Version française abrégée
1 Introduction
La composition chimique des biotites est classiquement utilisée pour l'estimation des conditions de genèse des roches magmatiques porteuses : fugacité d'oxygène des magmas parents [13,16], température du liquidus [1], classification typologique des granitoïdes [2,12] et la datation des événements thermiques subis par ces roches.
Cela implique que les biotites utilisées soient primaires et que leurs compositions reflètent bien des conditions magmatiques. Or, on constate que, dans un même massif, pour un faciès déterminé et pour des biotites considérées comme primaires selon les classiques critères pétrographiques, les compositions de ces minéraux peuvent varier considérablement. Ces variations sont attribuables à des altérations tardi- à post-magmatiques induites par des circulations hydrothermales et limiteraient donc, de fait, les possibilités d'emploi de la chimie des biotites pour les buts exposés plus haut.
Le but du travail présenté ici est de proposer un moyen de discriminer les biotites primaires magmatiques et celles qui ont subi un rééquilibrage chimique plus ou moins complet avec un fluide hydrothermal tardi-magmatique, et celles qui ont été néoformées à l'équilibre avec un fluide tardif.
Le moyen proposé est l'utilisation d'un diagramme triangulaire reposant sur les teneurs en certains constituants des biotites (TiO2, FeO, MnO et MgO), facilement dosables, avec une bonne précision, à la microsonde électronique.
2 Établissement du diagramme TiO2–FeO*–MgO
2.1 Choix des constituants
Parmi les constituants majeurs des biotites, nous avons retenu comme pôles du diagramme triangulaire les oxydes suivants : FeO* (FeO* = FeO + MnO), MgO et TiO2.
Parallèlement à la base FeO*–MgO du diagramme, seront distinguées les biotites issues de lignées magmatiques acides (vers le pôle FeO*) ou basiques (vers le pôle MgO). Selon la valeur de FeO*/(FeO* + MgO), la teneur en TiO2 sera fonction de la température de formation [3,15] ou de transformation des biotites.
De fait, le report d'environ 480 compositions chimiques de biotites d'origines variées, extraites de la littérature et/ou issues de ce travail (Fig. 1), montre bien la répartition des points dans l'ensemble du diagramme.
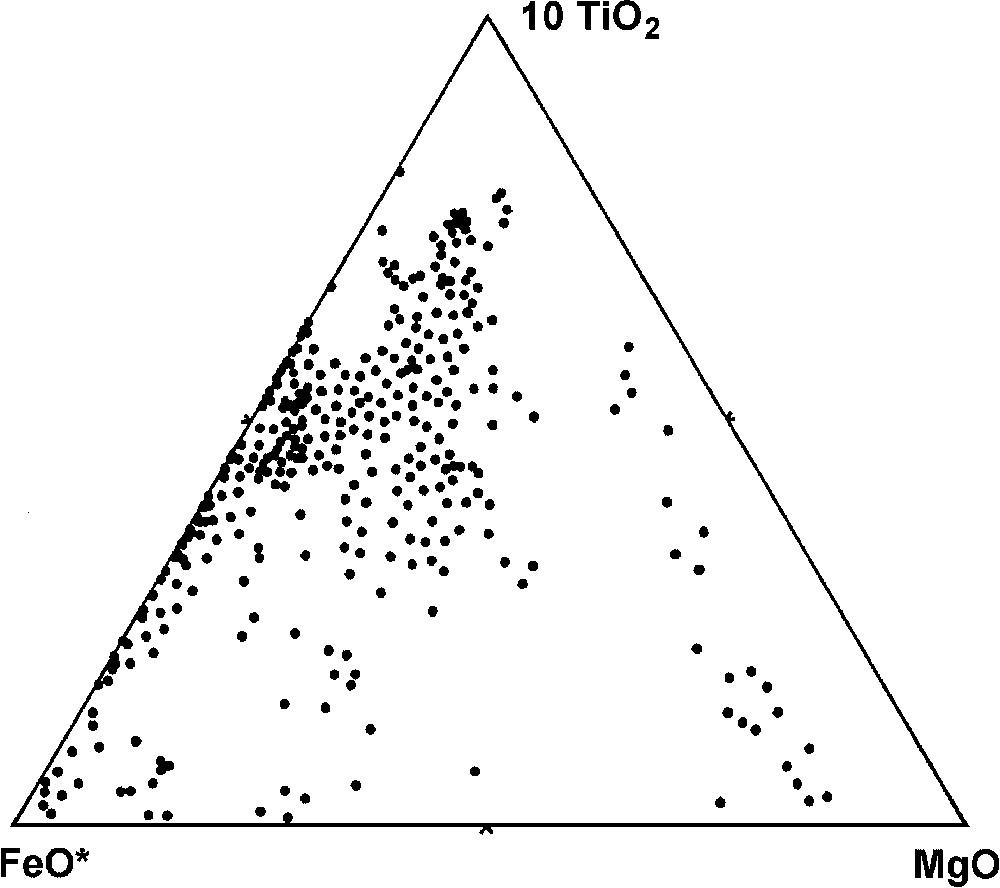
Chemical composition of biotites in the 10 TiO2–FeO*–MgO ternary diagram.
