1 Introduction
Fluid regimes attending granulite formation in the Earth's middle and lower crust provide important clues to lower crustal and crust-mantle interaction processes [12,23,24]. Granulite facies rocks that are characterized by dry mineral assemblages often contain CO2-rich fluids trapped as fluid inclusions in various minerals [13,14,18,23,25]. Recently syn-metamorphic carbonic fluids were also recorded from ultrahigh-temperature granulites formed by extreme crustal metamorphism at temperatures exceeding 1100 °C [26]. Precambrian high-grade terrains often associate late granite intrusions, and it is has been proposed that, in most cases, such granitoids emplaced into the upper continental crust were derived by partial melting of lower crustal granulites [3,4]. While the magma tectonics and geochemical features of these granitoids are well studied, information on their fluid characteristics is sparse. In this paper, we report results from a systematic fluid inclusion study of high to ultrahigh-temperature granite plutons that intrude Precambrian granulite facies rocks in southern India. These plutons belong to the Late Pan-African felsic magmatic suite recognized from several parts of the East Gondwana crustal fragments [11,16].
2 Geological framework
The three granite plutons investigated in this study are the Vattamali (VT) within the Palghat–Cauvery Shear Zone system in the north, the Gangaikondan (GK) at the southern part of the Madurai Block, and the Pathanapuram (PT) within the central part of the Achankovil Shear Zone (Fig. 1) from the Proterozoic granulite facies terrain of southern India. The GPS coordinates for the samples locations of VT, GK and PT plutons are

Geological framework of southern India (inset) showing major granulite blocks and shear zones. (a) Geology surrounding Vattamalai granite. (b) Geological map of the southern part of South India showing the locations of Gangaikondan and Pathanapuram granites (geological maps modified after [5]).
Cadre géologique du Sud de l'Inde (insert) indiquant les principaux blocs granulitiques et zones de cisaillement. (a) Géologie autour du granite de Vattamalai. (b) Carte géologique de l'extrémité méridionale du Sud de l'Inde, indiquant l'emplacement des granites de Gangaikondan et Pathanapuram (carte géologique modifiée d'après [5]).
The study area around VT granite falls within the central part of the Palghat–Cauvery Shear Zone system (PCSZ) (Fig. 1), a zone that marks the Archean-Proterozoic terrane boundary in southern India [6,20]. The dominant lithological units in the region are charnockites, ultrahigh-temperature granulites, hornblende gneisses, quartzofeldspathic gneisses, and intrusive granites. The granite shows coarse, massive texture without any visible foliation. Pink feldspar is the dominant constituent with subordinate plagioclase and quartz and accessory biotite. The pluton shows broad ovoidal shape and concordance of the contacts with the regional fabric of the host orthogneisses. The GK granite pluton occurs at the southern margin of the Madurai Block (cf. Fig. 1) and intrudes granulite facies gneisses. The highlands in this block are occupied dominantly by massive enderbitic granulites and charnockites, while the lowlands are intercalated with metasedimentary and meta-igneous rocks. The granite is medium grained and dominantly comprises pink K-feldspar, subordinate plagioclase and quartz with biotite as the major accessory. Few thin pegmatites and quartz veins several centimeters thick traverse the granite body. Development of a weak foliation and stretching of quartz in the granite indicate post-emplacement deformation. The PT granite occurs in the central domain of the Achankovil Shear Zone (ACSZ) (cf. Fig. 1) and is elongated parallel to the trend of the shear zone as well as the foliation of the host charnockites that carry cordierite and orthopyroxene. The granite is massive and shows no shearing or deformational features indicating that its emplacement post-dated shear deformation along the ACZ. The rock is medium to coarse grained with pink alkali feldspar and subordinate plagioclase and quartz. The major mafic mineral is hornblende. Biotite occurs as accessory. Minor quartz veins and pegmatites traverse the rock.
