Version française abrégée
1 Introduction
L'analyse de la chaı̂ne varisque de la zone axiale des Pyrénées, est souvent compliquée par la présence d'un plutonisme tardi-varisque (Stéphanien) se surimposant aux anciennes structures et oblitérant au moins partiellement l'histoire tectonométamorphique antérieure [2,4,15]. Le dôme de Bossòst, dans les Pyrénées centrales, correspond à une des zones clé pour la compréhension de l'histoire varisque de la zone axiale des Pyrénées [11,17].
Le dôme de Bossòst est constitué d'un cœur leucogranitique allongé, d'orientation est–ouest, et d'une couverture schisteuse cambro–ordovicienne. La faille de Bossòst, orientée vers l'est, divise le dôme en deux domaines distincts. Dans la moitié nord, le cœur granitique affleure largement, alors que, dans la partie méridionale, seuls de sporadiques filons granitiques apparaissent au sein d'une structure schisteuse doublement antiforme d'axe est–ouest (Fig. 1). La foliation dans les schistes cambro–ordoviciens est orientée ESE et la linéation, marquée par l'allongement minéralogique de la biotite, plonge vers le sud–est [9].
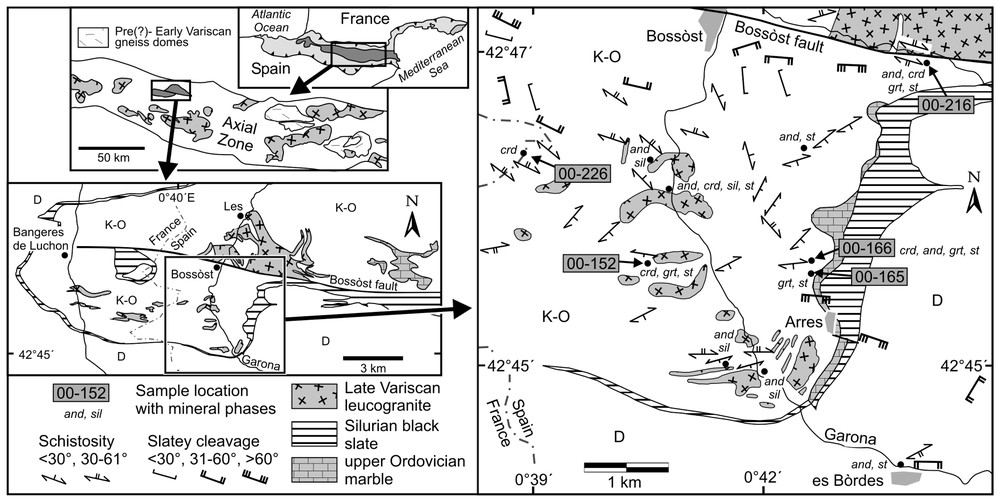
General map of the Bossòst dome and detailed geological map with the location of the microprobe samples and important mineral assemblages. D: Devonian. K–O: Cambro–Ordovician. Geological map is modified after de Sitter and Zwart [3]. Sil: sillimanite.
Carte générale du dôme de Bossòst et carte géologique détaillée, localisant les principales associations minéralogiques rencontrées, ainsi que les échantillons sélectionnés pour les analyses à la microsonde électronique. D : Dévonien. K–O : Cambro–Ordovicien. Carte géologique modifiée après de Sitter et Zwart [3]. Sil : sillimanite.
La présence d'un métamorphisme de contact s'exprime près du cœur granitique par l'occurrence d'andalousite, de sillimanite, et de cordiérite. Nous avons étudié en détail une zone de cisaillement de 1,5 km d'épaisseur, à l'est de la partie méridionale du dôme. Au sein de cette zone de cisaillement, grenat et staurotide montrent des figures de déformation syncinématiques en bon accord avec un cisaillement du toit vers le sud–est. L'andalousite et la cordiérite au contraire, montrent généralement une croissance minéralogique durant une déformation coaxiale postschisteuse [9].
Ce dernier point tend à prouver l'existence d'un métamorphisme polyphasé, ainsi que d'une période de déformation non coaxiale D2a séparant la croissance minéralogique de la staurotide et du grenat (M1) des autres phases (M2). Les analyses par microsonde et par fluorescence X sur roche totale ont été utilisées dans cette étude, afin de distinguer les deux principales phases du métamorphisme. Les pseudosections calculées pour deux échantillons représentatifs montrent que, dans notre cas, la paragenèse staurotide–cordiérite dans le système KFMASH n'est pas stable en présence de muscovite (+ quartz + eau) [5,10]. Une discussion plus détaillée sur les microstructures et la pétrologie du dôme de Bossòst est disponible dans la littérature [9].
