Version française abrégée
Au cours des 10 dernières années, les propriétés magnétiques des séquences sédimentaires marines ont été de plus en plus utilisées comme traceurs des changements paléoenvironnementaux. Le paramètre magnétique le plus communément utilisé en paléocéanographie, grâce notamment au développement des instruments embarqués à bord des navires océanographiques et permettant de mesurer les propriétés physiques des sédiments en continu, est la susceptibilité magnétique en champ faible
Il est avéré que la χ peut être contrôlée, entre autres, par des changements dans la productivité biogène, les arrivées détritiques, la quantité de carbonate de calcium, les conditions d'oxydo-réduction. Tous ces facteurs influent sur la nature, la concentration et la taille des grains magnétiques, qui sont autant d'éléments essentiels à déterminer si l'on veut déchiffrer les changements environnementaux et notamment les changements de source, de transport, de mode d'archivage sédimentaire.
Contrastant avec l'abondance des enregistrements de χ, les analyses détaillées des propriétés physico-chimiques de la fraction magnétique sont peu nombreuses et se sont développées en Atlantique nord, à haute résolution, surtout depuis 10–15 ans. De nombreuses séquences sédimentaires provenant de différents bassins en Atlantique nord et couvrant la dernière période glaciaire ont été analysées au laboratoire de magnétisme environnemental du LSCE [18,19,30,36]. Les résultats, complétés par ceux obtenus par d'autres laboratoires en parallèle sur d'autres carottes [21,26,29,32] (Tableau 1) ont montré que l'on peut distinguer deux provinces (Fig. 1). La première correspond à la bande de Ruddiman répartie entre 40° et 55°N. Cette région a reçu, pendant la dernière période glaciaire, les débâcles d'icebergs venant des calottes de glaces environnantes et surtout de la calotte Laurentide (niveau de Heinrich) [3,11,12,14] avec, parfois, des précurseurs européens [13]. Les analyses détaillées ont montré que les pics de susceptibilité magnétique, associés aux événements de Heinrich dans ces carottes (Fig. 2), illustrent une augmentation nette de la quantité de grains magnétiques par rapport au sédiment hemipélagique « normal », c'est-à-dire hors niveaux de Heinrich. Une partie des grains magnétiques dans ces niveaux sont « libres », d'autres sont inclus dans des agrégats de roches arrachés au continent ou dans d'autres minéraux de type feldspaths. De plus, la relation entre l'aimantation rémanente anhystérétique (ARA) et la susceptibilité, utilisée comme un indicateur de la taille des grains magnétiques [16,17], montre que les grains magnétiques présents dans les niveaux de Heinrich sont grossiers, de type multidomaine (Fig. 3). Il s'agit donc de la caractérisation du dépôt, par advection verticale (fonte d'icebergs), de grains magnétiques grossiers, formant un sable magnétique au sein du sable lithogénique provenant du craton canadien, érodés par les glaces et transportés jusqu'aux sites par les icebergs. Ceci est observé selon un transect plus ou moins ouest-est, depuis la mer du Labrador [29,30] jusqu'à la marge du Portugal [21,32].
