the Gubbins and Sarson model [14], sites at the equator and sites near meridians ±90° (called ‘nodal lines’) will not record persistent VGP paths, but rather random trajectories with rapid changes in direction and lower intensity than elsewhere. It is obvious that the category-A records from Hawaii and Iceland (Fig. 2) are characterized by random VGP paths with apparent rapid changes in direction, but these characteristics are not strikingly different from those of the Steens Mountain, despite the distance of this site from the nodal lines. The record of Lower Mammoth from Hawaii exhibits longitudinally confined VGPs along the Greenwich meridian. However, preference for this meridian is incompatible with the site location close to the 180° nodal lines. Alternatively, it could be explained if the flux was concentrated on the 0° and 180° meridians. Alternations of preferred flux locations along the 0° and 180° and the 90° and 270° longitudes could probably be envisaged, but needs further refinement in theory and additional detailed volcanic records.
6 Conclusion
Despite the limited dataset of reversal records from volcanics, interesting information can be gained by focusing our attention on the most detailed records. The concept of preferred longitudinal bands must be linked to the existence of a third ‘preferred’ longitude over Africa and Europe, which invalidates the existence of two exclusive bands, at least for these records. The rough concordance of these three sectors with long-term inclination anomalies could be appealing, but we cannot exclude that this is a consequence of uneven site distribution that would be distorted by additional detailed records from new locations. A dominant feature is the complex structure of the transitions with large directional swings. A second particularity is the presence of clusters of transitional directions, which are found in all records within various longitudinal bands and thus not only restricted to America and Australia. Since they are not reproduced between multiple records of the same transition, they probably result from intense volcanic activity rather than from steady transitional dipolar states. Alternatively, we cannot prove that such states do not exist. In the present situation, these episodes can simply not be validated as significant features of the reversing field. Complexity appears as a permanent feature of the transitional field and remains consistent with early models of field reversals [9,11] that assume that non-dipolar components similar to the present non-dipole field would dominate the field geometry.
Acknowledgements
This research was supported by the INSU–CNRS program ‘Intérieur de la Terre’. This is IPG contribution No. 1890.