2 Compositions minéralogiques et analyses sur roche totale des schistes à staurotide du Cambro–Ordovicien
Cinq échantillons des schistes cambro–ordoviciens ont été sélectionnés pour les analyses à la microsonde (microsonde JEOL-JXA 8900 RL de l'université Johannes Gutenberg, Mayence, Tableau 1). Quatre échantillons sur cinq sont caractérisés par la présence d'assemblages minéralogiques à cordiérite, alors que le dernier contient de façon abondante des porphyroblastes de staurotide et de grenat, mais pas de cordiérite (00-165). La zonation des grenats dans les échantillons à cordiérite (00-152, 00-166, 00-216) montre un enrichissement en almandin (Fe) et un appauvrissement en spessartine (Mn), du cœur à la périphérie (Fig. 2).
Representative electron microprobe data of Cambro–Ordovician schist of the Bossòst dome
Données représentatives de la microsonde des schistes cambro–ordoviciens du dôme de Bossòst
Sample | 00-152 | 00-165 | 00-166 | 00-216 | 00-226 | ||||||
Location | 0°41′00″ E, | 0°42′24″ E, | 0°42′26″ E, | 0°43′18″ E, | 0°39′49″ E, | ||||||
42°45′38″ N | 42°46′15″ N | 42°45′40″ N | 42°47′00″ N | 42°46′15″ N | |||||||
Additional phases | ilm, tur | ilm, pl, gr | and, chl∗, ilm | and, chl∗, ilm, rt | ilm | ||||||
Garnet normalized to 12 O atoms | |||||||||||
Core 1 | Core 2 | Rim | Core | Rim | Core | Rim | Core | Rim 1 | Rim 2 | ||
n | 1 | 3 | 14 | 5 | 9 | 4 | 9 | 2 | 3 | 3 | |
Si | 2.979 | 2.970 | 2.978 | 2.982 | 2.983 | 2.963 | 2.960 | 2.971 | 2.976 | 2.968 | |
Al | 2.039 | 2.036 | 2.035 | 2.046 | 2.043 | 2.056 | 2.054 | 2.050 | 2.040 | 2.060 | |
Fe2+ | 1.551 | 1.753 | 1.918 | 2.518 | 2.621 | 1.891 | 2.029 | 1.828 | 1.981 | 1.865 | |
Mn | 1.010 | 0.772 | 0.595 | 0.190 | 0.134 | 0.689 | 0.575 | 0.783 | 0.650 | 0.731 | |
Mg | 0.203 | 0.279 | 0.312 | 0.117 | 0.128 | 0.287 | 0.289 | 0.267 | 0.271 | 0.279 | |
Ca | 0.202 | 0.189 | 0.158 | 0.139 | 0.085 | 0.114 | 0.103 | 0.097 | 0.081 | 0.094 | |
ΣOxide | 100.49 | 100.79 | 100.67 | 100.19 | 100.37 | 100.12 | 100.59 | 100.88 | 100.94 | 100.80 | |
Xgrs | 0.068 | 0.063 | 0.053 | 0.047 | 0.029 | 0.039 | 0.034 | 0.032 | 0.027 | 0.032 | |
Xprp | 0.069 | 0.093 | 0.105 | 0.040 | 0.043 | 0.096 | 0.097 | 0.090 | 0.091 | 0.094 | |
Xalm | 0.523 | 0.586 | 0.643 | 0.850 | 0.883 | 0.634 | 0.677 | 0.615 | 0.664 | 0.628 | |
Xsps | 0.340 | 0.258 | 0.199 | 0.064 | 0.045 | 0.231 | 0.192 | 0.263 | 0.218 | 0.246 | |
Fe/(Fe+Mg) | 0.884 | 0.863 | 0.860 | 0.955 | 0.953 | 0.868 | 0.875 | 0.873 | 0.879 | 0.870 | |
Staurolite normalized to 48 O atoms | |||||||||||
n | 5 | 37 | 29 | 17 | |||||||
Si | 7.750 | 7.854 | 7.749 | 7.766 | |||||||
Ti | 0.124 | 0.096 | 0.119 | 0.115 | |||||||