UPb electron microprobe dating of monazite grains from the VT granite yielded ages of 500–520 Ma [21]. Monazites from the PT granite have 520–580 Ma cores mantled by rims as well as bright overgrowths ranging in age between 500–540 Ma. Zircons from the GK granite belong to two distinct populations defining ages of
3 Analytical techniques
Petrographic studies were carried out on polished thin sections and modal analyses of the granites were performed using a point counter. Electron microprobe analyses of the mesoperthites were done using a JEOL JXA-8600M instrument housed at the Kochi University. An acceleration voltage of 15 kV, probe current of
4 Petrography and feldspar thermometry
The modal analyses data from point counting when recalculated and plotted in Q–A–P diagram classify all the three plutons as granite. The K-feldspar in all the three plutons shows mesoperthitic texture with coarse exsolution lamellae of Na-feldspar within host K-feldspar. We analysed representative mesoperthites from the VT and PT plutons using an electron microprobe. The re-integrated chemical composition of the mesoperthites was obtained using the modal ratio of host lamella and their respective chemical compositions following the techniques developed in recent studies [7,28]. The integrated compositions of the mesoperthites in the VT granite lie around 800–900 °C solvus for 5 kbar in An–Ab–Or diagram. Those of the PT granite cluster along the 900–1000 °C ternary feldspar solvus curves. These high temperature estimates compare with identical values of 900–1000 °C from mesoperthites in the host granulite facies rocks in the region [19] indicating the ultrahigh-temperature history for the Late Pan-African thermal event in southern India. The data also compare with temperature estimates from sapphirine and spinel bearing ultrahigh-temperature metamorphic rocks in this region [10].
5 Fluid inclusion studies
Fluid inclusions are commonly present in quartz and subordinately in feldspar in all the three plutons. They are ubiquitous in quartz grains within the granite matrix (Fig. 2) and range in size from less than 5 μm up to 30 μm. Majority of the fluid inclusions in all the three plutons occur scattered and azonal, or as isolated groups within various domains in the quartz grain, termed here as group 1. A second (group 2) occurs as arrays that pinch out within the grains. Subsequent late-generation inclusions (group 3) that transect grain boundaries and aligned along healed cracks are present in VT granite.

Photomicrographs of carbonic fluid inclusions in quartz from the Vattamalai (VT), Gangaikondan (GK) and Pathanapuram (P–T) granites. A, C and E show primary monophase CO2 inclusions. B, D and F show the distribution pattern of pseudosecondary/secondary inclusions as trails.
Microphotographies d'inclusions fluides carboniques dans le quartz des granites de Vattamalai (VT), Gangaikondan (GK) et Pathanapuram (PT). A, C et E : inclusions à CO2 primaire, monophasées. B, D et F : distribution des inclusions secondaires/pseudosecondaires le long de microfissures recristallisées.
The most common phase category among all the three groups is represented by monophase inclusions that show a single fluid phase at room temperature, and are notably abundant in the studied rocks, in contrast to normal granites which often lack CO2 inclusions. Results of microthermometric measurements of all the categories of inclusions from the three granite plutons are summarized in Table 1, along with computations of molar volumes and density values in terms of CO2 densities. Histograms compiling homogenization temperatures are shown in Fig. 3. The melting temperature data indicate that majority of the monophase inclusions in all the three plutons contains pure CO2 fluid. Only those from the PT pluton show slight depression in melting temperatures. Laser Raman microscope (LRM) analyses of representative inclusions from the granite plutons showed distinct Raman shift at 1283 and 1388 cm−1 peak positions corresponding to CO2. No peaks for CH4 or N2 were detected in the primary carbonic inclusions. However, those inclusions with the highest melting point depression from the PT pluton showed a minor Raman shift at 2331 cm−1, indicating traces of N2. An approximate estimation of the concentration of additional volatile phase that causes melting point depression in carbonic inclusions can be made by combining the melting and homogenization data and employing the thermodynamic data for CO2N2 mixtures [22]. From the minor peak for N2 in laser Raman data, we estimate a maximum of less than 5 mol% N2 from the curve intersection method using combined melting and homogenization data for the corresponding inclusions showing maximum melting point depression. It is therefore evident that the dominant fluids in the granitic plutons of present study are pure CO2.