Location and water depths of the cores reported in Fig. 1. The references of the mineral-magnetic articles are given in the right column (those for which only χ was reported are not mentioned)
Localisation et profondeur d'eau pour chaque carotte reportée sur la Fig. 1. Les références aux articles faisant état d'analyse des propriétés magnétiques sont données dans la dernière colonne (ceux montrant uniquement les enregistrements de χ ne sont pas mentionnés)
Core | Latitude | Longitude | Water depth (m) | Reference |
Ruddiman latitudinal belt (40°N–55°N) | ||||
SU90-39 |
|
|
3955 | [36] |
SU90-08 |
|
|
3080 | [36] |
SU90-09 |
|
|
3375 | (this study) |
S8-79-4 |
|
|
3877 | [26] |
D9812 |
|
|
3898 | [26] |
P094 |
|
|
3448 | [29] |
MD95-2024 |
|
|
3448 | [30] |
MD95-2039 |
|
|
3381 | [32] |
MD95-2040 |
|
|
2665 | [21] |
MD95-2042 |
|
|
3146 | [21,32] |
Path of the North Atlantic Deep Water | ||||
MD95-2010 |
|
|
1048 | [18,19] |
MD95-2009 |
|
|
1027 | [18,19] |
SU90-24 |
|
|
2085 | [18,19] |
SU90-33 |
|
|
2370 | [18,19] |
SU90-16 |
|
|
2100 | [18,19] |
PS2644-5 |
|
|
777 | [18,19] |
MD95-2034 |
|
|
4461 | [19] |
SO82-05 |
|
|
1420 | [28] |
SO82-07 |
|
|
1580 | [28] |
LO09-18 |
|
|
1460 | [28] |

Map of North-Atlantic showing the location of the cores for which some detailed magnetic analyses have been reported (see Table 1 for references). The full dots are for the cores analyzed at LSCE and the open dots are for the cores analyzed in other laboratories. The dark grey thick line illustrates the path of the North Atlantic Deep Water and the light grey thick line illustrates the schematic limits of the Ruddiman belt.
Carte de l'Atlantique nord montrant la distribution des carottes ayant fait l'objet d'analyses détaillées de la fraction magnétique pour la dernière période glaciaire (voir Tableau 1 pour les références). Les points noirs correspondent aux carottes étudiées au LSCE, les points gris, sont les carottes étudiées dans d'autres laboratoires. La ligne épaisse en gris foncé schématise le parcours de l'eau profonde nord-Atlantique, et la ligne épaisse en gris clair les limites approximatives de la bande de Ruddiman.

Low-field susceptibility records (in 10−4 SI) reported versus depth (m) for a few representative cores and the last glacial period. The grey curves are typical for the Ruddiman Belt while the others show the short term variations in which Heinrich layers (vertical lines) coincide with minima. Same symbols as in Fig. 1.
Courbes caractéristiques de susceptibilité magnétique en champ faible, obtenues en Atlantique nord pour la dernière période glaciaire. Les courbes grises sont typiques de la bande de Ruddiman, alors que les autres courbes montrent les variations de courte période avec les niveaux de Heinrich coïncidant avec des minima magnétiques. Mêmes symboles que dans la Fig. 1.
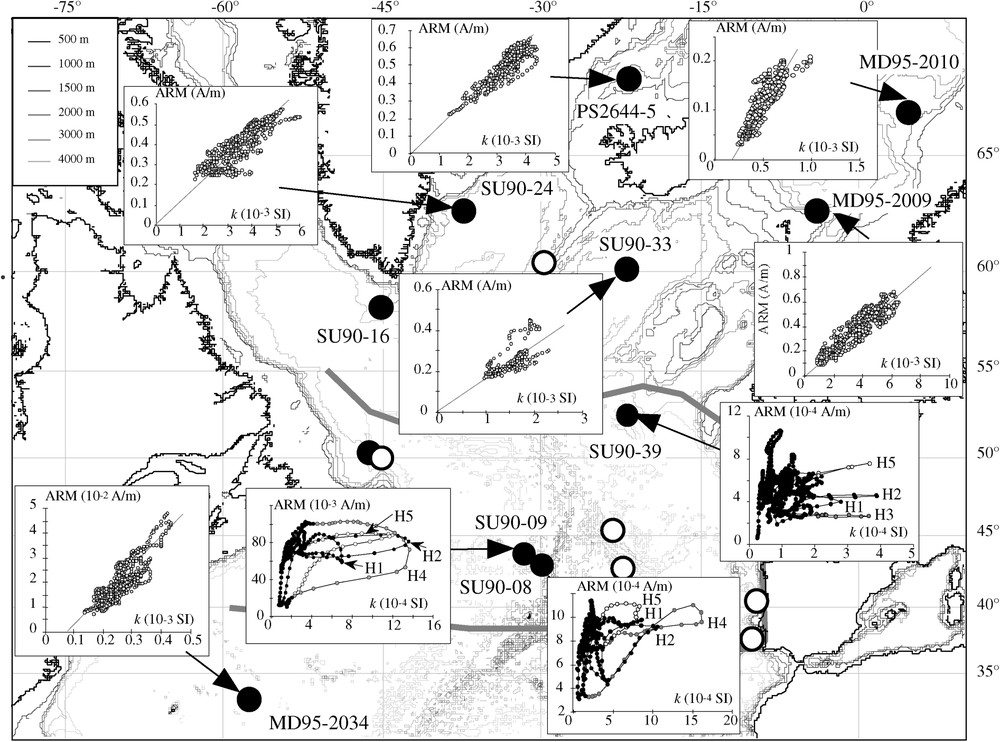