Al | 19.004 | 19.032 | 18.913 | 19.016 | |||||||
Fe | 2.722 | 3.222 | 2.998 | 2.718 | |||||||
Mn | 0.145 | 0.023 | 0.116 | 0.124 | |||||||
Mg | 0.497 | 0.254 | 0.692 | 0.605 | |||||||
Zn | 0.360 | 0.036 | 0.070 | 0.247 | |||||||
ΣOxide | 97.10 | 96.53 | 96.96 | 97.04 | |||||||
Mg/(Fe+Mg) | 0.142 | 0.073 | 0.187 | 0.181 | |||||||
Mn/(Fe+Mg+Mn+Zn) | 0.042 | 0.007 | 0.030 | 0.034 | |||||||
Zn/(Fe+Mg+Mn+Zn) | 0.096 | 0.010 | 0.018 | 0.066 | |||||||
Cordierite normalized to 18 O atoms | |||||||||||
n | 11 | 5 | altered to Ms | 16 | |||||||
Si | 5.014 | 5.040 | (see below) | 5.031 | |||||||
Al | 3.969 | 3.904 | 3.992 | ||||||||
Fe2+ | 0.725 | 0.756 | 0.680 | ||||||||
Mn | 0.059 | 0.044 | 0.011 | ||||||||
Mg | 1.177 | 1.181 | 1.158 | ||||||||
Na | 0.105 | 0.151 | 0.193 | ||||||||
ΣOxide | 97.95 | 97.45 | 97.17 | ||||||||
Mg/(Fe+Mg) | 0.619 | 0.610 | 0.630 | ||||||||
Mn/(Fe+Mn+Mg) | 0.022 | 0.030 | 0.006 | ||||||||
Biotite normalized to 22 O atoms | |||||||||||
contact | blast | altered | |||||||||
n | 9 | 11 | 1 | 11 | 12 | 12 | |||||
Si | 5.312 | 5.368 | 5.297 | 5.369 | 4.690 | 5.361 | |||||
Ti | 0.236 | 0.256 | 0.196 | 0.202 | 0.124 | 0.278 | |||||
Al | 3.501 | 3.462 | 3.757 | 3.489 | 3.926 | 3.480 | |||||
Fe | 2.444 | 2.329 | 3.518 | 2.404 | 3.105 | 2.360 | |||||
Mn | 0.043 | 0.033 | 0.006 | 0.025 | 0.055 | 0.008 | |||||
Mg | 2.240 | 2.223 | 0.893 | 2.246 | 2.933 | 2.173 | |||||
Ca | 0.016 | 0.003 | 0.000 | 0.013 | 0.007 | 0.002 | |||||
Na | 0.043 | 0.058 | 0.019 | 0.072 | 0.009 | 0.060 | |||||
K | 1.602 | 1.678 | 1.731 | 1.586 | 0.571 | 1.637 | |||||
ΣOxide | 94.43 | 94.70 | 94.28 | 95.00 | 90.03 | 95.14 | |||||
Fe/(Fe+Mg) | 0.522 | 0.512 | 0.798 | 0.517 | 0.514 | 0.521 | |||||
Muscovite normalized to 22 O atoms | |||||||||||
matrix | contact | matrix | altered Crd | ||||||||
n | 3 | 5 | 9 | 6 | 3 | 3 | 4 | ||||
Si | 6.164 | 6.069 | 6.084 | 6.059 | 6.018 | 6.084 | 6.056 | ||||
Ti | 0.053 | 0.041 | 0.024 | 0.039 | 0.033 | 0.003 | 0.062 | ||||
Al | 5.535 | 5.752 | 5.753 | 5.795 | 5.846 | 4.970 | 5.729 | ||||
Fe2+ | 0.129 | 0.082 | 0.124 | 0.097 | 0.094 | 0.515 | 0.086 | ||||
Mg | 0.176 | 0.090 | 0.046 | 0.085 | 0.082 | 0.878 | 0.109 | ||||
nA | 0.190 | 0.226 | 0.328 | 0.331 | 0.349 | 0.043 | 0.267 | ||||
K | 1.640 | 1.660 | 1.564 | 1.472 | 1.431 | 1.658 | 1.613 | ||||
ΣOxide | 95.45 | 95.40 | 94.27 | 95.63 | 95.48 | 95.76 | 94.74 | ||||
Fe/(Fe+Mg) | 0.423 | 0.477 | 0.729 | 0.533 | 0.534 | 0.370 | 0.489 |
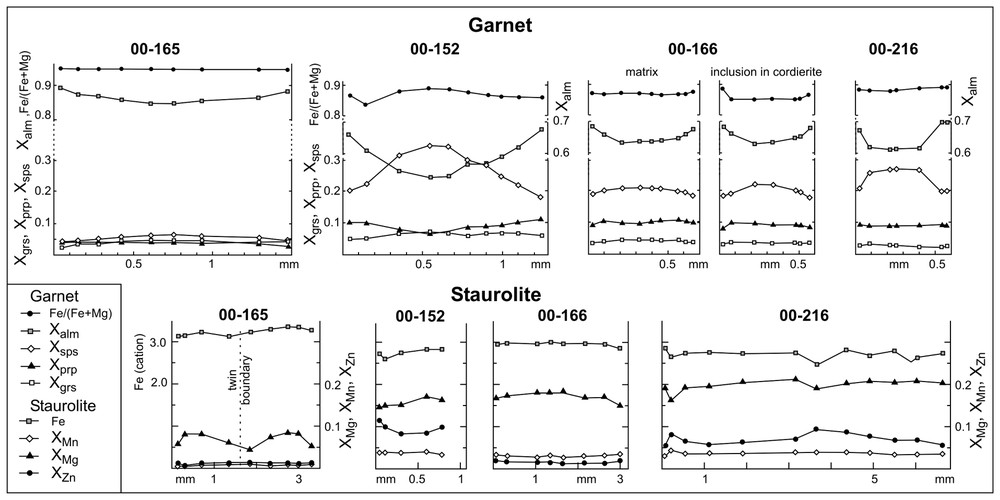
Representative compositional rim-to-rim profiles of garnet, staurolite and cordierite. Xalm(Xgrs,Xprp,Xsps)=Fe(Ca,Mg,Mn)/(Fe+Mn+Mg+Ca); XMg=Mg/(Fe+Mg); XMn(XZn)=Mn(Zn)/(Fe+Mn+Mg+Zn).
