1 Introduction
Carbonate, sulphide, sulphate, phosphate and silica concretions, which occur in a variety of geological environments (e.g., lacustrine and marine sedimentary rocks), have been studied in a number of research programmes over the several last years [55]. Concretions record a short moment in the chemical progress of pore water, which becomes saturated with respect to the minerals that make up the concretion. They are said to be products of an early-diagenetic process. Sulphide concretions have been studied less than the others, especially from a morphological standpoint.
Our research has focussed initially on sulphide occurrences in the Germav Formation, in southeastern Turkey (Fig. 1). The sulphides therein predominantly comprise pyrite and marcasite. Pyritised plant and animal fossils, and interestingly shaped pyrite concretions (likely related to bioturbation structures) have been observed in the study area. In this article, the morphologies and mineralogical features of the pyrite concretions and their major- and trace-element contents have been considered. The overall major aspects of the pyrite concretions and pyritised plant, fish and trace fossils have been studied collectively in this research, thus differing from most other work on pyrite.

General geological map of the district.
Carte géologique général de la zone étudiée.
Pyrite is a mineral widespread in almost all geological environments. However, studies that have been carried out on pyrite occurrences in sedimentary environments have typically not emphasised morphology [1,4,5,11,12,16,26,27,32,36,37,41,60–65]. The most widely accepted view is that euhedral and framboidal pyrite forms in sedimentary environments under anoxic conditions.
2 Geological setting
The sulphide-bearing units considered in this study belong to the Upper Cretaceous Germav Formation (Fig. 1) [24]. The Germav Formation of southeastern Turkey is of varying thickness (35 to 1325 m) and is exposed over rather large areas [15]. The Germav Formation generally comprises siltstone and sandstone alternating with greenish–grey shale and marlstone [66] and, locally, with conglomerate and detrital limestone [24]. Güven et al. [25] concluded that the Germav Formation formed in slope areas and submarine fans. Our own work in the same area corroborates that interpretation.
The Germav Formation comprises marlstone that contains the main pyrite concretions and bioturbated claystone that contains pyritised plant material (Fig. 2). At some levels, the unit is represented by chert-nodular limestone overlain by very thin sandstone layers. The sequence of claystone and marlstone layers reaches an apparent thickness of 150 m. Individual depositional layers have thicknesses between 50 and 100 cm. Sulphide-rich horizons, approximately 10-cm thick, occur locally within the sequence, and plant accumulations are also present in these sulphide-rich intervals.
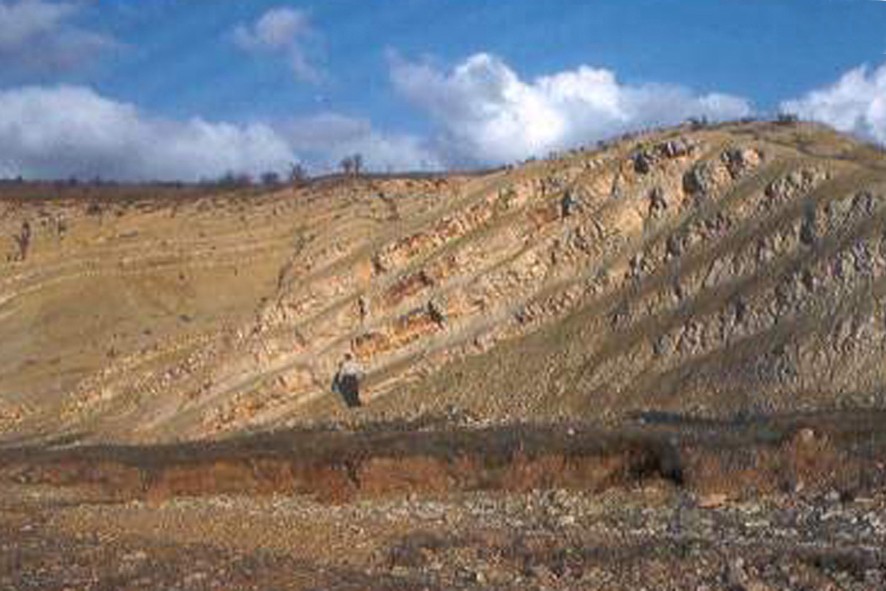
Marlstone and bioturbated claystone succession.
Succession de marnes et argilites à bioturbidites.
