1 Introduction
All along the Mediterranean coast, North-Africa is fringed by a young, Alpine orogenic system, i.e. the Rif–Tell belts (Fig. 1), also referred to as the Maghrebide belt [43,63,148]. This belt connects eastward with the Apennines through the Sardinia Channel [105], northern Sicily (Peloritan mountains) and the Calabrian Arc [22,43], whereas it connects to the west with the Betic Cordilleras through the Gibraltar Arc and Alboran Sea basement [35,43,64]. The Maghrebide External zones essentially consist of sedimentary material of the Jurassic–Cretaceous African margin, and escaped Alpine metamorphism, except some of their most internal units, affected by very low-grade to low-grade greenschist-facies metamorphism [56,60,89]. Likewise, the overlying Maghrebian Flyschs nappes, which are the remnants of a Neotethyan oceanic basin [47], are virtually devoid of metamorphism, except some internal ophiolite-bearing slivers [19]. By contrast, parts of the allochthonous, Maghrebide Internal zones or Kabylides of Algeria (Kabylias and equivalents) and Morocco (Northern Rif) display varied metamorphic complexes.

Sketch map of the Rif belt in the frame of the West Mediterranean Alpine belts, after [43]. Empty arrows: Africa–Eurasia active convergence, NUVEL 1 model, in [109]. Framed: location of Fig. 2A, with trace of cross-section Fig. 2B. BB: Beni Bousera; R: Ronda; SN: Sierra Nevada.
Localisation des Maghrébides dans le cadre des chaînes alpines de Méditerranée occidentale, d'après [43]. Flèches vides : convergence actuelle Afrique–Eurasie, modèle NUVEL 1, in [109]. BB : Beni Bousera ; R : Ronda ; SN : Sierra Nevada.
Blueschist- and eclogite-facies rocks were observed two centuries ago in the Sierra Nevada range of Central Betics [11,15], and ascribed since years to a typical Alpine, high-pressure, low-temperature (HP–LT) metamorphism [124]. By contrast, the Maghrebide metamorphic massifs and their Betic equivalents above the Sierra Nevada and Sierra de los Filabres deep complexes (Nevado-Filabrides) have been regarded for long as fragments of an ancient continental block passively included in the Alpine belt [21,43,86]. During the late 1970s–early 1980s, a wealth of Alpine ages was measured in the higher grade units of the Betic and Maghrebide belts [97,110,117,128,139]. Structural and petrologic studies also demonstrated the occurrence of post-Triassic, HP–HT metamorphism in some of these internal massifs [3,4,110,165], and more recently, post-Triassic, HP–LT metamorphism was unravelled in large areas of the Betic and Rif belts [23,67,114,168]. However, other studies emphasized in the meantime the occurrence of Variscan metamorphism in parts of the Internide massifs [17,29], thus leading to a great complexity in the metamorphic pattern of the belt. Controversies also arose concerning the age and mode of emplacement of the large, subcontinental peridotite massifs of the Betic Cordilleras (Ronda) and Rif (Beni Bousera). They were regarded either as metamorphic slivers of lithospheric mantle [86,111,147,172,176,179], or as Miocene asthenospheric diapir(s) [97,121,131,167], or else as the result of Miocene diapirism into older lithospheric mantle [135,170].
Improving our knowledge of the metamorphic evolution (i.e. the peak conditions of metamorphism, and associated time dates) of the Maghrebide Internal zones can bring valuable enlightenments to the geodynamic interpretation of the belt. The Betic–Rifian Internides, referred to as the Alboran Domain [9,64], the Kabylias, Peloritan mountains, and Calabrian nappes are currently regarded as the disrupted remnants of a single orogenic complex stuck to the Iberian–Sardinian southeast margin by the end of the Eocene. However, the formation of the latter orogenic prism prior to the Late Eocene is controversial, and regarded, either as involving two successive, and opposite subduction zones [36,41,42,62,115,144], i.e. an early, southeast-dipping Alpine–Betic subduction followed by a northwest-dipping Apenninic–Maghrebide subduction, or else as related to a single, protracted, northwest-dipping Apenninic–Maghrebide–Betic subduction zone [55,84,91,150]. This also means that the pre-Eocene, palaeogeographic origin of the allochthonous continental terranes included in the Maghrebides and associated arcs is controversial, either from an isolated block within the Alpine ocean (‘two-subduction’ models), referred to as ‘Alkapeca’ [22] or ‘Mesomediterranean’ block [73,74], or from the rim of the Iberia–Sardinia margin itself (‘one subduction hypothesis’).
The present paper firstly aims at summarizing the recent advances in calibration and dating of the Alpine events in the Rifian transect of Maghrebides, partly based on data from unpublished thesis or most recent publications of some of the authors [23,83,123,153], and implemented by the Betic data. Second, we compare the Rifian data with those from the Kabylian transects, taken from the literature. We eventually discuss the implications of the reported constraints on the Maghrebide belt mountain building in the highly complex geodynamic framework of western Mediterranean.
2 Geological setting of the Rif Internides
The Rif External domains, i.e. from south to north, the Prerif, Mesorif, and Intrarif zones (Fig. 2A) mostly consist of Mesozoic–Eocene, pre-orogenic sediments from the rifted continental margin of Africa [56], detached along Upper Triassic evaporitic redbeds and thrust towards the Atlasian–Mesetan foreland (Fig. 2B). Syntectonic turbiditic sediments accumulate from the Late Eocene onward within structural, synfolding depocentres [33,178]. The Jurassic–Lower Cretaceous low-grade metasediments of the most internal, Intrarif zone are mostly unrooted. However, in the Beni Malek massif, they stratigraphically overlie serpentinites and metabasites that likely originate from a sub-oceanic serpentinite ridge at the very tip of the continental margin [50,112].

Structural map (A) and sketch crustal cross-section (B) of the Rif belt, after [33,115,162]. See Fig. 1 for location. Offshore structures after [31,49]. Geological and crustal structure onshore after [56], deep structure of the Alboran basin after [164].
Carte structurale (A) et coupe à l'échelle crustale (B) de la chaîne rifaine, d'après [33,115,162]. Localisation : Fig. 1. Structure offshore d'après [31,49], onshore d'après [56], structure profonde du bassin d'Alboran d'après [164].
Two sets of allochthonous, unrooted units widely overthrust the External, North-African material, (i) the Maghrebian Flyschs, involving several diverticulated sequences, some of them beginning with pillow basalts and Jurassic radiolarites, and the uppermost nappe mainly made up by Late Oligocene–Aquitanian coarse siliciclastic turbidites (Numidian flysch); these nappes extend from southern Apennines to Gibraltar, and record the former occurrence of the Maghrebian-Ligurian ocean north of Africa [43,81]; (ii) restricted klippes of weakly metamorphic material, i.e. the Ras Afraou, Khebaba and Melilla-Tres Forcas units of controversial origin in eastern Rif.
The Internide units (Alboran Domain, or Terrane [115]) are juxtaposed to the Flyschs nappes and Intrarif units through a thrust contact or, locally, through a wrench fault (Jebha). The Internide tectonic pile includes, from top to bottom structurally (Figs. 2A–B, 6): (i) the Dorsale Calcaire, a stack of unrooted carbonate slabs made up of Upper Triassic–Liassic platform to margin deposits, Jurassic–Cretaceous pelagic sediments, Palaeocene–Eocene variegated clays and calcarenites, and Late Eocene–Aquitanian clastics and olistostromes [45,51,52], equivalent to the Betic ‘Rondaides’; the Predorsalian sequences [45,125] and the “Tariquide” carbonate units [48], i.e. the Gibraltar Rock and its Moroccan counterpart, are transitional between the Dorsale and Flysch domains; (ii) the Ghomaride nappes, equivalent to the Malaguide ones, involving Ordovician to Lower Carboniferous metasediments followed upward by unconformable Triassic redbeds, and more locally by Liassic limestones and Palaeocene–Eocene calcarenites [29,98]; (iii) the Sebtides units, equivalent to the Alpujarrides, and involving a sequence of Permian–Triassic metasediments (Upper Sebtides) on top of continental crust material associated with ultramafics (Lower Sebtides of Beni Bousera) [23,25,86,110,153,154]. Clastic Oligo-Miocene ‘post-nappe’ deposits unconformably overlie the Ghomaride pile close to the Alboran seashore [33,57,103]. Flysch inliers (e.g., Jbel Zemzem) locally emplaced on top of the latter deposits through extensional sliding.