Coupes représentatives des compositions du grenat, de la staurotide et de la cordiérite. Xalm(Xgrs,Xprp,Xsps)=Fe(Ca,Mg,Mn)/(Fe+Mn+Mg+Ca). XMg=Mg/(Fe+Mg) ; XMn(XZn)=Mn(Zn)/(Fe+Mn+Mg+Zn).
Les grenats des échantillons sans cordiérite sont riches en almandin (Xalm>85) par rapport aux autres échantillons (Xalm 52–68) et ne présentent pas de zonation significative. Dans tous les échantillons, la constance du rapport Fe/(Fe+Mg) suggèrent une température également constante pendant la nucléation. Les staurotides de l'échantillon 00-165 sont relativement riches en Mg et pauvres en Zn par rapport à ceux coexistant avec la cordiérite (Tableau 1, Fig. 2).
La cordiérite dans les échantillons 00-152 et 00-226 a un rapport Mg/(Mg+Fe) de l'ordre de 0,61–0,63, alors que, pour les autres échantillons, pinnite et chlorite cristallisent durant la kélyphitisation presque totale de la cordiérite. Les différentes générations de biotite ou de muscovite ne sont pas distinguables d'un point de vue chimique. Une possible interprétation est le rééquilibrage compositionnel durant le refroidissement. L'ilménite est abondamment présente en inclusion dans la cordiérite, et représente probablement le résidu de la transformation de la biotite en cordiérite, cette dernière ne pouvant pas incorporer le TiO2 (Tableau 1).
L'analyse des éléments majeurs sur deux échantillons représentatifs montre que les échantillons sans cordiérite (00-165) sont appauvris en Mn, Ca et Mg par rapport à ceux contenant de la cordiérite (00-166). Ce qui explique la nucléation du grenat riche en almandin et l'absence de cordiérite (Tableau 2).
Whole rock XRF analyses of Cambro–Ordovician schist from the Bossòst dome
Analyses par fluorescence X sur roche totale des schistes cambro–ordoviciens du dôme de Bossòst
Oxides (wt.%) | 00-165 | 00-166 |
SiO2 | 59.01 | 58.59 |
Al2O3 | 22.86 | 19.43 |
TiO2 | 1.08 | 0.61 |
Fe2O3 | 5.98 | 6.76 |
MnO | 0.04 | 0.12 |
CaO | 0.12 | 0.78 |
MgO | 0.56 | 2.77 |
K2O | 5.25 | 3.26 |
Na2O | 0.52 | 1.66 |
P2O5 | 0.09 | 0.11 |
Cr2O3 | 0.016 | 0.013 |
LOI | 3.46 | 2.75 |
Total | 98.56 | 97.27 |
3 Estimations des conditions P–T du métamorphisme polyphasé
Pressions et températures ont été estimées via le calcul de deux pseudosections en utilisant le logiciel THERMOCALC 3.2.1 [12]. Les conditions pour la phase M1 du métamorphisme (échantillon 00-165), peuvent être estimées de l'ordre de 5,5 kbar et 580 °C (champ divariant à grenat–staurotide–biotite, Fig. 3(a), (c)). La pseudosection pour l'échantillon 00-166 montre que, d'une façon théorique, la staurotide n'est pas stable en présence de cordiérite (Fig. 3(b), (d)). Ceci implique que la staurotide a probablement cristallisé avant l'apparition de la cordiérite, en bon accord avec