The following fossils have been identified in the course of studies of samples taken from the Germav Formation. Globotruncana linneiana (d'Orbigny), Globotruncana arca (Cushman), Globotruncanita stuarti (de Lapparent), Globotruncanita cf. stuartiformis (Dalbiez), Globotruncana cf. falsostuarti (Dalbiez), Globotruncana cf. Aegyptiaca (Nakkady), Globotruncanita sp., Globotruncanella sp., Rosita fornikata (Plummer), Rosita sp., Gansserina gansseri (Bolli), Heterohelix globulosa (Ehrenberg), Heterohelix sp., Heterohelicidae, Abathomphalus mayaroensis (Bolli), Abathomphalus sp., Pseudotextularia elegans (Rzehak), Praeglobotruncana sp., Rugoglobigerina sp., Globigerinelloides sp. This fossil content indicates that the Germav Formation is of Late Maastrichtian age.
3 Materials and methods
The concretions related to pyritised plant remains, animal fossils and bioturbation structures examined in the present work were observed at different levels of the marl–claystone sequence. These occurrences are mostly oxidized as a result of surface alteration in the places from which samples were taken. In the study area, a tunnel has been dug for irrigation purposes at a depth of approximately 150 m below the marl–claystone sequence. It is possible to reach that tunnel via subtunnels from different points. Our samples were taken during excavation of the subtunnels. Therefore, the samples taken were fresh rock and sulphide samples that had not been subjected to any surface alteration.
The sulphide and various host-rock samples were studied microscopically. Thirty samples with concretionary characteristics, with varying proportions of pyrite and cement, were chemically analysed by ACME Laboratories, Canada. Inductively coupled plasma mass spectrometry (ICP-MS), inductively coupled plasma optic emission spectrometry (ICP-OES) and neutron activation analysis (NAA) were used to analyse the trace-element contents of the concretionary pyrite samples.
4 Sulphide occurrences and the morphological classification of pyrite
Various types of sulphide occurrences are abundant in the lithostratigraphic succession of marlstone, claystone and sandstone in the Germav Formation. These were studied in four groups: layered and lensoidal pyrites; pyritised plant and animal fossils; concretionary and authigenic pyrites.
4.1 Layered and lensoidal pyrites
Layered pyrites reach thicknesses of 10 cm, but there are also laminar intervals only a few millimetres in thickness near the marl–claystone contact. These occurrences are mainly fine-granular and massive, but there is also lesser disseminated pyrite. The lensoidal pyrites were also deposited as concordant layers, a few millimetres in thickness, in the sandy marls. Pyrites tend to either partially or completely enclose sandstone granules. In one place, another pyrite zone developed around pyrite that previously developed around a granule. There are also rounded pyrite granules. In most cases, the structure wraps around the sandstone granules, and the fossils are considered to be micro-concretions that did not develop fully. Such pyrite occurrences are considered to have formed in either the sedimentary or early-diagenetic stages.
4.2 Pyritised plant and animal fossils
While pyritised plants are found only in the claystone layers, pyritised animal fossils are concentrated in the marls. Pyritised animal fossils are more abundant in particular marl horizons. Of particular note are partially pyritised and rather well-preserved fish fossils, and non-pyritised macrofossils such as gastropods and bivalves. An abundance of pyritised plant branches and leaves are present in claystones at the base of the sequence. These plant-fossil accumulations comprise branches, 1–2 mm in diameter and 10–15 cm in length, or tree (trunk) pieces, 5 mm in diameter and 10 cm in length, with almost completely preserved bark and probable leaf pieces. The pyrites in the branches and leaves comprise rather fine granules. Some gastropod fossils are completely pyritised; however, the species have not been determined exactly. Moreover, some completely pyritised Globotruncana fossils occur in rings of cement in the concretionary pyrites (Fig. 3).
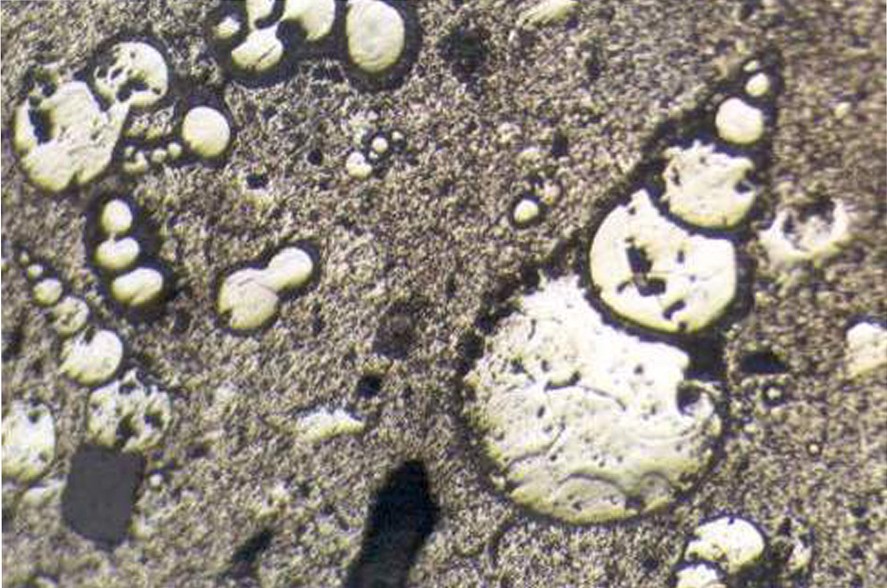
Completely pyritised Globutruncana fossils in rings formed by cements of concretionary pyrites.