The Alboran basin is characterized by a thick (up-to-8-km) Neogene turbiditic infilling, overlying a thinned continental crust (15-km-thick), where Alpujarride-type rocks have been cored on structural highs [31,35,133,155]. Late-orogenic, calc-alkaline volcanism is widespread both onshore and offshore (‘Trans-Alboran province’), mostly dated to Middle–Late Miocene [79,107]. Faulting and opening of the Gibraltar strait itself only occurred during the Pliocene [43,54].
3 Internal Rif metamorphic structure and P–T conditions
3.1 General
In the Rif Internides, the Dorsalian and Ghomaride Mesozoic–Cenozoic formations virtually escaped metamorphic recrystallization, and the greenschist-facies metamorphism that affects the Ordovician–Devonian formations of the Ghomaride nappes essentially results from the Variscan orogeny [29]. Only at the very bottom of the lowest Ghomaride (Aakaili) nappe, did occur Alpine chlorite and biotite growth (see Section 4.3). By contrast, the underlying Sebtide units registered strong Alpine metamorphic events. Therefore, they can be regarded as lower plate units of a metamorphic core complex, the upper plate of which consists of the Ghomarides. These lower plate units crop out in northern Rif within four antiformal structures, i.e. the large Beni Mezala and Beni Bousera antiforms, and the smaller Ceuta (Sebta) and Cabo Negro ones. Additionally, in eastern Rif, small allochthonous klippes (Ras Afraou and equivalents) can be hypothetically interpreted as Sebtide outliers.
3.2 Beni Mezala antiform
Four Upper Sebtide units have been recognized in this antiform (Fig. 3A), from top to bottom the Tizgarine, Boquete Anjera, Beni Mezala-2 and Beni Mezala-1 units (Federico units in [86]). They all exhibit the same stratigraphic succession, from bottom to top, Permo-Carboniferous metagreywackes, reddish (Tizgarine) to dark violet (Beni Mezala) phyllites derived from Permian–Triassic redbeds, Lower Triassic quartzites, and Middle–Upper Triassic dolomites. At the very bottom of the Beni Mezala-1 unit, the Benzu schists can be ascribed to the Lower Sebtides by comparison with the Filali schists (Section 3.3).
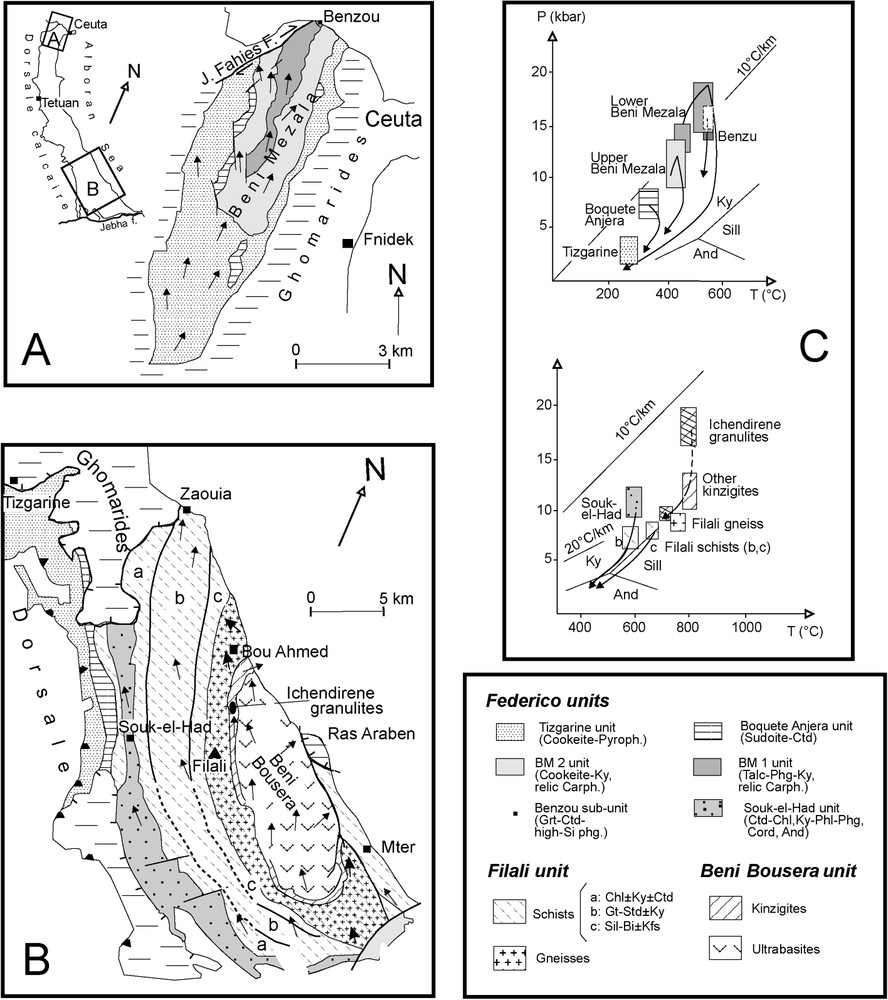
Metamorphic structure and P–T conditions of the main Sebtide units. A: Beni Mezala antiform, after [86]; arrows: late metamorphic shear sense indicators [153,177]. B: Beni Bousera antiform [29,86]; metamorphic zones of the Filali schists after [123]; internal structure of the Beni Bousera ultramafics after [147,154,163]; arrows: late metamorphic shear sense indicators after [147,154]. C: P–T conditions calculated for the northern Federico metapelites and Benzu schists (top), and for the southern Federico (Souk-el-Had) metapelites and Filali schists (bottom), after [23].
Structure métamorphique et conditions P–T des unités sebtides principales. A : Antiforme des Beni Mezala, d'après [86] ; flèches : directions de cisaillement tardi-métamorphiques [153,177]. B : Antiforme des Beni Bousera, d'après [29,86] ; zones métamorphiques des schistes du Filali d'après [123] ; structure interne des ultramafites d'après [147,154,163] ; flèches : cisaillements tardi-métamorphiques d'après [147,154]. C : Conditions P–T calculées pour les métapélites du Federico septentrional et les schistes de Benzu (en haut), et pour les métapélites du Federico méridional (Souk-el-Had) et les schistes du Filali, d'après [23].
Peak conditions (Fig. 3C, top) have been estimated by calculation of phase equilibriums in the Permian–Triassic metapelites [23,24,174]. In the Tizgarine unit, cookeite, pyrophyllite, low-substituted phengite assemblages correspond to LT–LP conditions. In the Boquete Anjera unit, sudoite associated with magnesio-chlorite and low-substituted phengite occur in the quartz veins, whereas chloritoid is widespread in their metapelitic matrix. This corresponds to conditions of medium pressure (ca. 0.7 GPa), and low-to-medium temperature (300–380 °C). In the Beni Mezala-2 unit, magnesiocarpholite has been recognized as relics in chloritoid-quartz or kyanite-quartz veins, which is relevant to blueschist-facies conditions between 0.8 GPa, 380 °C and 1.3 GPa, 450 °C. Finally, in the Beni Mezala-1 phyllites, magnesiocarpholite relics in magnesiochloritoid-quartz veins, and talc-phengite assemblages in quartz-kyanite segregations testify to eclogite-facies conditions between 1.3–1.5 GPa, 450 °C, and 1.5–1.8 GPa, 550 °C. In the Benzu schists, the main paragenesis includes almandin–chloritoid high-Si phengite assemblages, which indicates minimum pressure conditions of ∼1.4 GPa at 550 °C during the peak of metamorphism.
The occurrence of late tremolite–talc and phlogopite–chlorite associations in Beni Mezala-1 unit points to a roughly isothermal unloading evolution down to ca. 0.8 GPa. Further exhumation under decreasing T is recorded by the growth of late paragonite, muscovite, chlorite, kaolinite, cookeite and margarite in Beni Mezala-1 and 2 units [1,68], together with epidote in the Benzu schists. Microstructural observations indicate that these retromorphic recrystallizations are coeval with the top-to-the-NNW kinematic indicators, which are widespread throughout the antiform [123,153,177].