les microstructures associées. En d'autres termes, la composition sur roche totale n'est probablement pas exactement la composition effective de la roche à Tmax pendant la phase M2 du métamorphisme (métamorphisme de contact pendant l'exhumation). Le fractionnement compositionnel de cette roche après la première phase M1 du métamorphisme a donc probablement favorisé la cristallisation de la cordiérite durant M2 vers 525 °C et 2 kbar ; ceci, par exemple, au sein des biotites de première génération (M1) plus riches en Mg. En résumé, les pseudosections calculées pour ces deux échantillons représentatifs montrent que, dans notre cas, la paragenèse staurotide–cordiérite dans le système KFMASH n'est pas stable en présence de muscovite (+ quartz + eau) [5,10]. Le chemin P–T résultant est donc un chemin horaire en décompression (Fig. 3). Il est important de noter ici que le caractère métastable de l'association staurotide–cordiérite–biotite–muscovite ne peut être généralisé pour décrire un chemin en décompression. En effet, pour des roches pauvre en potassium – ce qui n'est pas notre cas ici – la projection par la muscovite n'est plus possible dans le système KFMASH. Dans ce cas, staurotide–cordiérite–andalousite–biotite, par exemple, est un champ divariant stable mais sans muscovite. D'autre part, ce dernier champ peut être très proche d'un champ à staurotide–biotite–andalousite–muscovite et la muscovite théoriquement métastable peut très bien être encore présente. Un des exemples possibles pour ce type d'association faiblement métastable peut être trouvé au sein des « schistes X » de la Montagne Noire [8] et a été décrit comme une des paragenèses critiques du métamorphisme de contact dans cette zone [13]. Une analyse fine des microstructures et le calcul de pseudosections est donc nécessaire avant toute conclusion [9, cette étude].
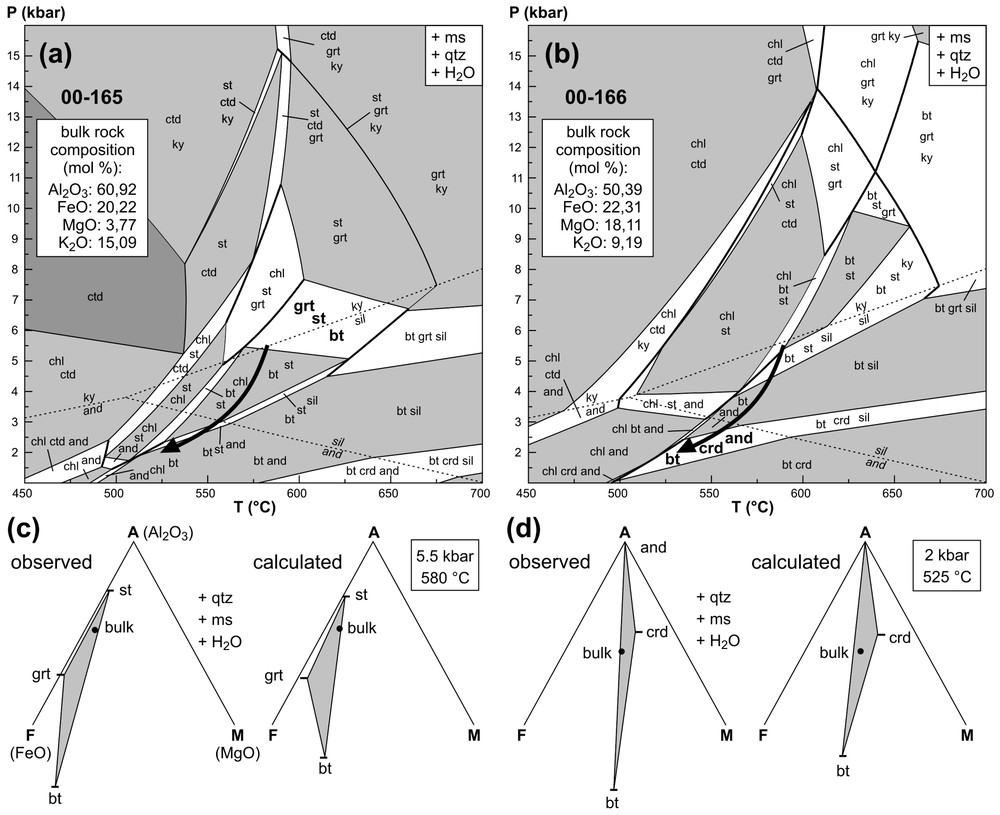
P–T pseudosections and AFM diagrams for samples 00-165 ((a), (c)) and 00-166 ((b), (d)) in the KFMASH system projected from muscovite, quartz and water. Pseudosections and ‘calculated’ AFM diagrams are computed with THERMOCALC (v. 3.2.1) [12] using normalized mol.% of the bulk rock composition (Table 2) with Fe2O3 recalculated to FeO. The pressures and temperatures were chosen to fit best the bulk rock compositions. ‘Observed’ AFM-diagrams use microprobe analyses of Table 1. The slight discrepancy in the location of biotite in the AFM diagrams is due to the fact that pure muscovite is used to obtain the observed AFM diagrams, while the Tschermak's substitution is considered in the calculated diagrams. Large bold letters present the stable assemblages in the samples. The bold arrow indicates the P–T path. ctd: chloritoid; ky: kyanite.