Fossiles complètement pyritisés de Globutruncana, en anneaux formés par des ciments de pyrite concrétionnée.
4.3 Concretionary pyrites
In the study area, the most widespread sulphide occurrences are concretions. The pyrite concretions are most abundant within shale that contains abundant organic material, and concretions also occur in marl intervals of the sequence that belongs to the Germav Formation. Such concretions developed in bioturbation structures and on transported tree fragments, and have tubular, spherical-ellipsoidal and cylindrical morphologies. The pyrite concretions formed when sulphide-bearing solutions filled cavities left by marine invertebrates that moved at or near the sediment–water interface during or just after sedimentation. Heterogeneity of the sediments may have played a role in the formation of the concretionary pyrites, as has been proposed for other concretions [8,9].
In the study area, tubular concretions are most abundant. These features are mostly perpendicular to but locally parallel layering; locally they are connected, but most are isolated from one another. The widths of the burrows vary from a few millimetres to 5 cm, and the lengths reach perhaps 3–4 cm and typically have an annular appearance. The rings are made up of massive pyrite and varying proportions of minerals and cements derived from the marls in which they are found. The inner parts of these concretions comprise repeating rings of fine granular and radial pyrite–marcasite crystals. Larger radial crystals occur in the outer parts of most specimens. The external surfaces of some specimens have been observed. These features were produced as a result of pyrite-filling of cavities produced by movement of organisms towards the sediment–water interface near the end of sedimentation and immediately prior to compaction. The external parts of some concretions have extended into the surrounding rock, and beautiful octahedral crystals have formed in these areas. Thus, the external parts of the concretions have a zigzag appearance (Fig. 4). These features form due to pressure of the developing crystal(s) onto proximal material at the outset of compaction. According to Seilacher [54], the pressure effect on the environs was probably obtained by means of pressurizing liquid cell or film of overpressured fluid in the development of the crystals.

Pyritic concretions showing ring-shaped, ‘zigzag’ occurrence due to octahedral crystal growth in the external part of a concretion.
Concrétions pyriteuses annulaires, montrant un contour en « zigzag » en raison d'une croissance de cristaux octaédriques dans la partie externe de la concrétion.
Another commonly observed concretion type is the spherical-ellipsoidal. Such concretions occur in marls with normal bioturbation and average carbonate contents. Locally, in some horizons, these types of concretions occur with chain-like forms, but most are independent of one another. In some cases, they are partially or completely rounded, but most are ellipsoidal. These concretions develop in cavities produced during vertical movement of organisms. The actual upward movement of organisms is apparently recorded locally. Spherical-ellipsoidal concretions comprise concentric rings 1 to 6 cm in diameter; such rings are largely persistent, but locally thin, and disappear. Each ring, more or less, presents an isotropic structure. Massive, fine-granular and radial pyrites occur, and rings made up of rock cements/matrices containing pyritised macroorganisms and microorganisms repeat once or more. On the other hand, there are concretions of this type made up only of pyrite and marcasite. These rings are single radial rings up to 1 cm in diameter, or multiple radial and random pyrite rings, 4 cm in diameter. Such concretions are generally accepted to have formed in the early-diagenetic stage [54]. When the mud is soft and still isotropic, a spherical lump around the burrow structure develops due to the addition of a layer, probably from the outside.
Abundant in the study area are cylindrical pyrite concretions that developed around transported tree fragments. Such concretions are 0.5 mm–1 cm in diameter and are an average of 4–5 cm in length (Fig. 5). The external surfaces of such concretions, up to 10 cm in length, have a tree bark-like appearance (Fig. 6). These concretions are made up only of pyrite and marcasite, and are characterised by an annular inner structure. Relatively well-preserved tree aspects indicate that such concretions may have formed in the early-diagenetic stage.

Pyrite concretions developed around transported tree fragments.
Concrétions de pyrite développées autour de fragments transportés d'arbres.

Concretions having a tree bark-like appearance.
Concrétions dont l'aspect rappelle une écorce d'arbre.
4.4 Authigenic pyrites
These pyrites are fracture-filling euhedral pyrites that probably formed both in the early- and late-diagenetic stages. While the euhedral pyrites of the early-diagenetic stage are abundant in the claystone intervals of the sequence, the late-diagenetic euhedral pyrites are mostly observed within the marl.