Composition chimique des biotites dans le diagramme 10 TiO2–FeO*–MgO.
2.2 Délimitation des domaines des biotites magmatiques, rééquilibrées et néoformées
2.2.1 Domaine des biotites magmatiques
La limite entre le domaine d'existence des biotites magmatiques (domaine A, Fig. 2) de celui des biotites transformées ou néoformées a été fixée de la façon suivante :
- – pour des valeurs de FeO*/(FeO* + MgO) allant de 0,70 à 0,85, nous nous sommes fondés sur les données de Monier [9] relatives à la coexistence de biotites avec des muscovites très précoces, de haute température (>650 °C) et donc considérées comme ayant cristallisé à l'extrême fin de la solidification d'un magma granitique ;
- – pour des valeurs plus basses de (), nous avons sélectionné des biotites de quelques séries particulières [10,11], qui présentent des caractères morphoscopiques et optiques semblables à ceux des biotites précédentes : lamelles automorphes, forte coloration brune, fort pléochroïsme, absence d'oxydes titanés exprimés et teintes de polarisation masquées par la couleur propre du minéral ;
- – pour des biotites dont le est inférieur à 0,5, nous avons choisi de prolonger la limite du domaine A vers le pôle titanifère du diagramme, incluant les biotites les plus titanifères des roches ultrabasiques et les plus titanifères de celles des roches basiques [5,14]. Au-delà, la limite du domaine est plus incertaine et ne représente qu'une extrapolation de la courbe limite vers le pôle TiO2 du diagramme.

Limits of the domain for magmatic biotites. (1) Early high-temperature muscovite (>650 °C) in equilibrium with magmatic biotites (2), from granites of Limousin (Massif Central, France). (3) Biotites from Commana cordierite granite, (4) biotites from Rostronen cordierite granite and (5) biotites from Plouaret diorite (Armorican Massif, France). (6) Biotites of high-temperature metasomatose (>1000 °C) of forsterite peridotites (Batan Island, Philippines). (7) Biotites from mafic rocks (gabbros from the Kerguelen Islands). (8) Biotites from alkaline granites of Corsica. (9) Biotites from alkaline granites of central Nigeria (a: unmineralized facies; b: mineralised facies). (10) Biotites from much differentiated granites (aplites, alkaline granites) (Nigeria).
Délimitation du domaine des biotites magmatiques. (1) Muscovites précoces de haute température (>650 °C) en équilibre avec les biotites magmatiques (2), dans les granites du Limousin (Massif central, France). (3) Biotites du granite à cordiérite de Commana (Massif armoricain, France), (4) biotites du granite à cordiérite de Rostrenen et (5) biotites de la diorite de Plouaret (Massif armoricain, France). (6) Biotites de métasomatose de haute température (>1000 °C) dans des péridotites à forstérite (île de Batan, Philippines). (7) Biotites de roches basiques (gabbros) des Kerguelen. (8) Biotites de granites alcalins de Corse. (9) Biotites de granites alcalins du Nigeria central (a : faciès non minéralisés, b : faciès minéralisés). (10) Biotites de granites très différenciés, aplites, granites alcalins (Nigéria).
En ce qui concerne les valeurs de supérieures à 0,85, elles correspondent, soit à des biotites de leucogranites très évolués de séries calco-alcalines ou alumino-potassiques, soit à des biotites de granites de séries alcalines. Pour des valeurs de supérieures à 0,96, la limite du domaine A est précisée par les compositions des biotites primaires de granites alcalins de Corse [4] et du Nigeria [6].