3.3 Beni Bousera antiform
This antiform (Fig. 3B) is truncated by normal faults along its northeastern, Mediterranean side, but includes Permian–Triassic imbrications, similar to the Beni Mezala ones, along its western side. The uppermost imbrications roughly compare with the Tizgarine and Boquete Anjera units. On the contrary, the Permian metapelites of the lowest imbrication, labelled the Souk-el-Had (or South-Federico) unit, likely suffered a metamorphic climax under lower P/T gradient than its northern equivalent (Beni Mezala-1 unit), with peak conditions close to 550–600 °C and 1.2 GPa (Fig. 3C, bottom) documented by phengite–chlorite–phlogopite–kyanite associations, and late cordierite and andalusite growth [23,110].
The underlying Filali micaschists and gneisses are currently described as a single unit [53,86], although the presence of ductile low-angle normal faults is likely as in the equivalent Jubrique unit of the western Alpujarrides [10]. The uppermost Filali levels are made up of chlorite–chloritoid–muscovite ± biotite ± kyanite schists, grading downward to garnet–biotite–staurolite–kyanite schists and finally garnet–biotite–sillimanite at the transition with the gneisses (Fig. 3B). Andalusite is almost ubiquitous in the whole sequence and presents syn- to post-kinematic habits. The coexistence of the three Al-silicate polymorphs reveals the succession of a more or less complex recrystallization history. Microstructural relationships suggest that kyanite formed before (most of) the HT polymorphs [23,86]. Thus, early peak conditions estimated through the classical thermobarometres [23] would range from ca. 0.7 GPa, 580 °C in the garnet–staurolite–kyanite schists to ca. 0.8 GPa, 680 °C in the sillimanite–biotite schists and finally to ca. 0.8 GPa, 780 °C in the gneiss, being followed by roughly isothermal, then cooling decompression paths (Fig. 3C, bottom).
A major pressure gap occurs between the Filali gneisses and the underlying Beni Bousera unit, which includes granulite (‘kinzigites’) slivers on top of the ultramafics. The garnet–sillimanite ± kyanite–graphite–bearing granulite yielded peak conditions in the range 0.9–1.3 GPa, 800–850 °C [53,86]. However, a lensoid inclusion of metabasic granulites yielded a primary assemblage of pyrope-rich garnet, jadeite-rich pyroxene (Cpx I), kyanite, rutile, plagioclase, quartz, indicating peak conditions at 1.6–2.0 GPa, 760–820 °C (Ichendirene HP–granulite, [25]). Sapphirine–plagioclase symplectites after kyanite, and Cpx II-Opx-amphibole-plagioclase symplectites after garnet and Cpx-I point to a nearly isothermal early exhumation down to ca. 1.0 GPa, 700 °C.
Besides a thin serpentinite envelope (Fig. 3B), the upper part of the ultramafics is characterized by lensoid bodies of mylonitic, garnet–spinel-bearing peridotites, followed downward by foliated spinel-bearing harzburgites, then banded and foliated spinel–lherzolites [147,163]. The estimated conditions for the garnet-bearing mylonitic peridotites of Beni Bousera and their Betic equivalents at Ronda [172] are close to 1.8–2.0 GPa, 850–900 °C. Much higher temperatures and pressures are recorded within the ultramafic body itself, where corundum–garnet-bearing pyroxenites equilibrated at >20 GPa, 1200–1350 °C [87], whereas graphite pseudomorphs after diamond points to early pressures as high as 4.5 GPa [37].
The stretching lineations in the Souk-el-Had Permian phyllites, Filali schists and in the upper part of the underlying peridotites trend to the northwest, with top-to-the-north kinematic indicators coeval with the late andalusite growth during the exhumation tectonics [147,154]. A swarm of ENE- to east-trending dykes of cordierite–andalusite-bearing, anatectic leucogranite emplaced in the Beni Bousera unit during the same tectonic phase. A further stage corresponds to the juxtaposition of the Ghomaride upper plate units on top of the Sebtide antiforms through a ductile–brittle low-angle normal fault (Zaouia Fault [29,30]), which obliquely truncates the Permian–Mesozoic imbrications and upper Filali schists (see Section 4.3).
3.4 Minor Sebtide antiforms
From Beni Bousera to Cabo Negro, then to Ceuta (Figs. 2 and 5), the Sebtide antiforms along the coast display deeper and deeper levels of the metamorphic pile beneath the lowest Ghomaride, Aakaili nappe. This likely results from the same, major extensional fault than already observed on top of the Beni Bousera Sebtide units, i.e. the Zaouia Fault. The Cabo Negro antiform is essentially made up of schists similar to the ones of the deeper levels of the Filali unit. They are intensely affected by extensional shear zones. The Ceuta (Sebta) antiform shows two juxtaposed units, i.e., from top to bottom, a thinned equivalent of the Beni Bousera unit, with an upper, granulite (kinzigite) sliver over serpentinized peridotites, and a still deeper unit, made up of the Monte Hacho sillimanite-cordierite-bearing orthogneisses and marbles [86]. Top-to-the-north kinematic indicators are widespread in Hacho [153]. The latter unit is strictly equivalent with the Ojen unit of the Betic Cordilleras, at the bottom of the Ronda peridotites [10,169], which yielded eclogite-facies relics [168].
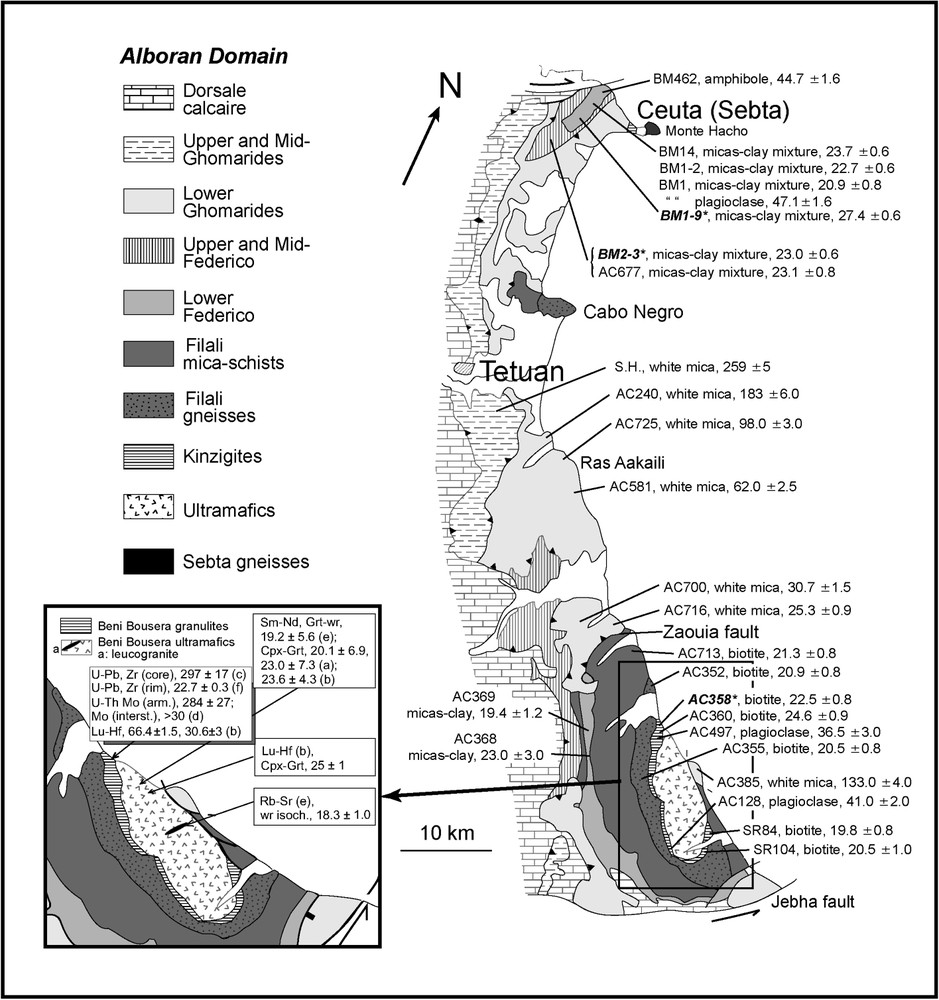
Isotopic datings of the Sebtide and Ghomaride units. Main map: KAr results, R. Montigny, analyst (wmm: white mica mixtures; bi: biotite), except SH date, S. Huon, in [111]. Asterisks indicate samples also dated by 40Ar–39Ar (Fig. 6). Inset: Beni Bousera multimethod datings. References: a: [90]; b: [14]; c: [156]; d: [121]; e: [138]; f: [134]. Mo (arm)/(interst.): monazite, armoured/interstitial.