The early-diagenetic euhedral pyrites comprise cubic pyrite crystals varying from 10 μm to 1 cm in length, and typically occur as crystal accumulations, grown one over the other. Locally, concretions made up of rather fine radial pyrites become larger cubic pyrite crystals, reaching almost 1 cm in diameter, due to continuing growth. Such a situation reflects development of the local euhedral pyrite crystals, syngenetic with the concretions.
Late-diagenetic euhedral pyrites have cubic and octahedral forms. The fractures they fill resemble rosary beads arranged on a string (Fig. 7). These euhedral pyrites comprise diagenetic pyrites – redissolved and recrystallised in cracks and fractures after the compaction of the rocks – and thus are clearly post-sedimentation features.
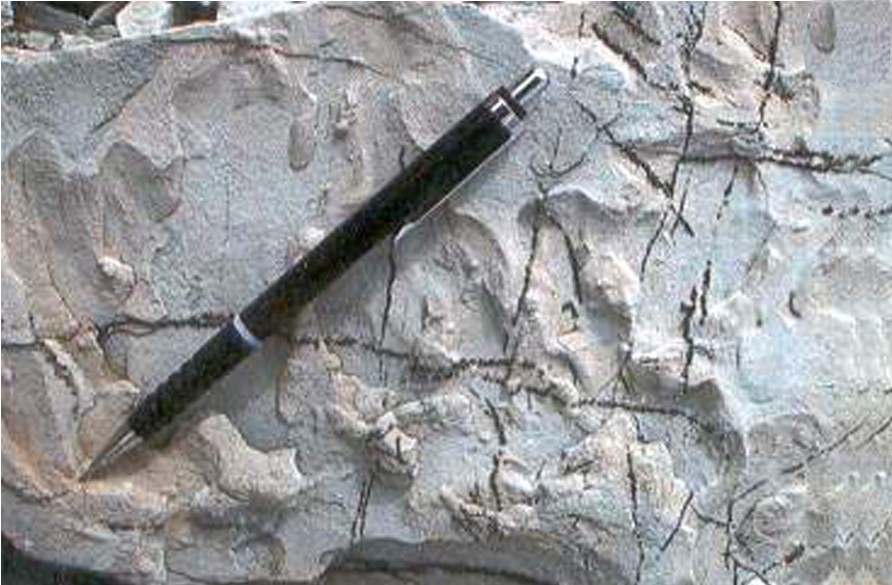
Late diagenetic or epigenetic euhedral pyrite.
Pyrite euédrique de diagenèse tardive ou d'épigenèse.
5 Mineralogical investigation of the sulphide mineral occurrences
During the course of reflected light microscopy of the concretionary and other sulphide occurrences, pyrite and marcasite were recognised as the dominant minerals. Bravoite, millerite and their alteration product, violarite, were identified in the central parts of some concretions.
Pyrite The most abundant sulphide mineral in the study area, pyrite is mainly observed in concretionary form, but also occurs as cubic and octahedral crystals, varying in size from a few microns to a few millimetres (coarse granules). The concretionary pyrites mainly possess radial textures.
Marcasite The second most abundant sulphide mineral in the study area is marcasite, and it typically occurs with interpenetrating texture. Strong colour anisotropy of the marcasites (green, brown, grey) facilitates their recognition. Some of the marcasites are partially or completely altered to violarite.
Marcasite is a form of FeS2 that forms at low temperature and under acidic conditions [13]. Marcasite crystallises more readily than pyrite at low pH (less than 5) and at low temperatures (under 75 °C) [40]. Various researchers report that marcasite forms via conversion of the leading ferrous sulphur, and that marcasite is not directly precipitated from the solution. Benning et al. [3] pointed out that in the formation of the marcasite, polysulphide concentration (more than 10−5 to 10−6 molar) is important, as are pH and temperature. Marcasite generally forms as a replacement of concretions or fossils in adequately reducing environments; however, it may also form by conversion of pyrite at the onset of very acidic conditions. In such cases, pyrite and marcasite may be intergrown [58].
Bravoite Lesser amounts of bravoite are encountered in some samples. Generally, pyrite and locally marcasite seem to have a zoned structure. Pyrite may accommodate Ni and Co in the Fe position of the crystal structure. Increase in Ni allows crystallisation of bravoite, and unit cell size also increases in response to increasing Ni content [58].
Millerite (NiS): Another sulphide mineral present in minor amounts is millerite, which mainly occurs as elongate rods. Locally violarite (Ni2FeS4) is an alteration product at grains margins and as plates. It is thought that millerite formed by alteration of nickeliferous pyrites instead of by direct precipitation from pore waters.
6 Chemical composition of the pyrite concretions
Various trace elements may be concentrated in pyrites of different origin [16]. The concentration of metals in pyrite depends on the concentrations of the various metals in the precipitation environment. Metals that may circulate in a hydrologic column become fixed in sediments rich in organic material [51].