Les biotites dont les compositions se situent dans le domaine A présentent toutes des caractères physicochimiques communs, compatibles avec leur équilibre avec un liquide magmatique : teneurs élevées en titane proches des maxima de solubilité dans les roches plutoniques [15], et teneurs faibles ou nulles en aluminium octaédrique [10]. Les valeurs en [6]Al des biotites des granites alumino-potassiques ne dépassent pas 1,5 a./f.u., valeur calculée sur la base de 22 oxygènes.
2.2.2 Domaines des biotites transformées et/ou néoformées
Ces biotites ont des compositions telles que leurs points représentatifs se situent entre la limite précédemment établie et la base FeO*–MgO du diagramme (domaines B et C, Fig. 3). Outre les différences de teneurs en TiO2, les domaines B et C se caractérisent par les critères morphoscopiques, optiques et paragénétiques suivants :
- – dans le domaine C, toutes les biotites sont en lamelles néoformées, soit en croissance épitaxique sur des biotites préexistantes, soit en remplacement total ou partiel d'autres minéraux ferro-magnésiens (olivine, cordiérite…), soit en néogenèse dans des pores ou des microfractures. Dans le cas du remplacement de minéraux ferromagnésiens (olivine, cordiérite, amphibole), ceux-ci présentent des valeurs de identiques ou très voisines de celles des biotites néoformées (étoiles du diagramme, Fig. 3) ;
- – dans le domaine B, avec des compositions chimiques analogues, peuvent coexister des biotites dont les caractères morphoscopiques et optiques sont identiques ou voisins de ceux des biotites du domaine A ou du domaine C.
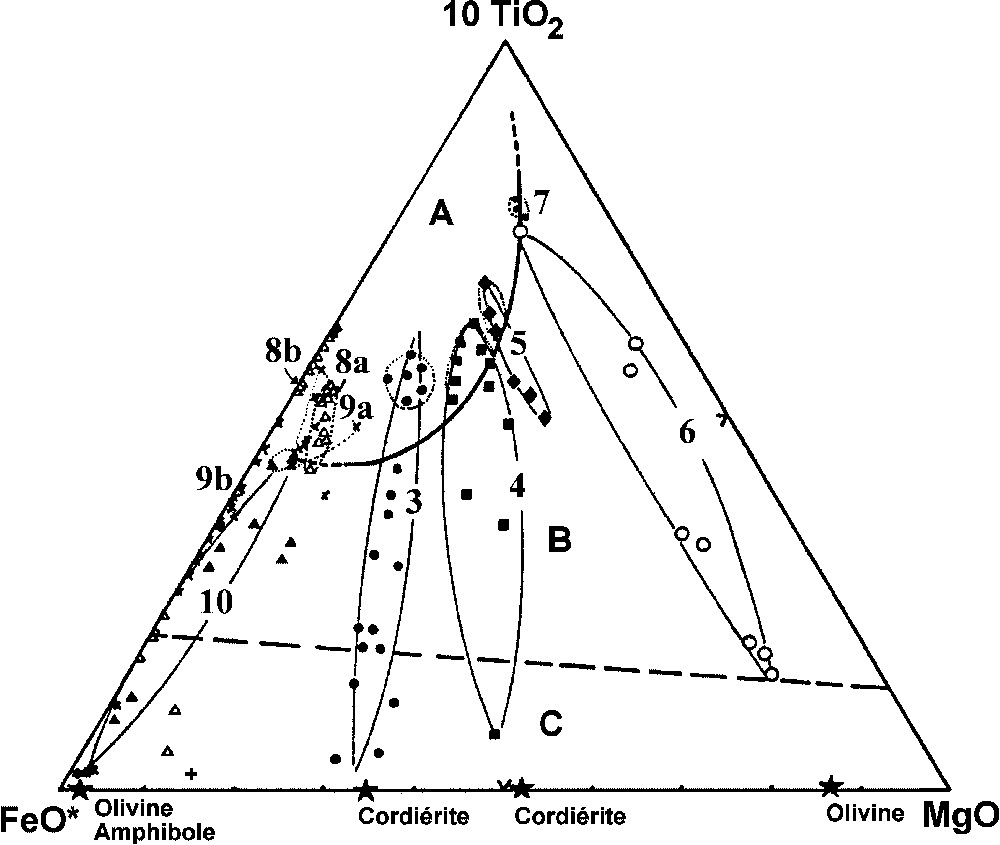
Domains of the reequilibrated and neoformed biotites. (A) Domain of the primary magmatic biotites, (B) domain of the reequilibrated biotites and (C) domain of the neoformed biotites. The numbers of the areas are the same as for Fig. 2. The stars correspond to FeMg minerals that coexist with the biotites.