Âges isotopiques obtenus dans les Sebtides et les Ghomarides. Carte principale : mesures KAr, analyste R. Montigny (wmm : mixtures de micas blancs ; bi : biotite), excepté SH : mesure de S. Huon, in [111]. Astérisques : échantillons datés par 40Ar–39Ar (Fig. 6). En cartouche : datations multiméthodes dans l'unité Beni Bousera. Mo arm/interst : monazite blindée/interstitielle.
3.5 Eastern Rif metamorphic allochthons
Three metasedimentary allochthonous units of controversial origin occur in the Temsamane area of eastern Mesorif, namely the Ras Afraou, Khebaba and Melilla-Tres Forcas allochthons (Fig. 4). An internal origin was suggested by Suter [162], based on their lithological characters which evoke that of the upper Federico units. Likewise, García-Dueñas et al. [65] describe in the Tres-Forcas allochthon the association of an upper, Ghomaride-type unit over a Sebtide-type unit with basal serpentinites. New petrographic studies [123] support the hypothesis of Internide outliers as these units display chloritoid-phengite-quartz assemblages which point to conditions around 0.6–0.8 GPa, 350–400 °C, similar to that of the Boquete Anjera unit. Additionally, 40Ar–39Ar dating on phengite suggests a minimum age of 20–23 Ma for the MP–LT episode [123], which also compares with that of the Federico units (see below). However, an origin from the distal part of the African paleomargin can be also hypothesized, as advocated by Frizon de Lamotte [60]. This alternative interpretation is consistent with (i) the lack of the expected Flysch slivers at the bottom of the metasedimentary allochthons, (ii) the occurrence of a minor ENE-trending suture zone within the eastern Rif external domain, documented by the Beni Malek serpentinites and greenschists close to the major Nekor fault [112], and the strong synmetamorphic deformation of the enclosing Ketama and northern Temsamane units, and (iii) the Late Oligocene–Early Miocene onset of the latter, oblique collisional deformation [33,60].

The metamorphic allochthons of the Temsamane region of eastern Rif: a sketch structural map, after [61,112,162]. Location: see Fig. 2.
Les lambeaux allochtones métamorphiques du Rif oriental (Temsamane) : carte structurale schématique d'après [61,112,162].
4 Dating the Sebtide–Alpujarride metamorphism
In this section, we first report on the stratigraphic and radiochronologic data from the Sebtide complex and overlying Ghomaride nappes. Additionally, we summarize the geochronologic dates obtained from the Alpujarrides, which usefully implement the Rifian dates.
4.1 Stratigraphic data
The oldest sediments that unconformably overlie the Rifian Internides, namely the Fnideq and Sidi Abdeslam Formations [33,57], are dated from the Late Oligocene–Aquitanian (23–20 Ma). These ‘post-nappe’ sediments were deposited onto the unmetamorphosed upper Ghomaride nappes, which means that cooling of the deeper, Sebtide metamorphic rocks may have lagged for some time after 23–20 Ma.
In western Betics, the Alozaina Formation above the Malaguide units is coeval with the Fnideq Formation [46]. Both these Aquitanian formations only contain pebbles from the Ghomaride–Malaguide and Dorsale units. By contrast, metamorphic pebbles from the Alpujarride units, and HP–LT minerals are observed in the Burdigalian deposits (20–16 Ma) of both the western and eastern Betics [46,94]. The gneissic pebbles found in the Fnideq-Alozaina (Viñuela) Formations [103] likely originate, together with the associated granite pebbles, from an uplifted Variscan domain similar to the Kabylian upper plate units (see Section 5). Therefore, the Sebtides–Alpujarrides units reached the surface at some time between 20 and 16 Ma.
4.2 Isotopic dating of the Sebtide rocks
Multimethod datings are now available in the Beni Bousera antiform (Fig. 5A–B). In the Filali schists, we obtained concordant K/Ar biotite ages comprised between and , with a mean value at . This is concordant with previous, KAr biotite and muscovite ages of and , respectively [97]. In the Beni Bousera granulitic envelope, we measured (R. Montigny, in [29,110,111]) KAr biotite ages ranging from to , with a mean value of , and a 40Ar–39Ar plateau age of . Consistently, a late leucogranite dyke crosscutting the lherzolite perpendicular to the dominant stretching direction yielded a whole-rock RbSr isochron age of [138].
Isotopic systems with high closure temperatures were also used in the Beni Bousera unit (Fig. 5B). SmNd ages were measured from garnet-whole rock pyroxenite at [138], and from clinopyroxene-garnet pairs at and [90], and [14], giving a mean age of . Blichert-Toft et al. [14] also measured a Lu–Hf mean age of on four samples from garnet pyroxene layers embedded in the peridotites, whereas a similar layer intercalated in the granulitic envelope yielded an age of , and a granulite sample a discordant age of . SHRIMP dating of zircon cores from the granulite-facies rocks yielded a preserved Variscan age, [156], whereas Platt et al. [134] obtained a on a zircon rim from the same rocks. Additionally, UThPb dating of monazite grains [121] yielded different ages according to the location of the grains in the granulite, i.e. a mean age of in nine grains perfectly included in garnet blasts, and variably reset ages between 170–30 Ma in the interstitial crystals.
The relatively cold, Upper Sebtide units of the Beni Mezala antiform yielded K/Ar [153] and 40Ar–39Ar ages (Fig. 5A) slightly older than that of the Souk-el-Had and Filali unit. In the Beni Mezala-2 unit, retromorphic white mica-clay mixtures yielded K/Ar results at and , and a 40Ar–39Ar plateau age of . In the Beni Mezala-1 unit, we measured from similar mica mixtures K–Ar ages of , , , and (mean age: ), and an integrated 40Ar–39Ar age of . The high-temperature Ar release of the reported 40Ar–39Ar analyses (Fig. 6) could result from inherited Ar content in the white mica from these metapelites or either reflect a higher pressure event. Additionally, ThPb and UPb ionic microprobe dating of retrograde monazite grains from the Beni Mezala-2 unit yielded mean ages of and , respectively, consistent with the KAr and 40Ar–39Ar data [83].
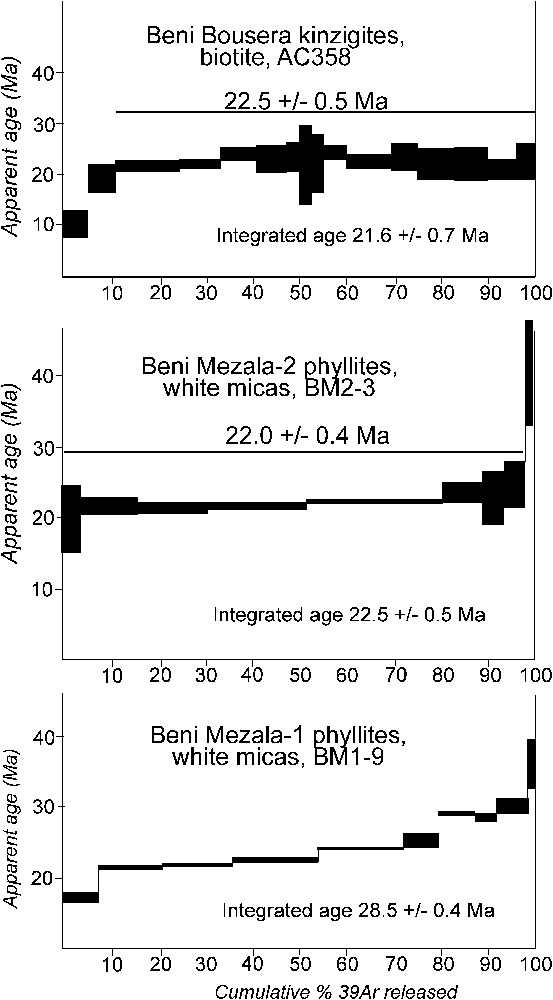
40Ar–39Ar analyses from the Beni Bousera kinzigites (A) and Beni Mezala phyllites (B). Analyst : R. Montigny. Localization of samples: see in Fig. 5.