Fluid inclusion microthermometric data
Données microthermométriques des inclusions fluides
Granite | Inclusion group | Host mineral | Melting temperature | Homogenization temperature | Molar Volume (cm3 mol−1) | Density (g cm−3) | Type of inclusion | ||||
Min. | Max. | Peak | Min. | Max. | Peak | ||||||
Vattamalai | group 1 | quartz | −57.2 | −56.6 | −56.9 | 2.0 | 5.0 | 2.5 | 48.25–50.22 | 0.912–0.876 | primary |
group 2 | quartz | −57.8 | −56.6 | −56.9 | 6.2 | 12.4 | 10.0 | 50.26–52.88 | 0.876–0.832 | pseudosecondary | |
group 3 | quartz | −58.3 | −56.6 | −56.9 | 13.6 | 21.2 | 17.0 | 53.42–56.90 | 0.824–0.773 | secondary | |
Gangaikondan | group 1 | quartz | −57.8 | −56.8 | −56.9 | 15.8 | 18.1 | 16.0 | 54.05–55.93 | 0.814–0.787 | primary |
group 2 | quartz | −57.0 | −56.6 | −56.6 | 18.6 | 23.6 | 19.5 | 56.15–60.24 | 0.784–0.731 | pseudosecondary | |
Pathanapuram | group 1 | quartz | −58.7 | −56.8 | −57.2 | 12.2 | 17.9 | 17.0 | 52.13–55.37 | 0.844–0.795 | primary |
group 2 | quartz | −58.3 | −56.9 | −57.2 | 18.1 | 29.9 | 29.0 | 55.51–73.59 | 0.793–0.598 | pseudosecondary |
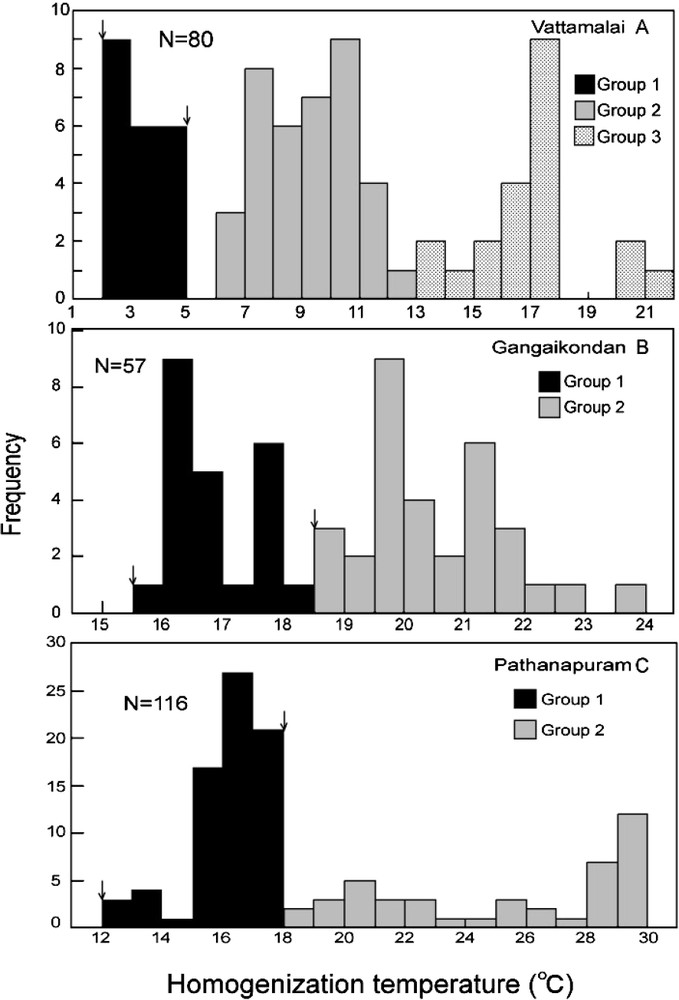
Histograms showing homogenization temperatures of carbonic inclusions in VT, GK and P–T plutons. The arrows denote the ranges of values used to compute the isochores shown in Fig. 4.
Histogrammes des températures d'homogénéisation des inclusions carboniques dans les plutons VT, GK et PT. Les flèches indiquent les valeurs utilisées pour calculer les isochores indiqués sur la Fig. 4.
The lowest homogenization temperatures (2.0 °C) were yielded by group-1 inclusions in the VT pluton. The highest homogenization temperature (29.9 °C) is recorded by group-2 inclusions in PT granite. The peak homogenization temperatures for group-1 inclusions in GK (16.0 °C) and PT (17 °C) are similar, but for those in the VT pluton, the temperatures are distinctly lower (2.5 °C). The homogenization temperature of group-1 inclusions in GK and PT are similar to the late inclusions (group 3) in VT (peak at 17 °C). Clearly, the VT pluton preserves the highest density CO2 fluids among all the three locations with a density up to 0.912 g cm−3 and molar volume of 48.25 cm3 mol−1. Also, the homogenization data show that there is systematic decrease in the density values from group-1 through group-2 inclusions in all the plutons. In VT, a late category of group-3 inclusions is also identified, showing the lowest density among the three groups of inclusions. Thus, although the absolute densities are different in the different plutons, in general, the primary inclusions in all cases preserve the highest density fluid.
Knowledge on the composition and density of a fluid constrain it to lie along an isochore in the P–T space. In the present study, the density data from CO2 inclusions have been employed in constructing representative isochores. Representative isochores of fluid inclusions in the three plutons are shown in the P–T space in Fig. 4.