Domaine des biotites transformées et/ou néoformées. (A) Domaine des biotites primaires magmatiques, (B) domaine des biotites transformées (rééquilibrées), (C) domaine de biotites néoformées. Les numéros des champs correspondent à ceux de la Fig. 2. Les étoiles correspondent aux minéraux ferromagnésiens coexistant avec les biotites.
3 Interprétation et conclusion
La variation de la teneur en titane de biotites à pratiquement constant pour chaque famille de roches magmatiques est le reflet de transformations progressives, partielles à totales, voire de néoformations dans un fluide caractéristique de cette roche. De fait, dans la plupart des cas, on constate que les directions dans lesquelles les compositions chimiques des biotites évoluent au cours de l'altération tardi- à post-magmatique (ou au cours de la néoformation) sont imposées par la composition d'autres minéraux ferromagnésiens primaires, coexistant initialement avec la biotite. On peut donc conclure que l'évolution de la composition des biotites à des stades tardi- à post-magmatiques s'effectue en général selon un rééquilibrage progressif, plus ou moins complet selon les cas, avec un fluide hydrothermal dont la composition est influencée par un ou des minéraux ferro-magnésiens primaires coexistants ; ces derniers sont eux-mêmes partiellement ou totalement déstabilisés.
Il résulte de ce qui précède que seules les biotites appartenant au domaine A du diagramme proposé peuvent être raisonnablement utilisées à des fins pétrogénétiques relatives aux conditions initiales de la cristallisation magmatique, celles des autres domaines (B et C) n'étant que le reflet de modifications tardives.
1 Introduction
The chemical composition of biotites is classically used to estimate the conditions in which their magmatic host-rocks were generated: oxygen fugacity in the parent magmas [13,16], liquidus temperature [1], to classify the granitoid [2,12] as well as to date the thermal events registered by these rocks.
This necessarily implies that the studied biotites are really primary magmatic and thus that their chemical compositions reflect magmatic conditions. Within a single granitic massif, for a specific facies and for biotites considered as primary from petrographical criteria, the chemical composition of these micas shows great variations. Since these variations are the consequence of late and/or post-magmatic hydrothermal alterations, they clearly restrict the use of the chemical composition of biotites for the above-mentioned purposes.
In this paper, we therefore propose a reliable tool to discriminate between the primary magmatic biotites and those that show evidence of: (i) a more or less complete reequilibration within a late-magmatic hydrothermal fluid, (ii) a neocrystallization at equilibrium with that fluid, or in another even later one.
This tool is a ternary diagram based on the contents, in biotites, of certain major elements (TiO2, FeO, MnO, and MgO) that are easily measured by electron microprobe analyses.
2 Construction of the TiO2–FeO*–MgO ternary diagram
2.1 Selection of the discriminating elements
Among the major constituent elements of biotites (Si, Al, Fe2+, Fe3+, Mg, K, Ti), four oxides FeO* (FeO* = FeO + MnO), MgO and TiO2 were selected for the following reasons:
- – the two end-members of biotites are phlogopite (Mg) and annite (the Fe2+ equivalent). The FeO*/(FeO* + MgO)} of biotites depends on that of the host-rock; it increases from in basic rocks to in felsic ones [3];
- – the Ti content of biotites is thermo-dependent [15], and varies with the of the host-rock [3]. In altered zones of porphyry copper deposits, the decrease of the Ti content in hydrothermal biotites (as compared earlier to previous magmatic ones) is linked to these conditions [7,8];
- – the alteration of biotites, already visible by optical microscopic methods (discolouring, pleochroism decrease, etc.) is frequently associated with a neocrystallization of FeTi oxides (rutile, anatase, ilmenite).
Consequently, the base of the ternary diagram with FeO* and MgO pole makes it possible to distinguish biotites originating from felsic series (FeO*) and mafic series (MgO). For specific , the TiO2 content is therefore dependent on the formation or transformation temperatures of the biotites.
In fact, 480 chemical compositions of biotites from various origins, compiled from the literature or determined in the course of this study, were found to have a nearly homogeneous distribution across the whole diagram (Fig. 1).
2.2 Limits of the domains for magmatic, reequilibrated and neoformed biotites
2.2.1 Magmatic biotite domain
As shown in Fig. 1, there is no wide gap in the TiO2 contents clearly dividing the domain of the magmatic biotites from that of the transformed or neoformed ones. This limit (Fig. 2, domain A) has therefore been determined according to the criteria defined by Monier [9] and related to the coexistence of biotites with very early high-temperature muscovites (>650 °C), which are considered to have crystallized during the very last stages of magmatic solidification.