Analyses 40Ar–39Ar de kinzigites de Beni Bousera (A) et de micas blancs des unités Beni Mezala (B). Analyste : R. Montigny. Localisation des échantillons : voir Fig. 5.
4.3 Alpine heating and resetting of the K/Ar chronometer in the Ghomarides
Within the lowermost, and thicker Ghomaride nappe (Aakaili), the K/Ar white mica ages increase with the distance to the Ghomaride–Sebtide contact (Zaouia Fault, Fig. 5A), from close to the contact to at Ras Mazari, 24 km northwest of Zaouia, i.e. ca. 2 km vertically above the Zaouia Fault [29]. In the Palaeozoic metapelites of the higher, Beni Hozmar nappe, the white mica fraction <2 μm yielded an age of (S. Huon, unpublished data in [111]). This age distribution suggests that the measured ages essentially result from downward increasing resetting of the Variscan micas in relation to increasing T, up to (closure temperature of white micas) close to the Zaouia fault, about 25 Myr ago [29,33]. New measurements of peak metamorphic temperatures in the Ghomaride rocks and underlying Sebtides by the Raman spectroscopy of carbonaceous material strongly support this interpretation. This study shows that the very base of the Aakaili nappe registered T close to 500 °C, i.e. about that of the upper Filali schists, and that the temperature decreases down to 330 °C in the uppermost levels [123].
4.4 Alpujarride dates and summary
Mica (biotite and muscovite) and amphibole KAr, 40Ar–39Ar, and RbSr ages from the western and central Alpujarride high-grade units display a striking convergence in the range 19–20 Ma, which suggest rapid cooling down to ca. 300 °C at that time [118,120,139,181]. Likewise, Sosson et al. [159] obtained 40Ar–39Ar laser-probe ages between 19 and 20 Ma on biotite and muscovite from high-grade gneisses of the Ojen nappe (at the bottom of the Ronda peridotites). The same result was also reported by Platt et al. [133] from 40Ar–39Ar isochrons yielded by muscovites and biotites from the Alboran Sea basement at ODP site 976, 50 km south of Malaga.
High-retentivity systems from the high-grade Alpujarride gneisses yielded both Alpine and pre-Alpine ages, consistent with the Sebtide results. A SmNd garnet-whole rock isochron age of was measured by Argles et al. [6], and interpreted as reflecting crustal extension during the Pangaea break-up. UPb SHRIMP dating of zircon yielded Hercynian ages in the Torrox gneiss (equivalent to the Monte Hacho gneiss), [180], and in the granulitic envelope of the Ronda peridotites, [156]. Studied by the same method, the inherited zircons from the garnet pyroxenites of the Ronda massif record a protracted mantle uplift evolution, referable to the Tethyan extension, from Early Permian () to Early–Middle Jurassic (), to Cretaceous (, ) times [156,157]. However, the rim of the studied zircons yielded recent Alpine ages, in the Ojen eclogite [157], and in the gneisses on top of the Ronda peridotites [132]. Likewise, SmNd isochrons at 22 ±2 Ma were obtained on Cpx–Grt pairs from Ronda pyroxenites, and referred to mineral pairs isotopically equilibrated during the Alpine metamorphism [145,146]. Therefore, in the Alpujarride and Sebtide high-grade units, similar dates ranging between 22–19 Ma are obtained from systems with a wide diversity of closure temperatures. This shows that the Sebtide–Alpujarride complex cooled down quickly during the Latest Oligocene–Early Miocene [120,132,135,157,181].
The age of the pressure peak have been tracked through 40Ar–39Ar dating of HP–LT phengites from low-grade, relatively cold Alpujarride units. Monié et al. [118] obtained a plateau age of on Si-rich phengite from a carpholite-bearing unit overlying the Sierra Nevada complexes east of Granada, and interpreted this result as the crystallization age of a late HP–LT phase, i.e. a minimum age for the peak of HP–LT metamorphism. This fairly compares with the KAr and 40Ar–39Ar ages reported above from the Beni Mezala HP–LT units (e.g., 23.7, 27.4, ). Ultimately, Platt et al. [136] obtained 40Ar–39Ar ages scattered between and Ma from low-grade, eastern Alpujarride units. These authors interpret the oldest ages, ca. 48 Ma, as the timing of the peak pressure, whereas the ages between 40 and 30 Ma would record the interplay between growth and early cooling of the fine-grained micas. The 48-Ma date could admittedly represent an Early Eocene thickening stage, at least in the eastern Betics. However, in view of the bias of the 40Ar–39Ar method in dating HP–LT polyphase mica grains (e.g., [7,38]), we assume that the oldest dates may be suspected to record the occurrence of excess Ar, and therefore we retain a conservative estimate of the timing of the pressure peak, relatively close to the timing of the temperature peak, i.e. between 25 and 30 Ma.
5 The Kabylides
In this section, we review the published, structural and data about the Kabylias and other North Algerian metamorphic massifs, and infer their fundamental similarity with the Rif Internides.
5.1 General
In Algeria, the Maghrebide Internides crop out in the Greater and Lesser Kabylia massifs, and in the smaller, Algiers and Chenoua massifs (Fig. 7). The position of these internal massifs within the Tell belt s.l. compares with that described in the Rif segment. In Lesser Kabylia (Fig. 8A), the Beni Touffout tectonic window shows that the crystalline massif is widely thrust over the Maghrebian Flyschs and the underlying External Domain. The Dorsale Calcaire units are stacked at the front of the crystalline massifs. In Greater Kabylia, the Maghrebian Flyschs also occur as large gravitary inliers (major equivalent to the Rifian Jebel Zemzem) on top of the Oligo-Miocene ‘post-nappe’ deposits that overlie the crystalline units [2]. The position of the easternmost Edough massif is controversial, as it was regarded alternatively as an internal or external massif (see Section 5.4). All along the Mediterranean coast, the nappe stack is intruded by calc-alkaline granites dated at ca. 16 Ma [107,127], and overlain by Langhian–Serravallian post-orogenic basins [2].
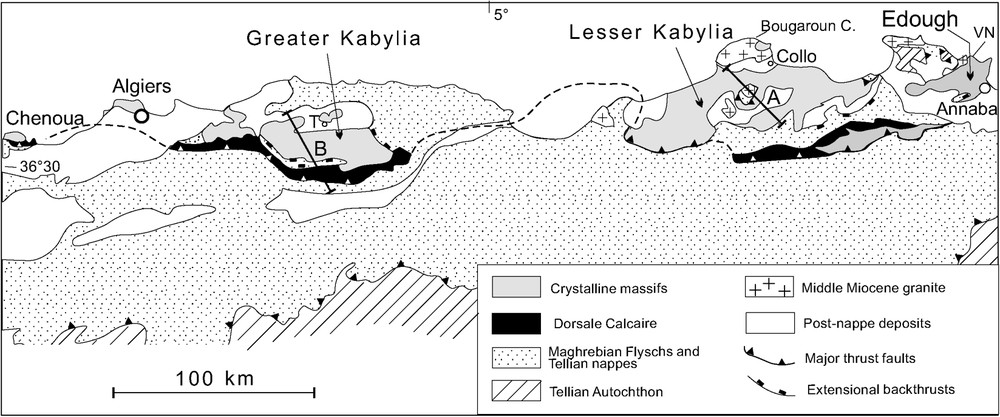
Sketch map of the Algerian Maghrebides showing the extension of the Kabylides, after [152], modified (see Fig. 1 for location). Abbreviations: T: Tizi Ouzou; VN: Voile-Noire.
Carte schématique des Maghrébides algériennes montrant l'extension des Kabylides, d'après [152], modifié (localisation sur la Fig. 1). Abréviations : T : Tizi Ouzou ; VN : Voile-Noire.