Pseudosections P–T et diagrammes AFM associés pour les échantillons 00-165 ((a), (c)) et 00-166 ((b), (d)), système KFMASH (+ muscovite + quartz + eau en excès). Les pseudosections et les diagrammes AFM ont été calculés en utilisant le logiciel THERMOCALC (v. 3.2.1) [12]. Afin de préciser les conditions P–T, nous avons comparé, pour les deux échantillons, les diagrammes AFM obtenus avec THERMOCALC, avec ceux obtenus par projection des phases minéralogiques via les analyses microsondes (Table 2). Noter que la projection via les analyses à la microsonde utilise la muscovite au sens strict du terme (membre pur) d'où la légère différence entre les biotites projetées (calculées, observées). Le chemin P–T en décompression est aussi figuré. ctd : chloritoı̈de ; ky : kyanite.
4 Conclusions
Les pseudosections calculées, ainsi que les relations de phases et les microstructures associées [9], permettent de proposer un chemin horaire en décompression au sein des schistes cambro–ordoviciens du dôme de Bossòst. La phase M1 du métamorphisme s'effectuerait à moyenne pression et haute température, alors que la phase M2 prendrait place à basse pression et moyenne température. Cette décompression est à rattacher à la présence d'une zone de cisaillement majeure en extension vers l'est, au sud–est du dôme de Bossòst. Les contraintes temporelles pour ce détachement majeur sont, d'une part, la formation de la principale foliation durant le Westphalien moyen [7] et, d'autre part, l'emplacement du pluton granitique durant le Stéphanien [4]. Ce qui correspondrait à une courte période de temps pour l'extension vers l'est, dans un contexte régional NNE–SSW en compression [8]. Le même type d'extension tardi-varisque décrite dans le massif de l'Hospitalêt (zone axiale est) [15], ainsi que les conditions du métamorphisme dans le Massif de Lys-Caillaouas au sud [6] et dans le dôme de Soulcem à l'ouest du massif d'Aston [16], indiquent que l'extension tardi-varisque n'est pas un phénomène rare dans la zone axiale des Pyrénées.
1 Introduction
The importance of the relation between deformation and porphyroblast growth for tectonic interpretation of metamorphic terranes was first recognized by Zwart [17] in a landmark study of the Bossòst dome, also known as the dôme de Garonne, in the Variscan Axial Zone of the Central Pyrenees (Fig. 1). Key areas to study the polyphase Variscan deformation history of the Axial Zone are the structural and metamorphic domes, orthogneiss or migmatite cores mantled by Cambro–Ordovician metasedimentary rocks [2]. Tectonic interpretations are complicated by widespread Late Variscan (Stephanian) plutonism which obliterated older structures and metamorphic events in many areas [4,15]. A later study of the Bossòst dome [11] proposed a polymetamorphic origin of staurolite–bearing assemblages related to diapiric emplacement of the granitic core. Recent petrological studies [5,10] suggest that staurolite–cordierite–muscovite assemblages are the result of decompression along a clockwise P–T path. We present electron microprobe and whole rock XRF data from staurolite–bearing assemblages of different bulk rock compositions that preserve earlier regional M1 and later contact M2 metamorphism. P–T pseudosections constrain the metamorphic conditions during M1 and M2. A detailed discussion of the microstructure and petrology of the Bossòst dome is presented elsewhere [9].