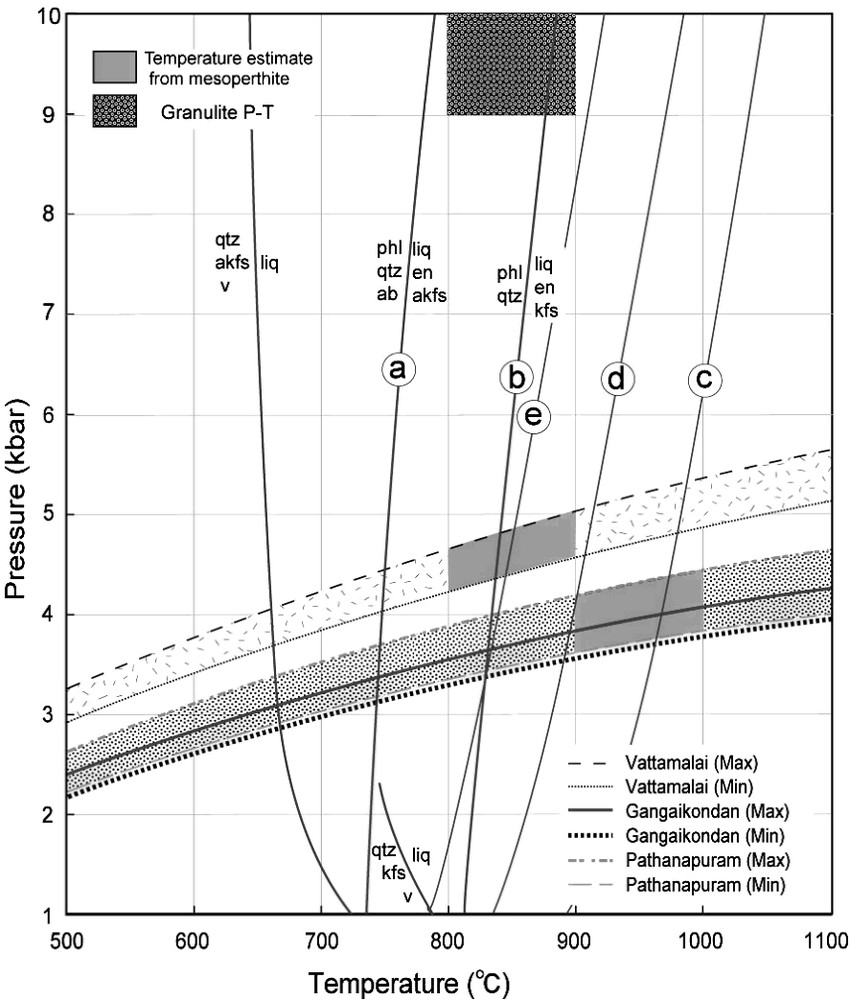
Combined P–T diagram showing CO2 isochores for primary inclusions in the granite plutons and temperature data from feldspar thermometry in mesoperthites. Also shown are phlogopite dehydration melting curves (marked as a and b, after Johannes and Holtz, 1966, see [2]) and liquidus for water undersaturated granitic systems with 1, 2 and 3% H2O (curves c, d and e, respectively, from Johannes and Holtz, 1966, see [2]). P–T box for granulitic lower crust is also shown. See text for discussion. Masquer
Combined P–T diagram showing CO2 isochores for primary inclusions in the granite plutons and temperature data from feldspar thermometry in mesoperthites. Also shown are phlogopite dehydration melting curves (marked as a and b, after Johannes and Holtz, 1966, ... Lire la suite
Diagramme P–T synthétique, indiquant les isochores d'inclusions primaires dans les plutons granitiques et les températures déduites par thermométrie sur feldspaths dans les mésoperthites. On a aussi indiqué les courbes de fusion par déshydratation du phlogopite (a et b, d'après Johannes et Holtz, 1966, voir [2]), ainsi que les liquidus pour les systèmes granitiques sous-saturés en eau à 1, 2 et 3% H2O (courbes c, d et e, respectivement, d'après Johannes et Holtz, 1966, voir [2]). Les conditions P–T de la croûte inférieure granulitique sont également indiquées. Voir le texte pour la discussion. Masquer
Diagramme P–T synthétique, indiquant les isochores d'inclusions primaires dans les plutons granitiques et les températures déduites par thermométrie sur feldspaths dans les mésoperthites. On a aussi indiqué les courbes de fusion par déshydratation du phlogopite (a et b, ... Lire la suite
6 Discussion
The temperature estimates from feldspar thermometry in the granites (800–1000 °C) are similar to the recent estimations of high and ultrahigh-temperatures (900–1000 °C) for granulite facies metamorphism in the region (e.g., [8,10]). The isochores of fluid inclusions computed from density data can be used in conjunction with the feldspar thermometry data to derive broad position of the P–T box. We show the combined plots in Fig. 4. Assuming that the granites were generated from granulitic lower crust (see below) at ca. 800–900 °C and 10 kbar, the possible model for dehydration melting in this domain is given by phlogopite dehydration curves of Johannes and Holz, 1996 (quoted in Barbie and Libourel [2]) and can be bracketed by the following reactions:
At low T:
(a) |
(b) |
The granulite P–T box shown in Fig. 4 is clearly bound by the above two reactions. The second reaction (reaction (b) also passes through the P–T window for the VT granite. We also compare in Fig. 4, the position of the P–T boxes with respect to the liquidus for water-undersaturated granitic system from the work of Johannes and Holz (1966, quoted in Barbie and Libourel [2]). Curves c, d and e in Fig. 4 represent 1, 2 and 3% H2O in the melt, respectively. Curve c penetrates the P–T box for GK and PT granites, while curve e passes through the P–T box for VT granite. These results are in good agreement with the P–T conditions of crystallization of the granite plutons. The consumption of the dissolved aqueous phase for the formation of biotite upon crystallization of the melt would explain the lack of common occurrence of aqueous inclusions. The relatively higher water content in the magma indicated for VT granite also accounts for the higher modal abundance of biotite in this granite. The host granulite facies rocks record a steep