The understanding of the differences in the electrical characteristics of continental and oceanic thunderstorms (i.e. differences in the lightning production and consequently in the values of the thunderstorm cloud electrification rate) is an important condition for the understanding of the global electrical circuit mechanisms. Two different hypotheses have been advanced in order to explain these differences. The first hypothesis (thermal hypothesis) has been formulated by Williams [1,6]. It considers that the surface wet-bulb temperature, well correlated to the convective available potential energy (CAPE) and consequently to the dynamic thunderstorm cloud characteristics, is a preferred parameter for assessing changes in cloud electrification. This hypothesis attributes the differences in the electrical characteristics of continental and oceanic thunderstorm clouds and consequently of the corresponding electrical flash frequencies to the land and sea temperature and humidity differences. The second hypothesis (aerosol hypothesis) has been advanced by Molinié and Pontikis [4,5] and Michalon et al. [8]. This hypothesis attributes the differences in the electrical characteristics of continental and oceanic thunderstorm clouds to the land and sea differences in the sub-cloud layer aerosol. The aerosol hypothesis clearly states that low aerosol and CCN concentrations in the sub-cloud layer lead to early formation of warm precipitation, thus inhibiting the cloud vertical velocities and the large droplet and ice-crystal concentrations in the mixed phase region of maritime cumulonimbi. This behavior decreases the cloud electrification rate and consequently the corresponding electrical flash frequency. In contrast, due to the high aerosol and CCN concentrations in the sub-cloud layer of continental regions, continental cumulonimbi show the opposite behavior, with high small and large droplet and ice crystal concentrations in the mixed phase layer and reveal high electrification rates and corresponding electrical flash frequencies.
Earle Williams and Sharon Stanfill (hereafter referred to as WS) [10] present a “critical re-examination of the thermal and aerosol hypotheses”. This re-examination consists of three arguments presented respectively in paragraphs 2–4, and 6 of their paper. However, the paper's conclusions are unconvincing and this situation deserves some comment.
The first argument is extracted from the study of the behavior of the annual thunder-day number (ATD number) observed at given islands as a function of the corresponding island area S (Fig. 4 in WS and Fig. 1 in this paper), whereby a thunder day corresponds to a day during which at least one thunder has been heard. By using a very rough procedure, WS first deduce two characteristic island radii. The first radius is supposed to be representative of a limit island area value (110 km2) above which the thermal oceanic air-mass characteristics are more or less shifted towards the continental characteristics, while the second is supposed to represent the limit area value (20 000–30 000 km2), above which the corresponding oceanic aerosol concentrations in the sub-cloud layer are more or less shifted towards the continental aerosol concentrations. Secondly, the ATD numbers observed in the vicinity of various islands are plotted versus the corresponding island areas. According to WS, “despite considerable scatter over all, Fig. 4 does show clear evidence for a transitional area in the range of 102–103 km2 between oceanic and continental thunder-day behavior”. And further “this result is decidedly closer to the prediction for a thermal perturbation (110 km2) than an aerosol perturbation (20–30 000 km2)”, thus supporting the traditional hypothesis for the land-ocean lightning contrast. However, in Fig. 2, where the ATD numbers are plotted versus the corresponding island elevations h, one may observe a similar trend in the data points as in Fig. 1, which could be interpreted as the existence of a transitional island elevation in the range of 400–1000 m between the so-called oceanic and continental ATD behavior. For islands with elevations lower than 1000 m, 65% of them have ATD numbers lower than 20, while above this height, 87% of the islands have ATD numbers larger than 20. This introduces an important ambiguity in WS's conclusion of the existence of a critical area. Further, Fig. 3 presents the island elevations plotted against the island areas and reveals the absence of islands higher than 1000 m with areas lower than 600 km2. This absence is, most probably, at the origin of an artifact (discontinuity) in the ATD number-vs-S plot, interpreted by WS as denoting a transitional area from oceanic to continental thunder-day behavior. Note, however, that the existence of a critical island elevation would more attest for the role of the island relief forcing in the enhancement of pre-existing deep convection rather than for the role of local thermal anomalies in the development of local deep convection. This is also supported by our observations in Guadeloupe and Martinique (F.W.I.), suggesting that all thunder days observed there are related to pre-existing deep convection, enhanced by the island's relief, corresponding to the passage of large and meso-scale perturbations (easterly waves, tropical storms and Intertropical Convergence Zone debris) and not to local thermal convective developments. Further, the aerosol hypothesis has been advanced in order to explain differences in the lightning production of continental and oceanic thunderstorms. Consequently, the use of the ATD number is inappropriate, since it is an index of annual thunderstorm occurrence and not an index of thunderstorm cloud electrification (a thunder cloud producing one or more than one thunder), thus not allowing any conclusion in relation to the lightning frequency and in extension to the corresponding thunderstorm cloud electrification rate. As an example, in Sumatra and Guadeloupe, respectively 53 and 52 ATD are observed (Table 1 in WS), whereby Sumatra's area is roughly three orders of magnitude larger than the Guadeloupe area. In contrast, according to the LIS flash rate density (Fig. 1 in WS), the annual lightning flash number in Sumatra is one order of magnitude larger than in Guadeloupe, thus attesting for the absence of a clear relation between electrical flash frequency and ATD number. It appears therefore that the main conclusions of § 2 are based on both an incomplete analysis and the use of an inappropriate index.