The biotites that are considered to be primary magmatic are grouped in field 2 of domain A; they are in equilibrium with muscovite in field 1. Thus, the lowest TiO2 contents determine the lower limit of existence of magmatic biotites for ranging from 0.70 to 0.85.
For lower values, we selected biotites from certain magmatic series [10,11], with paragenetic, optical and morphological characteristics similar to those of the first group: euhedral crystals, strong brown colour and strong pleochroism, lack of Ti oxides. These biotites are plotted in fields 3, 4 and 5, and correspond to ranging from 0.50 to 0.80. For decreasing values of , the limit of the primary magmatic biotite field is strongly tilted toward the TiO2 pole of the diagram.
Only a few biotites with (in fact, near 0.10), are available: primary biotites from mafic rocks without secondary alterations (point 7) [5] and secondary biotites from mafic rocks but crystallized at very high temperatures (>1000 °C) [14].
For the lowest values of , the field has therefore been extended towards the TiO2 apex, taking in the most Ti-rich biotites of the mafic and ultramafic host-rocks. Beyond, the limit is less reliable and represents no more than an extrapolation toward the TiO2 apex of the diagram.
The values above 0.85 belong to biotites either from strongly evolved leucogranites (calc-alkaline or AlK series) or from alkali-granites (fields 8, 9 and 10). For values above 0.96, the limit of domain A is defined by the compositions of primary biotites from alkali-granites from Corsica (field 8) [4] and Nigeria (field 9) [6].
All biotites plotted in domain A present common physicochemical characteristics compatible with their equilibrium with a magmatic liquid: high TiO2 content close to the maximum solubility in plutonic rocks [7], low to zero content of [6]Al [11,12] (the highest contents of [6]Al in biotites from AlK granites do not exceed 1.5 a./f.u., value calculated on the basis of 22 oxygens).
2.2.2 Domains of reequilibrated and neoformed biotites
The composition of these biotites plot between the defined limits of domain A, and the base of the ternary diagram (domains B and C).
Considering the magmatic suites described above, we note that, for each of them, the compositions of biotites are arranged in narrow -specific trends (Fig. 3); in fact, for each series, does not remain strictly constant with decreasing TiO2, but is slightly lowered (series 4, 5, and 6, 7). For biotites from alkali-granites, remains much more constant (series 9) or, by contrast, slightly increases (series 10).
This second part of the diagram shows two domains (B and C); they correspond to TiO2 content variations and to paragenetic, optical, and morphoscopic characteristics of the biotites. With decreasing the TiO2 contents, the brown colour becomes lighter (biotites may become pale green), their pleochroism is weaker and titanium-bearing oxides appear:
- – in domain C, all biotites are individual neocrystallized lamellae in the form of: (i) epitaxic growth on early biotites, (ii) total or partial replacement of these biotites or other FeMg minerals, (iii) neocrystallization in cracks and pores. In the case of the replacement of earlier FeMg minerals (previous pyroxenes, olivines, cordierites, amphiboles) (stars in Fig. 3), reflects of these minerals have the same than the neoformed biotites;
- – in domain B, biotites with identical chemical compositions and optical properties similar to those in domain A and/or C may coexist.
The biotites from domains B and C always have a high [6]Al content (1<[6]Al based on 22 oxygens) [10].
3 Interpretation and conclusion
Transformations and neoformations of biotites are classically interpreted as resulting from hydrothermal alterations associated with the circulation of various late- to post-magmatic fluids. The variation of TiO2 content in biotites, with nearly constant for each series of magmatic rocks, indicates progressive partial to complete transformations, by and/or within a fluid buffered by the whole rock composition. In most cases, the evolution of the biotite compositions (increasingly altered to neoformed) is governed by that of another pre-existing FeMg mineral, which may have been partially or totally replaced.
In all cases described here, the of the alteration fluid seems to be controlled by that of the FeMg minerals of the altered rocks nearby.
To conclude, the use of the ternary diagram TiO2–FeO*–MgO is a necessary preliminary to the typological study of granitoids based on the biotite chemistry. Electron microprobe analysis of these minerals is a quick and easy method, allowing on this basis, the distinction between unaltered primary magmatic biotites and more or less reequilibrated, possibly neoformed ones by post-magmatic hydrothermal fluids.