2 Structural and metamorphic character of the Bossòst dome
The Bossòst dome is an elongated, easterly trending structure consisting of a core of leucogranitic intrusions concentrically mantled by Cambro–Ordovician metapelites and micaceous quartzites (Fig. 1). The main fabrics are an ESE-striking schistosity (S1/2), gradually steepening to subvertical at the dome margins, and a strong south–east–trending biotite mineral lineation [9]. The east–trending Bossòst fault divides the Bossòst dome into two distinct structural domains, a northern half-dome where deeper parts of the granitic core are exposed, and a southern east–west–trending, doubly plunging antiform, cored by granitic sills. Contact metamorphism is indicated by andalusite, sillimanite and cordierite present near the granitic core and decreasing metamorphic grade from the core outwards. In the northern section, garnet and staurolite are rare, and andalusite and cordierite porphyroblasts record strong coaxial deformation. The eastern part of the southern antiform is characterized by a 1.5 km thick shear zone with rotated garnet and staurolite porphyroblasts, indicating top–to-the-SE shear that were overprinted by poikiloblastic andalusite and cordierite, showing late synkinematic growth [9]. Structurally below the shear zone, garnet and staurolite occur as relics. Lenses of fibrolitic sillimanite and cordierite porphyroblasts indicate generally coaxial deformation. Hence, metamorphism appears to be polyphase, and a period of non-coaxial deformation D2a separates the growth of staurolite and garnet (M1) from andalusite, sillimanite and cordierite (M2).
3 Staurolite–bearing Cambro–Ordovician schist
3.1 Sample description and analytical procedures
The five samples of Cambro–Ordovician schist selected for mineral analyses (garnet, biotite, staurolite, muscovite and cordierite) are from the eastern part of the southern antiform (Fig. 1, Table 1). Two samples (00-166, 00-216) record the highest number of mineral phases. These are, in order of growth sequence, muscovite, biotite, garnet, staurolite, andalusite+second biotite, and cordierite. Centimetre-sized cordierite porphyroblasts are generally altered and contain inclusions of earlier phases and ilmenite. Samples 00-152 and 00-226 contain no andalusite, and cordierite porphyroblasts are less altered. Different from the other samples is 00-165, structurally overlying nearby 00-166, because it contains abundant large (∼5 mm) euhedral porphyroblasts of garnet and twinned staurolite. Cordierite is conspicuously absent.
Mineral analyses were carried out at the Johannes Gutenberg-University Mainz using a JEOL-JXA 8900 RL electron microprobe. The instrument is operated at an acceleration voltage of 20 kV and a beam current of 20 nA for garnet and staurolite measurements, and at 15 kV and 12 nA for biotite, cordierite and muscovite. Peak counts were measured for 15–30 s. The analytical precision of the oxides at the level of several weight percent is approximately 1–2% relative and, for oxides in lower abundance (<1 wt.%), the precision is 10–20% relative. Microprobe data were reduced using CIT–ZAF reduction procedures.
3.2 Mineral composition
Garnet compositions differ significantly between cordierite–bearing and cordierite–absent assemblages (Table 1). Distinct garnet zoning patterns are observed in cordierite–bearing samples 00-152, 00-166 and 00-216. The almandine (Fe) component increases significantly from core to rim, while the spessartine (Mn) component decreases (Fig. 2). Low abundances and weak zoning characterize the pyrope (Mg) and grossular (Ca) components. Garnet inclusions in cordierite have similar rim compositions as matrix garnets and show no textural evidence of rim corrosion, suggesting that cordierite formation did not consume significant amounts of garnet. In contrast to cordierite–bearing assemblages, garnets of the cordierite–absent sample 00-165 are enriched in the almandine component (Xalm>85 versus Xalm 52–68). Zoning patterns are similar, but less pronounced (Fig. 2). All samples display constant Fe/(Fe+Mg) ratios along compositional profiles, suggesting constant temperatures during growth. Minor increases near the rim are attributed to re-equilibration during cooling [14].
Staurolite compositions differ between cordierite–bearing and –absent assemblages. Staurolite porphyroblasts of 00-165 are relatively enriched in Fe and depleted in Mg and Zn with respect to staurolites with co-existing cordierite. High Zn content (1.7 vol.% ZnO) of relict staurolite of 00-152 rimmed by muscovite pseudomorphs is attributed to back partitioning of Zn during temperature increase. All samples display moderate core–rim decreases in the Mg-content of staurolite. Sector zoning with low Mg-contents can be observed at boundaries of adjacent staurolite twins (Fig. 2).
Mg/(Mg+Fe) values of unaltered cordierite (00-152, 00-226) are in the order of 0.61–0.63. Significantly altered cordierite (00-166, 00-216) consists of fine–grained intergrowth of muscovite and chlorite, known as pinitization. Pinitic muscovite is relatively enriched in Mg and Fe, and depleted in Na, compared to matrix muscovite, preserving the chemical signature of the replaced cordierite. The composition of fine grained matrix muscovite is similar to that of younger coarse muscovite rimming staurolite or forming pseudomorphs after staurolite. Likewise, first generation biotite porphyroblasts do not differ in composition from second generation foliation-parallel biotite. We consider this as a result of chemical re-equilibration during cooling rather than an original feature. Biotite of one sample (00-216) has been chloritized, although it still can be distinguished optically, providing K for the pinitization process.