Many elements, such as Cu, Zn, As, Sb, Ni and Co, may be highly concentrated in sedimentary rocks, especially in anoxic sediments [63]. For example, jointly precipitated As and Co may be concentrated in Fe-sulphides in such environments [33,57]. The enrichment of Cd in anoxic sediments is clearly related to the sulphide fraction [31,56]. Furthermore, Zn is related to the sulphides in sediments [19], and significant Ni also occurs in sedimentary pyrites [35,44]. Stoichiometrically, Ni and Co in pyrite replace ferrous iron, and Se and Te replace sulphur [30]. In fact, these elements tend to be concentrated in pyrite and marcasite, the main sulphide phases in such environments [51].
Trace elements in pyrite, such as Ag, Pb, Cu, Mn, Ti and Ni, may aid in elucidation of ore genesis [49]. However, the Co and Ni contents of pyrites or, more specifically, their Co:Ni ratios, are primarily used as indicators of ore genesis [7,22,23,34,50,62]. Hydrothermal pyrites have extremely variable Co and Ni contents and rather variable Co:Ni ratios. Pyrites in massive sulphide deposits have Co:Ni ratios of 5–50 (average 8.7), with high Co contents (average 480 ppm) and Ni contents less than 100 ppm (average 56 ppm) [7]. Sedimentary pyrites in shale have high Ni contents [34] and low Co:Ni ratios (average 0.63) [7].
Our analytical results from the concretions (Tables 1 and 2) indicate that mineralogical components other than sulphide phases (pyrite ∼80%) are also present in significant amounts (∼20%). Major oxides, such as Al, Ca, K, Mg, Na and Si (not analysed, probably) may have been derived from the host-rock cement that binds the concretions. Apart from the sulphide cements, the clay-fraction minerals comprise carbonates and probably quartz grains. In addition to those major oxides, trace elements of lithophilic character, such as Zr and Sn, may have been concentrated in other (non-sulphide) components within the concretions. Of course, elements such as Ni, Co, Zn and Cu tend to be enriched in sulphide minerals [19,28,29].
Major-element contents (in wt%) of pyrite concretions
Teneur en éléments majeurs (en poids %) des concrétions pyriteuses
Samples | Al | Ca | K | Mg | Na | P | Ti | Fe | S |
B-1 | 0.3 | 0.31 | 0.10 | 0.04 | 0.042 | 0.002 | 0.024 | 44.0 | 42.5 |
B-2 | 0.4 | 0.25 | 0.13 | 0.05 | 0.026 | 0.003 | 0.032 | 41.6 | 38.9 |
B-3 | 0.32 | 0.07 | 0.09 | 0.03 | 0.028 | 0.001 | 0.027 | 39.8 | 36.4 |