Cross-sections of the Kabylides (location: Fig. 7), and isotopic age data. A: Lesser Kabylia, after [99]. B: Greater Kabylia, after [152], with plot of the main multimethod isotopic dates after the literature (see text). Framed ages: UPb zircon: ellipses; RbSr: lozenges; 40Ar39Ar: squares.
Coupes des Kabylides (localisation sur la Fig. 7) et âges isotopiques. A : Petite Kabylie, d'après [99] ; B : Grande Kabylie, d'après [152], avec indication des principaux âges isotopiques d'après la littérature (voir texte). Zircon UPb : ellipses ; RbSr : losanges ; 40Ar39Ar : carrés.
5.2 The Kabylias: structure and P–T data
The Lesser Kabylia continental allochthon includes two major units, i.e. the Lower Unit (Beni Ferguen nappe, [18]), which displays the higher-grade metamorphism, and the Upper Unit, with lower grade, and partly fossiliferous rocks. Likewise, Greater Kabylia is divided into a low-grade Upper Unit and a high-grade Lower Unit, the intervening contact being interpreted as a major detachment fault, labelled the Kabylian Detachment Fault (KDF) [152]. In the following, these major Kabylian units are referred to as the Upper and Lower Plates.
On top of the Kabylian Upper Plate, a well-dated Cambrian to Carboniferous sequence [12,20] is locally preserved beneath the Oligo-Miocene onlap. A Late Devonian (Eovariscan) phase is responsible for the greenschist-facies metamorphism of the pre-Carboniferous layers, whereas a Late Carboniferous (Hercynian) phase is responsible for the folding of the unconformable, Lower Carboniferous deposits. In the Chenoua massif, similar Devonian–Carboniferous terranes are unconformably overlain by Triassic redbeds [93]. Therefore, the uppermost crustal sequence of the Kabylian Upper Plate compares with the Ghomaride–Malaguide one. However, contrary to the latter, the Kabylian Upper Plate also involves a thick Phyllite sequence, at the bottom of the unconformable Cambrian conglomeratic layers [12]. Moreover, in Lesser Kabylia, the Upper Plate includes peraluminous granites emplaced at 270–280 Ma [130], and low-pressure metapelitic assemblages (garnet, biotite, cordierite, sillimanite) associated with their emplacement [99].
The Lesser Kabylia Lower Plate includes an upper subunit, made up of ortho- and paragneisses, and a lower subunit, which essentially consists of mica-rich graphitic schists with accessory calc-silicate layers, amphibolites and orthogneisses (Fig. 8A). Mahjoub et al. [99] recognize an early HT–LP mineral association quartz-muscovite-biotite 1-garnet 1-plagioclase ± post-kinematic andalusite (M1), followed by a higher pressure assemblage (M2) with more magnesian biotite 2 and garnet 2, kyanite (formed at the expense of andalusite), staurolite and rutile. M1 can be ascribed to a late Hercynian event, whereas the M2 event would correspond to an early Alpine thickening event (see below). The Greater Kabylia Lower Plate exposes a ca. 8-km-thick metamorphic pile that includes [152], from top to bottom, (i) micaschists with marbles, metarhyolites, amphibolites and metagabbros; (ii) orthogneisses derived from igneous bodies intruded within the micaschists (metamorphic contacts with chiastolite and cordierite); (iii) sillimanite-bearing, anatectic paragneisses; (iv) marbles, up to 1-km-thick, with HT minerals such as forsterite, diopside and phlogopite; and finally, (v) a deep unit of orthogneisses. The metamorphic rocks are locally crosscut by granites and pegmatites. In the case of the Sidi Ali Bou Nab granite of North Greater Kabylia, the pelitic hornfelses on top of the granite display HT–LP assemblages (biotite, chiastolite, cordierite or sillimanite), whereas in a mylonitic shear zone at the bottom of the granite (Fig. 8B), synkinematic kyanite and garnet replace the LP minerals.
Last but not least, it is worth noting the occurrence, in Lesser Kabylia, of a large peridotite massif, belonging to the polymetamorphic Lower Plate, but completely surrounded by the Cap Bougaroun Miocene granite. The ultramafic massif is quite similar to the Beni Bousera one, and mainly consists of foliated spinel-lherzolite with secondary overgrowth of plagioclase around spinel grains, and includes pyroxenite layers [19]. The ultramafics are associated with some granulites (‘kinzigites’) and calc-silicate layers.
5.3 Dating the Kabylian metamorphism
As reported above, Eovariscan metamorphism occurred in the Upper Plate, followed by Variscan granite emplacement and associated metamorphism. Consistently, 40Ar–39Ar plateau ages of 314 Ma on white mica, and 295 Ma on green biotite were measured in the (Late Proterozoic–Early Cambrian?) phyllite sequence [117]. By contrast, varied ages, ancient as well as recent, have been measured during the last couple of decades in the Greater Kabylia Lower Plate and Algiers massifs, which fueled warm controversies [17,117,128,129]. These ages (Fig. 8B) can be interpreted, even in the absence of Permian–Mesozoic formations, in the frame of a polymetamorphic, pre-Alpine and Alpine evolution of the deep Kabylian crust.
Panafrican UPb zircon conventional ages have been measured from the deepest orthogneisses of Greater Kabylia, i.e. 510 and 514 Ma [17]. Variscan ages were obtained by the same method from the Sidi Ali Bou Nab granite, (J.-J. Peucat, unpublished results, quoted in [152]), and from the associated mylonitic shear zone, [129]. The muscovite and muscovite-K felspar pair from Sidi Ali Bou Nab metapegmatites also yielded RbSr ages of 250 and 258 Ma, respectively [129], thus confirming a Variscan emplacement of the granite, and of its early deformation. These ages contrast with 40Ar–39Ar plateau ages of 25–26 Ma obtained on muscovite and biotite from the mylonitic kyanite–sillimanite-bearing gneisses beneath the granite [117]. In fact, the scatter of the 40Ar–39Ar results from the Tellian Internides compares with that observed in the Sebtide–Alpujarride units, as it ranges from 126 Ma to 25 Ma [117], or from 214 Ma to 40 Ma in a small area of East Greater Kabylia [34]. Moreover, frequent sigmoidal shape or saddle shaped depressions of the 40Ar–39Ar release spectra attest either to the incomplete resetting of presumably Variscan micas [117], or to the occasional incorporation of excess argon. Therefore, we consider that the hypotheses of high-temperature tectono-metamorphic events at 80 Ma [117,152] or 128 Ma [34] are not adequately demonstrated by the available isotopic data yet. We rather suggest that the Tellian Internides were affected by about the same 25–30-Myr-old metamorphic event than the Sebtide–Alpujarride units.
5.4 The Edough massif
In this dome-shaped massif, two stacked tectonic–metamorphic complexes are shown, separated from each other by a major detachment fault [26]. The lower complex, referred to as the Edough Lower Plate hereafter, mainly consists, from bottom to top, of migmatitic paragneisses and orthogneisses with kyanite–sillimanite–andalusite-bearing micaschists and marbles (‘Lower Unit’ in [66,119]), followed upward again by aluminous, andalusite–sillimanite-rich micaschists intruded by a meta-leucogranite in the northern part of the massif (‘Upper Unit’ in [66,119]), whereas they are free of such an intrusion and of andalusite–sillimanite minerals south of the massif, where they display staurotide–kyanite–garnet–rutile-bearing assemblages [26]. This Lower Plate also includes peridotites and metabasites, in a small outcrop within (below?) the migmatitic gneisses [16], on the one hand, and in the Voile Noire sliver on the other hand [27,119]. The ultrabasites are serpentinized harzburgitic tectonites associated with retromorphic eclogites and (Voile Noire sliver) ‘kinzigites’, similar to that of the Lesser Kabylia and Sebtide–Alpujarride units. The Voile Noire sliver shows granulite-facies assemblages, whereas the (overlying) micaschists units record peak conditions close to 1.2–1.4 GPa, 550–600 °C [27]. Remarkably, some quartzitic layers (metaphtanites) interleaved within the metapelitic schists north of Annaba yielded some Acritarchs fossils dating the protoliths from the Ordovician–Devonian [82].