Representative ARM versus diagrams used to retrieve changes in magnetic grain size. The Heinrich layers are clearly characterized in the Ruddiman belt by loops toward coarser grains while in the other cores, the magnetic grain size is rather uniform. Same symbols as in Fig. 1.
Diagrammes d'ARM en fonction de χ, utilisés pour déterminer les changements de taille de grains magnétiques. Les niveaux de Heinrich sont clairement caractérisés dans la bande de Ruddiman par des boucles indiquant des grains plus grossiers, alors que dans les autres carottes, la taille des grains magnétiques est uniforme. Mêmes symboles que dans la Fig. 1.
La deuxième province est caractérisée par un signal de susceptibilité magnétique radicalement différent, mentionné pour la première fois par Rasmussen et al. [24] dans le chenal Faeroe–Shetland et que l'on retrouve systématiquement aux sites distribués le long du parcours de l'eau profonde nord-Atlantique depuis la mer de Norvège jusqu'aux Bermudes [19] (Fig. 2). La taille des particules magnétiques dans ces carottes est relativement uniforme (Fig. 3) et les variations observées résultent donc essentiellement de variations dans la concentration magnétique. Les événements de Heinrich coïncident, cette fois-ci, avec des minima de concentration et sont séparés par des variations à plus courte période. Il a été montré que toutes ces variations rapides dans la concentration magnétique de ces carottes sont en phase avec les changements de température de l'air au-dessus du Groenland [5]. Les magnétites contenues dans ces carottes provenant principalement de la zone basaltique Islande–Faeroe, leurs variations en concentration sont interprétées comme étant liées à un transport plus ou moins actif par les courants de fonds, depuis la source basaltique au nord vers les basses latitudes. Ainsi, pendant les périodes chaudes (froides), plus (moins) de matériel magnétique est transporté par cette masse d'eau profonde. Ceci est le témoin d'une convection et donc formation d'eau profonde plus active pendant les périodes chaudes que pendant les périodes froides.
Ainsi, l'océan profond peut avoir une dynamique très rapide, beaucoup plus rapide que ce qui était observé jusque-là à l'échelle orbitale et des changements très rapides peuvent affecter la circulation thermohaline dans son ensemble, ce qui a bien sûr des répercussions importantes sur l'ensemble du climat.
Ces analyses détaillées des propriétés magnétiques des sédiments nord-Atlantique, couplées à d'autres analyses de la fraction détritique et biogénique du sédiment, ont montré qu'il s'agit d'un outil très performant pour déchiffrer les variations environnementales passées.
1 Introduction
Over the past 20 years, the use of the magnetic properties of sediments as tracers for past environmental/climatic changes has played an increasing role. In particular, the low field magnetic susceptibility easily measured continuously on cores or on sub-sampled sections of cores has been used for inter-correlations of sedimentary sequences from the same area.