B-4 | 0.26 | 0.44 | 0.08 | 0.03 | 0.038 | 0.005 | 0.022 | 39.7 | 36.8 |
B-5 | 0.30 | 0.34 | 0.09 | 0.04 | 0.024 | 0.005 | 0.029 | 39.0 | 35.8 |
B-6 | 0.36 | 0.55 | 0.11 | 0.04 | 0.037 | 0.009 | 0.030 | 38.6 | 37.1 |
B-7 | 0.18 | 0.12 | 0.05 | 0.02 | 0.022 | 0.006 | 0.015 | 40.2 | 37.7 |
B-8 | 0.26 | 0.30 | 0.09 | 0.03 | 0.030 | 0.008 | 0.023 | 38.8 | 36.6 |
B-9 | 0.23 | 0.29 | 0.07 | 0.03 | 0.026 | 0.007 | 0.020 | 40.3 | 38.0 |
B-10 | 0.27 | 0.26 | 0.09 | 0.04 | 0.030 | 0.002 | 0.026 | 39.8 | 37.1 |
B-11 | 0.26 | 0.11 | 0.08 | 0.03 | 0.028 | 0.004 | 0.024 | 40.0 | 37.2 |
B-12 | 0.21 | 0.39 | 0.07 | 0.03 | 0.031 | 0.008 | 0.021 | 41.1 | 39.0 |
B-13 | 0.16 | 0.17 | 0.05 | 0.02 | 0.017 | 0.006 | 0.016 | 43.0 | 39.6 |
B-14 | 0.22 | 0.41 | 0.07 | 0.03 | 0.044 | 0.002 | 0.021 | 40.1 | 36.6 |
B-15 | 0.09 | 3.88 | 0.04 | 0.06 | 0.023 | 0.005 | 0.009 | 36.1 | 37.6 |
B-16 | 0.21 | 0.24 | 0.06 | 0.03 | 0.031 | 0.005 | 0.021 | 38.2 | 43.5 |
B-17 | 0.30 | 0.27 | 0.09 | 0.04 | 0.023 | 0.003 | 0.029 | 40.6 | 40.7 |
B-18 | 0.22 | 0.23 | 0.07 | 0.03 | 0.030 | 0.002 | 0.023 | 39.3 | 38.6 |
B-19 | 0.21 | 0.47 | 0.07 | 0.03 | 0.024 | 0.002 | 0.023 | 39.4 | 39.2 |
B-20 | 0.33 | 0.20 | 0.11 | 0.04 | 0.050 | 0.002 | 0.031 | 39.5 | 38.8 |
B-21 | 0.20 | 0.25 | 0.07 | 0.03 | 0.019 | 0.001 | 0.021 | 41.2 | 40.5 |
B-22 | 0.14 | 0.09 | 0.04 | 0.02 | 0.033 | <0.001 | 0.015 | 42.4 | 40.1 |
B-23 | 0.23 | 0.24 | 0.07 | 0.03 | 0.030 | 0.001 | 0.024 | 40.5 | 40.2 |
B-24 | 0.20 | 0.26 | 0.06 | 0.03 | 0.024 | <0.001 | 0.022 | 41.8 | 40.9 |
B-25 | 0.10 | 0.08 | 0.03 | 0.01 | 0.019 | <0.001 | 0.013 | 41.7 | 46.6 |
B-26 | 0.24 | 0.35 | 0.07 | 0.03 | 0.035 | <0.001 | 0.024 | 39.8 | 41.7 |
B-27 | 0.15 | 0.27 | 0.05 | 0.02 | 0.025 | <0.001 | 0.017 | 40.3 | 40.4 |
B-28 | 0.17 | 0.19 | 0.06 | 0.03 | 0.043 | 0.002 | 0.016 | 40.1 | 41.6 |
B-29 | 0.25 | 0.20 | 0.09 | 0.03 | 0.032 | <0.001 | 0.025 | 39.3 | 40.5 |
B-30 | 0.32 | 3.49 | 0.22 | 0.06 | 0.039 | <0.001 | 0.037 | 38.0 | 40.3 |
Trace-element contents (Au in ppb; others in ppm) of pyrite concretions. Italicised bold numbers indicate elements analysed by NAA
Teneur en éléments en trace contents (Au en ppb ; les autres in ppm) des concrétions pyriteuses. Les chiffres en italiques gras indiquent des éléments analysés par activation neutronique (NAA)
Samples | Au | Ag | As | Co | Cu | Mo | Ni | Pb | Zn | Ba | Cr | La | Mn | Nb | Rb | Sc | Sr | Th | V | Y | Zr |