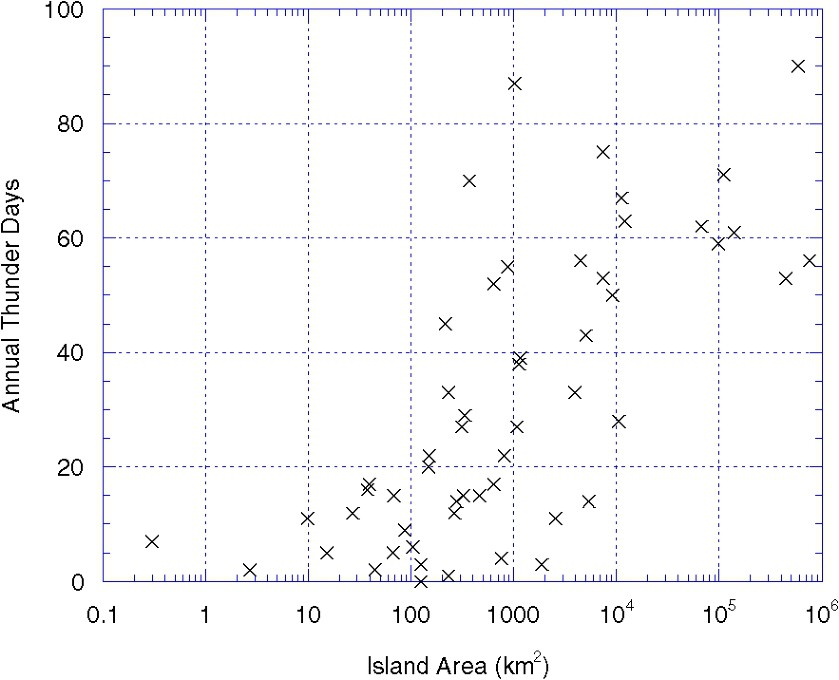
Annual number of thunder days versus island areas.
Nombre annuel de jours d'orage en fonction de la surface des îles.
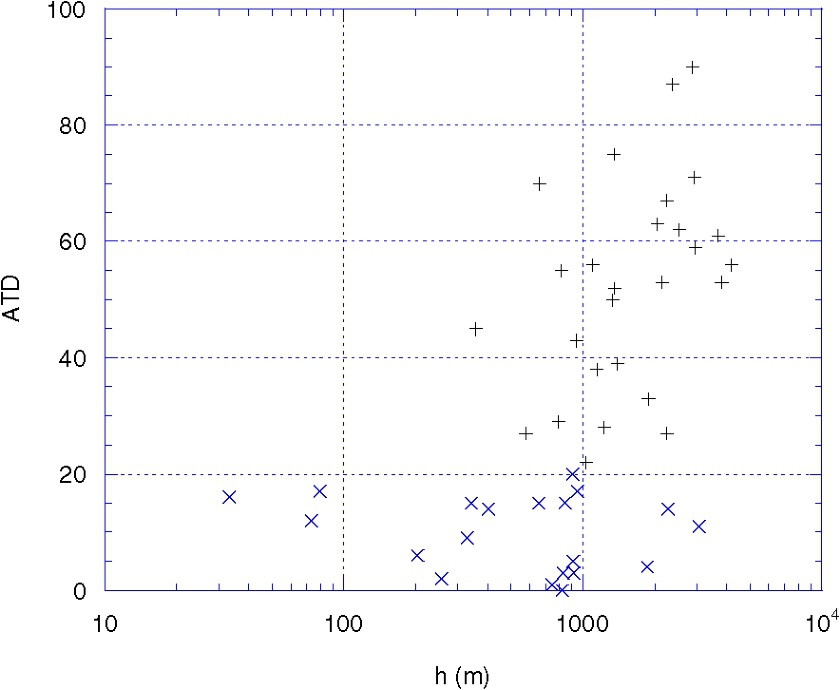
Annual number of thunder days versus island elevations (x: ATD<20; +: ATD>20).
Nombre annuel de jours d'orage en fonction de l'altitude des îles (x : ATD<20 ; + : ATD>20).