Downcore variations in the susceptibility
A completely different susceptibility pattern was observed in a core located at the northeast Faeroe Island margin [24]. In this core, the χ record exhibits much shorter time variations than in the Ruddiman belt. The authors observed that these short term changes mirror the
These different studies demonstrated that χ is capable of recording short climatic events and that in the subpolar North Atlantic the χ signal shows a different general pattern than that observed in the Ruddiman belt. It is well established that changes in biogenic productivity, in terrigeneous input, in carbonate content and/or in Red-Ox conditions control the behavior of magnetic susceptibility records. These factors impact on the mineralogy, the concentration and the granulometry of magnetic grains which are, therefore, essential parameters to the understanding of the meaning of the observed variations in the low field susceptibility. Many records of the low field susceptibility have been obtained in North Atlantic but only a few of them are accompanied by a full characterization of the magnetic fraction.
We present here a review of the results obtained on the basis of mineral-magnetic studies conducted at LSCE over the last 10 years on cores from the different basins of North Atlantic and covering the last glacial period. These results are compared with and completed by those obtained in parallel by other authors, mainly in the area concerned by the iceberg discharges.
2 Core descriptions and methods
All the cores studied at the LSCE have been collected during French and/or international cruises on the R.V. Suroit during the Paleocinat cruises in 1990 and 1992 (SU90- and SU92-) and on the R.V. Marion Dufresne during the IMAGES cruise (MD101: MD95-). A core collected by the German R.V. Polar Stern (PS-2644-5) has also been analyzed. The location of the cores is reported in Fig. 1 and in Table 1. Three of them are located in the central part of the Ruddiman belt (SU90-08 and SU90-39 [36]; SU90-09 has been studied since then and results have not been published, except for the susceptibility record [13]), one has been taken at the exit of the Labrador Sea [30], six of them are located north of 55°N and one south of 40°N [18,19]. In the Ruddiman belt, other cores have also been studied with various details from a magnetic point of view. The first one is located on the western side just at the exit of the Labrador sea (core P094; [29]) and it was the pilot core for core MD95-2024 then analyzed at LSCE [30]. Two other cores, north of the Azores (S8-79-4 and D9812) have been studied by Robinson et al. [26] and finally the third group, off Portugal (cores MD95-2039; -2040 and -2042) was studied by Thouveny et al. [32] and Moreno et al. [21]. Magnetic grain size determinations were also conducted on three cores located on the Reykjanes Ridge at about 57°N [27].
At LSCE, the cores have been sampled continuously with u-channels [31,35]. The remanent magnetizations were measured using a 755-R 2G cryogenic magnetometer with the high resolution set of SQUID (4 to 5 cm) and the low field susceptibility with a small diameter Bartington coil. The magnetic parameters (susceptibility, anhysteretic remanent magnetization (ARM), isothermal remanent magnetization (IRM), S-ratio) have been measured using the methods described in [35,36] for cores SU90-08 and SU90-39 and in [19] for the other cores. The other studies [21,26,29] were made on discrete cubic samples. Core MD95-2039, also sampled with u-channel was measured with a 760R-2G magnetometer [32].
3 Results
The detailed analyses of the magnetic properties mainly consist in identifying the magnetic minerals, the variations in their grain size and in their concentration. Not all the same parameters are measured by the different authors. While the details of the analyses can be found in each article, in order to compare the results, we focus here on the combination between the low field magnetic susceptibility and the ARM, as they are the parameters commonly measured in all the cores. ARM is mostly carried by fine grained ferromagnetic particles and when this parameter is reported versus χ, it gives access to the changes in the magnetic grain size [16,17]. On these ARM versus χ diagrams, uniformity of magnetic grain size is illustrated by the points plotted falling along a line passing through the origin. Any departure from this line toward steeper (shallower) slopes corresponds to a decrease (increase) of the average magnetic grain size. These observations were coupled with the magnetic hysteresis parameters measured every 5 or 10 cm and combined as suggested by Day et al. [6] in [19,29]. Two main magnetic patterns can be distinguished as described above and the cores can be divided into two groups: the one located within the Ruddiman belt and those along the path of the North Atlantic Deep Water.