isothermal decompression event after the high to ultrahigh temperature metamorphism, correlated to a distentional tectonic regime (e.g., [8]). The emplacement of the felsic plutons are also perceived to have occurred during extensional collapse following suturing of the East and West Gondwana megacontinents [17], with a clockwise exhumation path (not shown in the figure). Thus, the P–T paths of the granitic plutons are characterized by decompression at high temperatures (adiabatic intrusion) during the magmatic stage, followed immediately by sub-isobaric cooling upon crystallization, and finally decompression during erosion and unroofing. The preservation of high density negative-crystal shaped inclusions indicates that the P–T path closely followed the slope of the isochore.
Carbonic fluid inclusions are known to occur in a wide variety of rock types formed under variable pressure-temperature conditions and under different tectonic settings. CO2-rich fluid inclusions have also been reported from mantle xenoliths [1]. CO2 forms the dominant fluid species in high-grade metamorphic rocks [24], including deep crustal and ultrahigh temperature granulites [18,26]. Touret [23] proposed a model of fluid stratification in the earth's crust with the upper regions dominated by H2O and brine solutions incorporating variable amounts of CH4 and/or N2. The deeper domains, on the other hand, are rich in CO2H2O fluids that merge into a dominantly CO2-rich lower crust. The observation of common occurrence of CO2-rich fluids in rocks derived from the deep crust and upper mantle, some of which show extremely high density [18] has validated this proposal.
The spectacular abundance of CO2-rich fluid inclusions in the three granite plutons of the present study closely correlate with the fluid inclusion characteristics of the host granulite facies rocks in the terrain [12–14,25], but is distinct from normal mid-crustal granites which do not contain CO2 in abundance. Following the techniques outlined by Touret and Hansteen [25], we estimate up to 1 wt% CO2 in our samples, which suggests that these magmas were substantially enriched in CO2. Recent studies show that partial melting reactions which generate granite magmas under upper amphibolite to granulite facies conditions were water deficient [4]. Since the granulite facies protoliths were already water deficient, it is unlikely that the CO2-charged granites reported in this study were generated through vapor-absent processes. Preliminary carbon isotope data from fluid inclusions in the Pan-African felsic magmatic rocks from this region show a range of
Available geochronological data indicate that the latest granulite facies metamorphism and felsic magmatism in this region are broadly coeval, related to the Late Pan-African tectonothermal event associated with the collision and subsequent extensional collapse accompanying the birth of the Gondwana supercontinent within which southern India was an integral part [20]. Southern India and Madagascar preserve crucial evidence for CO2 infiltration from mantle sources during supercontinent assembly.
Acknowledgments
Prof. J.L.R. Touret provided valuable comments and very helpful advice that aided in substantially improving an earlier version of this paper. Dr. Michel Cuney and an anonymous referee are thanked for helpful comments. We thank Kochi University for research facilities. This is a contribution to the project funded by Japan Ministry of Education, Science, Culture, Sports, Science and Technology (M. Arima, project leader).