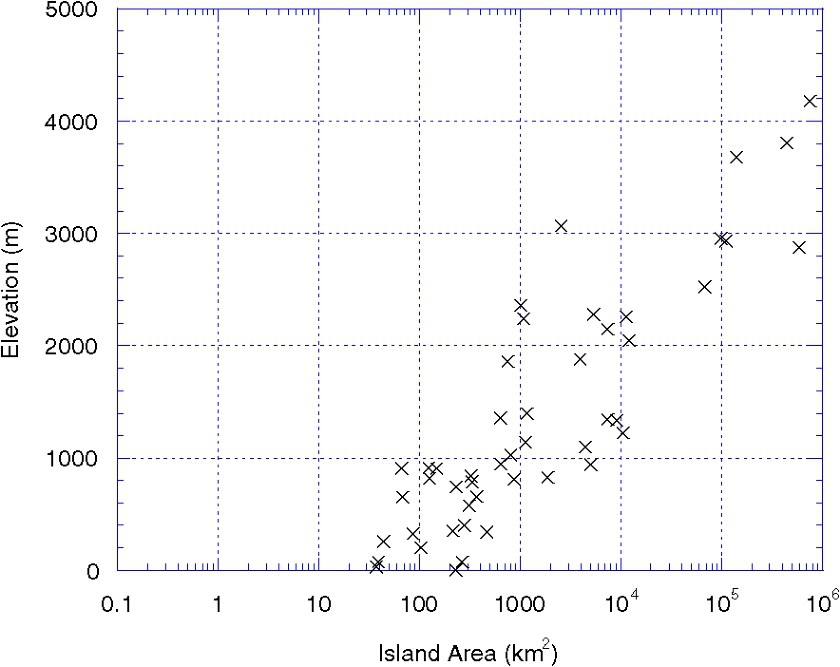
Island elevations versus island areas.
Altitude des îles en fonction de leur surface.
The second argument concerns the land–ocean contrast in lightning activity as related to warm-rain production. WS argue that similarly to the aerosol hypothesis, “the traditional thermal hypothesis for the land–ocean lightning contrast also carries with it a qualitative prediction for the land–ocean contrast in warm rain production… Following the work of [6] and others, the larger updrafts over continents […] will allow less time for droplets to interact for coalescence, and may therefore forestall the formation of warm rain.” The latter assertion constitutes the inversion of Jorgensen and Lemone's [9] remark formulated for oceanic clouds that in such clouds “…the time scales for air to rise from cloud base to the freezing level are in excess of 30 min, allowing ample time for warm-rain processes to produce precipitation […]”. It is clear that this only occurs under the condition of previous development of precipitation embryos [3] with radii larger than 20 μm. Below this value, the warm rain formation processes (collision–coalescence and break-up) are inefficient. Oceanic clouds with low droplet concentrations N are capable of developing precipitation embryos in the warm region below the freezing level, thus allowing the initialization of rain in this region. For example, an adiabatic ascent of 1500 m of a cloudy air parcel in a tropical environment is sufficient for developing precipitation embryos. Continental clouds with high droplet concentrations (as from roughly ) are incapable of developing precipitation embryos in the above-mentioned region, thus not allowing the initialization of warm rain below the freezing level. In other words, continental convective clouds, even with low vertical velocities, would most probably be incapable of producing warm precipitation. Consequently, the conclusion advanced by WS in § 4 of the commented paper that the thermal hypothesis also provides a qualitative explanation for the land-ocean contrast in warm rain is incorrect.
The last argument presented by WS in favor of the thermal hypothesis is based on the reference of a case study that contrasts with the ‘green ocean regime’ during the ‘wet-to-dry-season’ transition in Rondonia [2]. However, the corresponding daily CCN and CN concentrations (respectively of 470 and 800 cm−3) for this case were both higher than the usual concentrations observed in oceanic air masses (less than 300 cm−3) [7] and higher than the above-mentioned concentration cut-off value for cloud electrification. Consequently, the observed contrast is more consistent with the aerosol hypothesis than with the thermal hypothesis. Note also that, in tropical regions, during the transition and wet regime, the frequent rainfalls wash out the air masses and shift the sub-cloud layer aerosol concentrations towards the oceanic concentrations at time scales shorter than one day, thus attenuating the significance of mean daily CCN and CN concentration measurements. As a consequence, WS's last argument fails to strengthen the thermal hypothesis.
To conclude, greater care is needed in selecting indices in favor of the thermal or aerosol hypotheses and in quantifying relationships between these indices and cloud electrification. It is clear that many questions relative to the validity of the hypotheses advanced in order to explain the land–ocean contrast in lightning activity remain still unanswered and that further investigations are necessary in order to give them plausible answers. Note, however, that the thermal instabilities can be considered as an index of convective cloud development representative of the dynamic convective cloud characteristics and not as a cloud electrification index. The link between the dynamic cloud characteristics and cloud electrification is made by the microphysical cloud characteristics that are principally driven by the cloud droplet concentration and consequently by the sub-cloud layer aerosol concentration. Most probably, both hypotheses hold and are complementary. Further, any critical re-examination of the validity of these hypotheses should be based on analyses of the simultaneous behavior of the meteorological convection indices (e.g., temperature, wet-bulb temperature, equivalent potential temperature, CAPE, sub-cloud layer aerosol concentrations, etc.) and of the cloud electrification indices (e.g., electrical flash frequency, flash rate density, etc.). A final step could be the use of flash frequency parameterizations based on these hypotheses in connection to appropriate GCMs and/or meso-scale models allowing a comparison between calculated and observed distributions of lightning. The authors are encouraged to revisit their issues, as analyses of this kind are scarce and could help in better understanding the global circuit mechanisms.