In all cases, magnetite is recognized as the dominant magnetic mineral in the studied sediments. This is documented by thermomagnetic curves showing a single Curie point around 580 °C [18,19,36], by the S-ratio close to unity [18,19,29,30], by low temperature measurements showing the Verwey transition [29] or by direct observation of the magnetic grains with scanning electron microscope [32]. Off Portugal, variable amounts of hematite are also detected in the cores and attributed to climatically induced eolian arrivals of dust from the continent [21,32].
3.1 Ruddiman latitudinal belt (40°–55°N)
The susceptibility pattern reported for the first time by Robinson et al. [25] is confirmed in every core located in the IRD belt with marked peaks in the susceptibility records coinciding with the massive iceberg discharges, the so-called Heinrich layers [14] (this has also been incremented by 17 additional susceptibility records reported by Robinson et al. [26]). Representative records of cores SU90-08, SU90-39 [36] and SU90-09 [13] are reported in Fig. 2. The peaks may reach 12 to 20
All the authors notice that the correction for carbonate content does not modify the main pattern of the records confirming the observation first made on longer time scale by Poutiers and Gonthier [23]. However, these features are not visible in the records of ARM often expressed as the anhysteretic susceptibility (
The analysis of the magnetic properties of these cores thus shows that the Heinrich layers are characterized by a significant increase in both the relative concentration and the grain size of magnetic particles. This is the expression of abrupt delivery by the icebergs of magnetic ‘sand’ mixed with the main lithogenic sand. In the studied cores, the background magnetic level is so weak compared to the Heinrich peaks that any other source than ice rafted magnetic particles seems to be negligible.
Robinson et al. [26] have published a map showing the χ values of North-Atlantic deep-sea sediment for the Last Glacial Maximum (LGM) relative to the core tops. On this map, high values of this susceptibility ratio, attributed by the authors to the occurrence of melting icebergs during the LGM, are distributed along the East Greenland coast, in the latitudinal Ruddiman belt and on the Rockall plateau. Low values are found in the Norwegian Sea and on the eastern side of the Mid-Atlantic Ridge. This distribution led the authors to define the general trends of the surface currents driving the icebergs trajectories during the LGM. However, this is valid only for the LGM and assuming that no differential dilution by non-magnetic fraction between the LGM and the core tops is modulating the magnetic record at any site. In other words, the authors assume that a single source (ice-rafting) is at the origin of the observed distribution.
3.2 Path of the North Atlantic Deep Water
Core ENAM 93-21, in which Rasmussen et al. [24] recognized a low field susceptibility record very different from that observed within the main IRD belt and mimicking the
Similar susceptibility patterns are recorded in these cores during the last glacial period [18,19] (Fig. 2). They are characterized by large amplitude, short-term variations which coincide with changes in the laboratory induced ARM (and also IRM). This similarity between the three bulk magnetic parameters indicates that the susceptibility variations do not result from changes in magnetic grain sizes. In fact, as illustrated in Fig. 3, when ARM is reported versus χ, the points are rather well grouped along a line and do not show any significant excursions toward coarser grains as in the IRD belt. The magnetites have a uniform average grain size of a few micrometers as indicated by the hysteresis parameters falling into the pseudosingle domain area of the Day diagrams [19]. In addition, the magnetites in these cores are easy to extract indicating that they are not included into other minerals or into rock aggregates. Therefore, the variations observed in the susceptibility records along the path of the North Atlantic Deep Water illustrate only variations in the relative amount of relatively well sorted magnetite grains within the detrital fraction. The origin and the pathways of these magnetites are therefore different from those invoked for the Ruddiman belt.