Ilmenite is abundant as inclusions in cordierite where it is thought to be a product of the breakdown of biotite to form cordierite, incorporating TiO2 from biotite which is not accommodated by cordierite (Table 1). This concurs with a lower abundance of biotite inclusions in cordierite compared to biotite in the matrix.
4 Bulk rock analyses and P–T constraints of regional and contact metamorphism
Whole rock major element analyses of two representative samples show a significant depletion in Mg, Mn and Ca for the sample lacking cordierite (00-165) compared to the cordierite–bearing sample 00-166 (Table 2). In 00-165, cordierite growth is suppressed by insufficient Mg. The relative abundance of Mn in 00-166 favoured nucleation of spessartine-rich garnets prior to staurolite growth, which is supported by the presence of garnet inclusions in staurolite [14]. On the other hand, almandine garnets of 00-165 probably post-dated staurolite growth, since they are not observed as inclusions. This suggests that the bulk rock composition controls the sequence of mineral nucleation during the same thermal event [9].
Pressure and temperature estimates were derived from pseudosections corresponding to the analyzed rock compositions, as well as AFM diagrams computed with THERMOCALC 3.2.1 [12]. For the garnet–staurolite schist 00-165 values of 5.5 kbar and 580 °C were calculated, which lie at the lower pressure and temperature range of the garnet–staurolite–biotite stability field, and are thought to reflect conditions during regional metamorphism M1 (Fig. 3(a), (c)). The calculated AFM diagrams are in good agreement with AFM diagrams obtained from individual phase analyses (Fig. 3(c), (d)). The pseudosection for sample 00-166 shows that staurolite is not stable within the cordierite–andalusite–biotite stability field (Fig. 3(b)). This implies that staurolite grew before cordierite, which is in agreement with observed microstructures [9]. Thus, compositional fractionation occurred after M1, which means that the rock chemistry which favoured crystallization of cordierite at 525 °C and 2 kbar during M2 contact metamorphism is not identical with the analyzed bulk rock composition. The availability of Mg from the first generation biotite facilitated cordierite growth. The calculated pseudosections show that in our case the staurolite–cordierite association is not stable with muscovite in the KFMASH system [5,10]. Therefore, we can assume a clockwise decompression P–T path (Fig. 3). It should be noted that the metastable character of the staurolite-cordierite association does not automatically imply a decompressional P–T path. Staurolite and cordierite can be stable in the KFMASH system in potassium-poor rocks lacking muscovite. Nevertheless, the proximity of the staurolite–muscovite stability field can result in the presence of metastable muscovite in these rocks, as seen in the ‘schistes X’ of the Montagne Noire [8], where the critical paragenesis shows staurolite, cordierite, biotite and muscovite in textural equilibrium during contact metamorphism [13]. This exemplifies that it is crucial for the understanding of P–T paths to combine microstructural analyses with calculation of pseudosections.
5 Conclusions
Rocks of different bulk rock composition record various phases of the metamorphic history of the Bossòst dome, which describes a clockwise P–T path [9]. P–T pseudosections and AFM diagrams show that early, higher pressure regional metamorphism (M1), preserved in Mg-poor staurolite–garnet schist, is followed by low pressure contact metamorphism (M2).
The metastable nature of staurolite in cordierite–andalusite M2 assemblages provides additional evidence for Late Variscan decompression path inferred from the presence of an extensional shear zone located in the southeastern Bossòst dome [9]. Timing of orogen-parallel extension is constrained by formation of the main schistosity during the Mid-Westphalian [7] and the Stephanian emplacement of the granitic plutons [4], and may reflect a short period of extensional in an overall NNE–SSW compressional setting [9]. Similar Latest Variscan extensional structures, a crustal-scale shear zone overlying orthogneisses of the Hospitalêt massif in the eastern Axial Zone [15], and metamorphic conditions in the Lys-Caillaouas massif to the south [6] and the Soulcem metamorphic dome west of the Aston massif [16], indicate that Late Variscan extension was not uncommon throughout the Axial Zone of the Pyrenees. In the easternmost Axial Zone different metamorphic conditions may have prevailed, as reported in a recent study on the Canigou massif, where kyanite–staurolite assemblages represent a late medium pressure metamorphism [1].
Acknowledgements
This research was supported by the Deutsche Forschungsgemeinschaft (PA 578/5-1). Birte Anders helped with the microprobe analyses. Comments by Philippe Matte and an anonymous reviewer are appreciated.