B-1 | 6 | 0.1 | < 0 . 5 | 8 | 14 | 0.3 | 154 | 5.4 | 18 | 9 | 14 | 0.8 | 65 | 0.6 | 4.1 | 0 . 9 | 17 | 0.2 | 1 | 0.7 | 3.7 |
B-2 | 5 | 0.1 | 1 . 4 | 7 | 10 | 0.3 | 145 | 5.3 | 27 | 8 | 17 | 1.3 | 126 | 0.9 | 5.5 | 1 . 4 | 18 | 0.3 | 3 | 1.2 | 4.3 |
B-3 | 6 | 0.2 | 0 . 9 | 4 | 8 | 0.3 | 29 | 2.5 | 30 | 7 | 14 | 0.8 | 170 | 0.9 | 4.1 | 0 . 9 | 9 | 0.2 | 6 | 0.8 | 3.1 |
B-4 | 6 | 0.1 | 1 . 5 | 6 | 10 | 2.3 | 107 | 13.6 | 39 | 11 | 16 | 0.8 | 168 | 0.8 | 3.1 | 0 . 8 | 19 | 0.2 | 2 | 0.7 | 3.1 |
B-5 | 24 | 0.2 | 1 . 2 | 6 | 10 | 0.2 | 94 | 4.6 | 35 | 9 | 14 | 1.1 | 244 | 0.9 | 4.1 | 1 | 20 | 0.2 | <1 | 0.8 | 4.5 |
B-6 | 29 | 0.1 | 1 . 4 | 5 | 17 | 3.3 | 154 | 11 | 35 | 15 | 24 | 1.3 | 334 | 0.9 | 5.5 | 1 . 4 | 24 | 0.3 | 2 | 1.1 | 5.1 |
B-7 | <2 | <0.1 | < 0 . 5 | 4 | 5 | 0.1 | 36 | 2.5 | 18 | 6 | 8 | 0.6 | 62 | 0.4 | 2 | 0 . 7 | 9 | 0.1 | 2 | 0.5 | 1.7 |
B-8 | 10 | 0.1 | 0 . 9 | 5 | 12 | 2.3 | 63 | 10.1 | 28 | 8 | 17 | 0.9 | 152 | 0.8 | 3.8 | 0 . 9 | 16 | 0.2 | <1 | 0.6 | 3.6 |
B-9 | 7 | <0.1 | 0 . 9 | 6 | 6 | 0.2 | 98 | 2.7 | 27 | 9 | 12 | 0.7 | 131 | 0.7 | 3.4 | 0 . 9 | 15 | 0.2 | 2 | 0.9 | 2.8 |
B-10 | 5 | 0.1 | 1 . 1 | 5 | 12 | 2.5 | 112 | 11.8 | 29 | 21 | 18 | 0.7 | 163 | 0.6 | 4.1 | 1 | 16 | 0.2 | <1 | 0.8 | 4.7 |
B-11 | 9 | <0.1 | < 0 . 5 | 4 | 8 | 0.2 | 67 | 4.2 | 29 | 7 | 14 | 0.8 | 128 | 0.7 | 3.7 | 1 | 11 | 0.2 | 2 | 0.6 | 4 |
B-12 | 8 | 0.1 | < 0 . 5 | 7 | 10 | 3.8 | 96 | 5.9 | 30 | 9 | 18 | 0.7 | 147 | 0.6 | 2.9 | 0 . 8 | 17 | 0.1 | 1 | 0.5 | 2.7 |
B-13 | <2 | <0.1 | 0 . 9 | 4 | 6 | 0.1 | 71 | 3.3 | 18 | 7 | 9 | 0.6 | 46 | 0.5 | 2.8 | 0 . 6 | 10 | 0.1 | <1 | 0.6 | 1.6 |
B-14 | 10 | 0.1 | < 0 . 5 | 6 | 11 | 3.1 | 110 | 7.6 | 28 | 8 | 19 | 0.8 | 172 | 0.6 | 3.1 | 0 . 9 | 17 | 0.2 | <1 | 0.6 | 3.7 |
B-15 | 6 | 0.1 | < 0 . 5 | 3 | 4 | 0.2 | 68 | 6.1 | 15 | 11 | 6 | 0.7 | 59 | 0.3 | 1.5 | 0 . 5 | 86 | 0.1 | 1 | 0.8 | 1.6 |
B-16 | <2 | 0.1 | 1 . 2 | 6 | 10 | 3 | 54 | 4.8 | 18 | 5 | 17 | 0.7 | 69 | 0.6 | 2.9 | 0 . 7 | 12 | 0.1 | <1 | 0.5 | 3.5 |
B-17 | 10 | <0.1 | < 0 . 5 | 5 | 10 | 0.1 | 85 | 3.2 | 26 | 7 | 15 | 0.8 | 137 | 0.8 | 4.5 | 1 . 1 | 17 | 0.3 | 2 | 0.9 | 3.9 |
B-18 | 6 | 0.1 | 1 . 4 | 6 | 11 | 3.7 | 68 | 5.6 | 20 | 8 | 20 | 0.7 | 92 | 0.6 | 2.9 | 0 . 9 | 13 | 0.1 | 2 | 0.7 | 2.3 |
B-19 | 18 | 0.2 | 1 . 5 | 5 | 9 | 0.1 | 65 | 4.2 | 32 | 9 | 11 | 0.8 | 290 | 0.9 | 3.3 | 0 . 8 | 20 | 0.1 | 1 | 0.8 | 2.9 |