When they are available on the same cores, depleted values of planktic
Because they look similar, susceptibility records from the Faeroe Shetland Channel [24] and from the Reykjanes ridge [22] were directly correlated by these authors to the
The average level of all the bulk magnetic parameters measured in these cores is very high, indicating that these sediments are very rich in magnetites most likely originating from the basaltic province of Iceland and Faeroe islands [18,19,22–24]. Considering the geographical distribution of the sites, and because no significant contribution of coarse magnetic IRD is observed in these records, Kissel et al. [19] concluded that transport of the magnetic particles by deep sea currents appears as the only realistic mechanism. Oscillations of the bulk magnetic parameters may either be due to variations in the efficiency of these bottom currents or to varying dilution. Dilution by carbonates is very unlikely as carbonate content does not vary so much in these high latitude cores. Dilution by non-magnetic IRD input during cold periods has also been at least partly rejected [19] because not all the magnetic minima coincide with IRD events (see, for example, core MD95-2034 from the Bermuda Rise). The authors concluded that dilution may play a role in modulating the magnetic records but cannot account for the entire observed variability. The bottom currents associated to the NADW thus transport high (small) amounts of pseudosingle domain magnetite grains during warm (cold) events along the path of the overflow waters. This indicates a more active or reduced convection and formation of deep waters during interstadials and Heinrich and stadials respectively. This is consistent with the benthic
Off Portugal, changes in the ARM/χ ratio have been observed also in phase with the Greenland ice
It has been recently suggested that rather than the concentration in magnetic grains which might be subjected to dilution effects, the very slight changes in the magnetic grain size around the average value are better tracers of variations in the bottom current activity [28]. In cores from the Reykjanes ridge, the authors observe a saw-tooth pattern in the magnetic grain sizes with the coarsest grains deposited at the end of the interstadials and the finest during each cold event. In the core located at the southern tip of Greenland, Kissel et al. [19] also mentioned slightly coarser grains during interstadials but with a symmetrical pattern. So far, these observations are only made locally and their regional significance is not yet proved. In order to better constrain the variations in the magnetic content and grain size along the NADW path and their relationship with the other detrital components of the sediment, a multi-proxy approach at very high resolution on these cores is now underway at LSCE (Ballini et al., in prep.)
4 Conclusion
Beyond the simple measurement of the low field magnetic susceptibility which allows inter-correlations of cores to be made, complete rock magnetic properties analyzed on cores taken from different parts of the North Atlantic ocean have proven the ability of the magnetic analyses to detect and trace rapid marine environmental changes. Depending on the location of the cores, different modes of re-distribution of the detrital fraction of the sediment among which the magnetic grains can be distinguished. In the IRD belt between 40° and 55°N, abrupt vertical deliveries of magnetic sandy material from icebergs are associated with changes in the surface conditions. By contrast, along the path of the North Atlantic Deep Water, the magnetic content of the cores is rather uniform in grain size and the changes in the magnetic concentration are controlled by changes in the dynamic of the bottom current. The latter are related to atmospheric changes over Greenland with enhanced (respectively reduced) strength of the bottom currents transporting more (respectively less) magnetic material to the sites during relatively warm (respectively cold) periods.
The use of the magnetic properties of marine sediments is thus a powerful tool for reconstructing past environmental changes. The geographical distribution in North Atlantic of the cores in which the rock magnetic properties have been analyzed in details is rather large and allows one to describe the dynamic of the bottom current during the last glacial period. Further effort should be carried out now to compare these records with other proxies for bottom current and sediment provenance such as clay minerals, microgranulometry, benthic carbon isotopes,... If such analyses are conducted on the same cores, they would allow us to discriminate the local effects and the regional ones carried by each proxy.
Acknowledgements
The research scientists, students, post-doc fellows, and technicians of the Environmental Magnetism team of LSCE are fully acknowledged for the work reported in this paper. In particular, these studies were motivated by C. Laj, who is also at the origin of the development of our laboratory around the high-resolution continuous magnetic measurements of sedimentary sequences.