By contrast, the Edough Upper Plate is mainly made up of low-grade greenschist-facies phyllites with Triassic (?) marbles, locally overlain through a calc-mylonite level by very-low-grade, fossiliferous Jurassic marbles and Cretaceous calcschists and turbidites [26]. The peak conditions here do not exceed 0.3–0.4 GPa, 350–400 °C, which suggests an important extensional movement between the upper and lower plates [26,27]. This movement occurred top-to-the-NW, as indicated by the widespread, late metamorphic kinematic indicators observed from the migmatites to the micaschists, to the Mesozoic meta-sediments themselves [26,66].
As for the isotopic datings, they yielded contrasted ages, according to the method used and/or to the sampled unit. UPb dating of idioblastic zircon separates, one from the gneiss dome and the other one from the overlying micaschists, yielded ages of and , at the Concordia upper intercept, and and at the lower intercept, respectively [77]. This suggests the occurrence of a Panafrican crust, affected by a Tertiary thermal event. SmNd garnet-whole rock pairs from the aluminous micaschists yielded an Hercynian age, , whereas recent ages, and , were obtained from the gneiss dome. Likewise, plateau ages of and were obtained on amphiboles from the retromorphic eclogites beneath the gneiss dome, whereas the micas from the gneisses and micaschists yielded plateau ages grouped around 16–17 Ma [119].
To conclude this section, we should emphasise the striking similarities between the Edough and Kabylian lithologies (both crustal and mantellic), metamorphic structure (upper and lower plate separated by a detachment), conditions (up to granulitic and/or eclogitic in the lower plate), and isotopic ages (Precambrian relics, strong Variscan imprints, and Late Oligocene–Aquitanian dates). This seems in contradiction with the alleged external position of the Edough massif [175], and with its origin from the African margin instead of the Alkapeca terrane [27]. We observe that, (i) the Numidian flysch directly overlies the external Cretaceous series northwest of the massif, which suggests that the poorly exposed contact of the flysch nappe over the boundary of the crystalline massif is an out-of-sequence fault; (ii) the low-grade Mesozoic sediments of the Upper Plate are either of Dorsalian–Predorsalian origin (i.e. internal), or more probably backthrust from the Tellian (external) domain; and (iii) the Numidian klippe observed on top of the Upper Plate units also emplaced through back-sliding from a more external position during the Early Miocene, as it occurred in the Kabylias [2,43] and Rif [33]. If correct, the Edough massif actually belongs to the Tellian Internides, as early assumed by Hilly [80], and more recently by Mahjoub et al. [99].
6 Discussion and conclusion
6.1 The Maghrebide Internides: a disrupted, extensional metamorphic complex
The Maghrebide Internides display a remarkably constant metamorphic structure involving an upper plate, virtually unaffected by Alpine recrystallizations except at its very base (Section 4.3), and a lower plate that generally consists of normally ordered, low- to high-grade units. Both plates are separated from each other by major detachments, i.e. the Zaouia Fault (Section 3.3) and Kabylian Detachment Fault (Sections 5.2, 5.4), which operated during the Latest Oligocene to Middle Miocene times as their Betic equivalents [8,64,95,104,126]. The upper plate includes Late Proterozoic (Kabylias)-Palaeozoic metasediments affected by Eovariscan HT–LP metamorphism, then deformed again and intruded by calc-alkaline granites during the Variscan orogeny (Kabylias). On top of the plate, or detached at its front in the Dorsale Calcaire, Triassic–Eocene sediments occur, followed by synorogenic clastics and olistostromes of Oligo-Miocene age. The lower plate includes Permian–Triassic metasediments (at least in the Sebtide–Alpujarride units), and a polymetamorphic continental material locally associated with infracontinental mantle units and granulite slivers (Beni Bousera-Ronda, Cape Bougaroun, Edough). The deepest gneisses from Greater Kabylia and Edough yielded UPb Panafrican ages, whereas UPb and SmNd Variscan dates were obtained from granulites (Beni Bousera), granites and mylonites (Greater Kabylia) and micaschists (Edough). Alpine metamorphism evolves from HP–LT gradients, still recorded in the lower-grade metasediments of northern Rif, to intermediate pressure gradients recorded in the higher-grade schists of northern Rif and Kabylias, to HT–LP gradients superimposed to the previous ones during decompression. Alpine peak conditions correspond to that of the eclogite facies, ∼1.6 GPa, 550–600 °C (Sections 3.2, 3.3), and likely occurred at about 30–25 Ma (Sections 3.4, 4.4, 5.3). Rapid cooling from 600 °C, or ∼700 °C in the deepest units, to ca. 300 °C occurred between 22–20 Ma.
6.2 Interpretation: competing models and unsolved questions
The Betic–Maghrebide orogen was interpreted either as (i) a collisional orogen, unrelated to any subduction zone [121,131,171], or (ii) an orogen related to a northwest-dipping subduction zone extending from Gibraltar to the Apennines, and operating from the Late Cretaceous onward [5,8,84,91,96,161,179]; or else, (iii) an orogen involving two subduction zones, i.e. a Late Cretaceous–Eocene, southeast-dipping Alpine-Betic subduction, and an Oligocene-Neogene, northwest-dipping Apenninic–Maghrebide subduction [32,36,41,42,62,73,74,115,144,182]. Dercourt et al. [40] assume that two subduction zones operated east of Corsica and Calabria, while only one north-dipping subduction would have operated along the southern margin of a Kabylia–Alboran block. Maldonado et al. [101] retain the occurrence of two opposite subductions dipping beneath the Alboran Domain, but assume that the youngest ‘subduction’ only concerned the delaminated, lower African lithosphere. Only the subduction-related models can account for the occurrence of blueschist- and eclogite-facies metamorphism reported above from the Maghrebide lower plate units (Beni Mezala; Section 3.1), and from the underlying complexes to the west, i.e. the upper Nevado-Filabride complex of central–eastern Betics [8,69,141–143,166], and to the east, i.e. in the ophiolite-bearing units of Calabria (see [150], with references therein).
The ‘one-subduction’ and ‘two-subduction’ competing models differ in the palaeogeographic interpretation of the Maghrebian, Betic and Peloritan-Calabrian continental terranes, which they ascribe either to the Iberian paleomargin (Fig. 9A–D), or to an Alkapeca (Mesomediterranean) intra-oceanic continental plateau (Fig. 9E–H), respectively. In support of the latter hypothesis, Michard [111,115] and Chalouan and Michard [32] emphasize that (i) the Triassic–Liassic sequences of the Ghomaride-Malaguide and Dorsale nappes strongly contrast with that of Morocco, southern Iberia, Sardinia, Corsica, and Briançonnais, and, on the contrary, compare with that of southern Apulia, Tuscany and Austroalpine areas, and (ii) the Eovariscan–Variscan evolution of the Alkapeca basement resembles that of eastern Morocco and Adria, rather than of southern Sardinia and eastern Iberia [29]. In particular, it is worth noting that the Sardic phase, responsible for tectometamorphic and magmatic events at ca. 450 Ma in Corsica–Sardinia [78,92] and the Briançonnais basement of Western Alps [13,75] is not known in the Alkapeca terranes. By contrast, the southern Calabria basement allochthon exposes a tilted cross-section of the Variscan continental crust, which demonstrates striking similarities with that of the Ivrea Zone of the Alps [71,72]. Therefore, Alkapeca could be a larger equivalent of the Sesia–Dent Blanche block of western Alps, drifted away from the Adriatic margin (lower plate of the asymmetric Tethyan rift) during the Jurassic [102]. Such an origin is consistent with the occurrence of granulites and peridotites in the basement of the Sesia and Ivrea zones in the Alps, and in the Alkapeca-derived blocks, while they are lacking in the Briançonnais-Dora Maira units of European (upper plate) origin [113]. Consistently, UPb high-resolution datings of zircon at 183–131 Ma in the Ronda ultramafics point to their early exhumation being related to the Pangea break-up [156], coeval with the Ivrea ultramafic uplift [158,161].
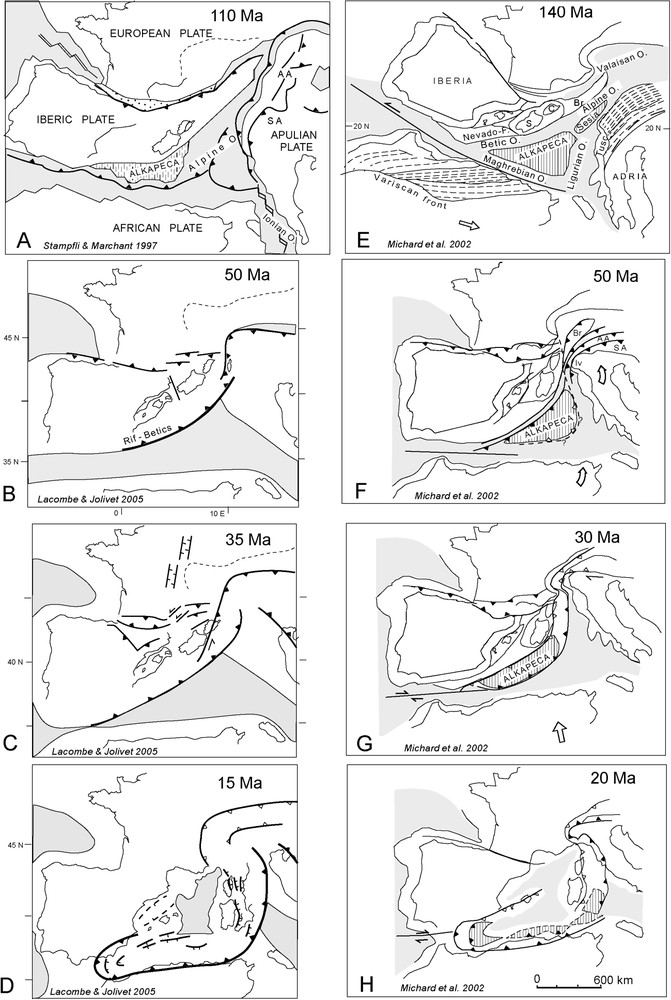
Alternative scenarios for the geodynamic setting of the Maghrebide belt from Early Cretaceous to Early–Middle Miocene. Left (A–D): two interpretations of the ‘one-subduction model’ (stage A: after [161]; stages B–D: after [91]). Right (E, H): the ‘two-subduction model’, after [115]. In stage F, the hypothesis of an early development of the Apenninic subduction south of Alkapeca is shown after [41]. See text for discussion.
Scénarios alternatifs interprétant le cadre géodynamique de la chaîne des Maghrébides du Crétacé inférieur au Miocène inférieur–moyen. À gauche (A–D) : deux variantes du modèle à une seule subduction (stade A d'après [161] ; stades B–D d'après [91]). À droite (E, H) : modèle à deux subductions, d'après [115]. Au stade F, l'hypothèse d'un développement précoce de la subduction apenninique au bord sud d'Alkapeca est tirée de [41]. Voir discussion dans le texte.
The ‘one-subduction’ model implies a subduction flip changing the southeast-dipping Alpine subduction into a northwest-dipping Apenninic subduction, either between the Ligurian Alps and Corsica [140], or south of Corsica (Fig. 9A, B). However, Lacombe and Jolivet [91] assume that two opposite subductions (Alpine, then Apenninic) operated successively on the Corsica transect (Fig. 9B, C) with a single, Apenninic = Ligurian–Maghrebian subduction prevailing more to the southwest. As far as Corsica is concerned, this is consistent with the structural and metamorphic homologies between Ligurian Alps and eastern Corsica [44,100], and with the deep seismic data across the north-Tyrrhenian–Adriatic profile [59]. But, once admitted that two subductions have operated successively in the Corsica–northern Apennine transect, there is no definitive reason to discard the hypothesis of the Alpine subduction going on southwest-ward up to the Azores–Gibraltar plate boundary. In that case, the Alpine (or Alpine–Betic) subduction would be responsible for the consumption of an Alpine–Betic oceanic gateway west of the Alkapeca plateau (Fig. 9F), and for the Nevado-Filabrides HP–LT metamorphism, whereas the Apenninic-Maghrebide subduction would account for the closure of the Maghrebian ocean, and for the high- to intermediate-pressure metamorphism of the Alpujarrides–Maghrebides. Dèzes et al. [41] suggest that the Apenninic subduction could have been initiated as early as the Late Palaeocene (Fig. 9F, dashed line). Indeed, the age constraints reported above from the Alpujarrides–Maghrebides suggest peak conditions around 30–25 Ma. The dates obtained from the Apennines and Calabria [85,150] also suggest a Late Oligocene HP–LT event, consistent with the age of the Apenninic subduction as inferred from the associated back-arc extension [62,96,106,144,151,173] (Fig. 9G and H). On the other hand, the available isotopic datings from the meta-ophiolite-bearing, upper Nevado-Filabride complex (Mulhacen Complex, [142]) include 39Ar/40Ar ages scattered between 90–15 Ma [38,39,118], and SmNd ages of and [142]. Augier et al. [8] infer from in situ laser 39Ar/40Ar dating on phengite that the peak of pressure should be around 30 Ma or older. These various data suggest, although ambiguously, an early, Late Cretaceous?–Eocene HP–LT evolution overprinted by the regional Early Miocene event. If correct, the Apenninic-Maghrebide subduction would postdate the Alpine–Betic subduction up to the Betic–Maghrebide transect. Remarkably, and following the proposal of Doglioni et al. [42], the Oligocene–Neogene subduction would continue southwestward the backthrust zone that formed in the western Alps during the collisional stage [116,158].
Final building of the Maghrebide Internides depends on two coeval geodynamic processes, the discussion of which is beyond the scope of this paper, i.e. the retreat of the Apenninic–Maghrebide subduction, and the Africa–Iberia convergence (see [8,55,62,91] for details and references). Subduction retreat is responsible for the Oligo-Miocene opening of the Alboran and Algerian basins [31,35,106], and triggered the operation of major extensional detachments such as the Zaouia Fault (Section 4.3), the Kabylian Detachment Fault (Section 5.2), and their equivalents observed throughout the Betic pile. Disruption of the Eocene tectonic prism, block migration and block rotations [28,58,88,137] resulted from the subduction retreat process. Being broadly contemporaneous with the ongoing plate convergence, the retreat-related extensional events should have alternated with contractional ones, both onshore [10,33] and offshore [31]. These combined geodynamic processes eventually resulted in the formation of the orogenic arcs at the eastern and western tips of the Maghrebides, and for their fast rollback towards the free boundaries (Atlantic and Ionian, respectively) [106]. In particular, the Betic–Rifian (Gibraltar) Arc coincides with the complex triple junction [115] between the vanishing Alpine–Betic subduction, the active Apenninic–Maghrebide subduction, and the Azores–Gibraltar transform zone, converted into a collisional zone between Iberia and Africa since Chron 18 (36 Ma) [70,149]. Compelling evidence for an active east-dipping subduction beneath the Alboran basin, with its accretionary prism in the Gulf of Cadiz, are gained from seismic, gravimetric and tomographic surveys [76,101,108,122,160].
To conclude, the constraints from the Maghrebide Internides and western Betic Cordilleras (Gibraltar Arc) would be better accounted for by a ‘two-subduction’ geodynamic model, rather than by a ‘one-subduction’ scheme, in the framework of the Mediterranean Alpine belts. However, we must emphasize that important questions have to be answered yet before reaching a definitive model, such as the original palaeogeography of the postulated Alkapeca intra-oceanic plateau, the original location of the Nevado-Filabride complexes with respect to Iberia and Alkapeca, the timing of their HP–LT metamorphism, the age of peak pressure in the Alpujarride–Sebtide and equivalent Kabylian units (30–25 Ma or older?), and that of the thrusting of the Alpujarride–Malaguide metamorphic complex above the seemingly older Nevado-Filabres complex.
Acknowledgements
Field and laboratory works were supported by the CNRS–INSU, Programme DBT 5, the ‘Coopération inter-universitaire franco-marocaine’, A.I. 93/629, 98/161 and MA1999, and the ‘Programme d'appui à la recherche scientifique’ (Maroc), S.U. 26. We are gratefully indebted to Victor García-Dueñas, Paco González-Lodeiro and the numerous colleagues from Granada who guided us in the Betics. We are also indebted to Carlo Doglioni, Claudio Faccenna, Michel Durand-Delga, and Dominique Frizon de Lamotte for constructive criticism of early drafts of this paper.