B-20 | 17 | 0.1 | < 0 . 5 | 6 | 12 | 2.9 | 68 | 4.6 | 29 | 7 | 25 | 0.9 | 264 | 1.1 | 4.8 | 1 . 4 | 14 | 0.2 | <1 | 1 | 6.9 |
B-21 | 5 | <0.1 | < 0 . 5 | 5 | 7 | 0.2 | 111 | 2.7 | 13 | 9 | 12 | 0.7 | 34 | 0.6 | 3.1 | 0 . 8 | 12 | 0.2 | 3 | 0.8 | 3.7 |
B-22 | <2 | 0.1 | < 0 . 5 | 5 | 17 | 3.5 | 107 | 6.5 | 17 | 6 | 14 | 0.4 | 70 | 0.4 | 2 | 0 . 5 | 6 | 0.1 | 3 | 0.4 | 2.9 |
B-23 | 16 | 0.1 | 0 . 9 | 4 | 7 | 0.1 | 80 | 3.2 | 31 | 8 | 13 | 0.8 | 201 | 0.9 | 3.2 | 0 . 8 | 14 | 0.2 | <1 | 0.7 | 3.4 |
B-24 | <2 | 0.1 | < 0 . 5 | 7 | 15 | 2.4 | 114 | 5.4 | 15 | 7 | 17 | 0.6 | 68 | 0.8 | 2.8 | 0 . 6 | 14 | 0.2 | <1 | 0.7 | 2.9 |
B-25 | 3 | 0.1 | < 0 . 5 | 4 | 12 | 0.1 | 56 | 3.6 | 16 | 5 | 6 | 0.3 | 33 | 0.5 | 2.1 | 0 . 4 | 4 | <0.1 | 2 | 0.2 | 2 |
B-26 | 18 | 0.1 | 1 | 5 | 10 | 3.5 | 81 | 3.6 | 28 | 8 | 22 | 0.7 | 153 | 0.7 | 4.6 | 0 . 8 | 16 | 0.2 | 1 | 0.6 | 3 |
B-27 | 11 | 0.1 | < 0 . 5 | 4 | 6 | 0.1 | 34 | 2.7 | 30 | 9 | 9 | 0.4 | 194 | 0.6 | 3 | 0 . 6 | 12 | 0.1 | <1 | 0.5 | 2.6 |
B-28 | 4 | <0.1 | < 0 . 5 | 4 | 8 | 2.4 | 56 | 4 | 23 | 9 | 14 | 0.6 | 96 | 0.4 | 2.3 | 0 . 7 | 11 | 0.1 | 1 | 0.6 | 2.7 |
B-29 | <2 | <0.1 | < 0 . 5 | 3 | 4 | 0.2 | 112 | 3.1 | 35 | 8 | 13 | 0.7 | 323 | 0.8 | 4.2 | 0 . 9 | 13 | 0.2 | <1 | 0.7 | 3.4 |
B-30 | 33 | 1.2 | 1 . 4 | 12 | 159 | 1.3 | 254 | 18.2 | 23 | 20 | 43 | 1.7 | 140 | 1.2 | 5.3 | 1 . 2 | 91 | 0.3 | <1 | 1.4 | 8 |
It is well known that Co and Ni are enriched in pyrite and marcasite in the sedimentary environment. In the studied concretions, pyrite, bravoite (the nickeliferous form of pyrite) and marcasite are the main sulphide phases. Millerite and violarite occur in trace amounts in only a few samples. In sedimentary pyrites, there is typically a strong correlation between Co and Ni (; [7]), but their correlation is weaker in the study area (), probably due to the fact that they were derived from local materials; alternatively, these metals may have been redistributed. Co values of the concretions are rather low (range 3–12; average 12), but Ni contents are rather high (range 29–253.7; average 91.54). The Co:Ni values (average = 0.06) of the concretionary pyrites from the study area are rather low compared to pyrites of other environments (Fig. 8). These data indicate that the studied pyrites are of sedimentary origin.
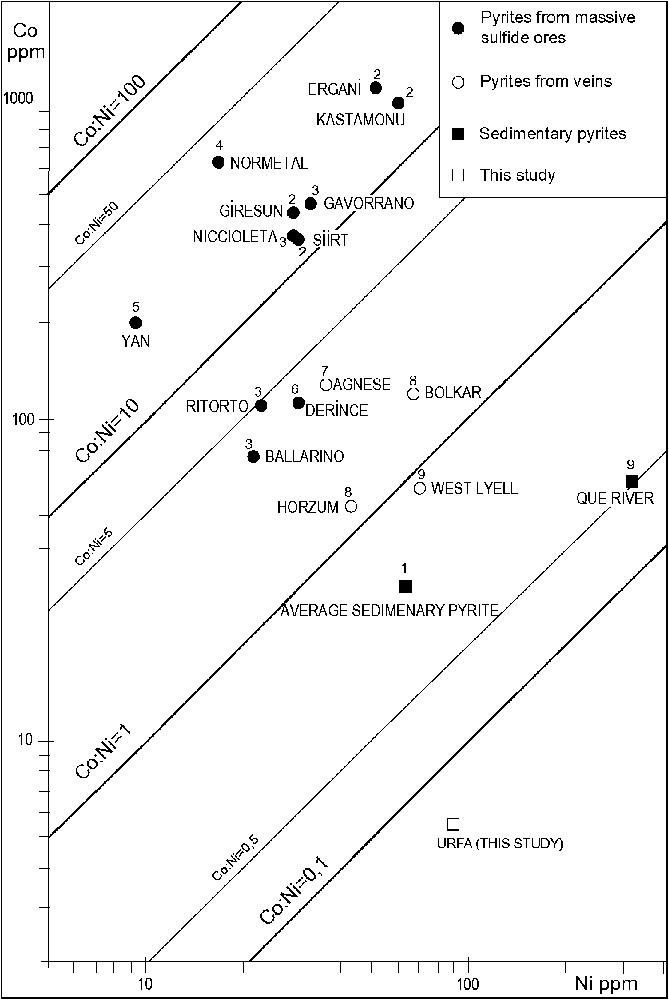
Co versus Ni plots of average values of pyrites from various ores. (1) Average of sedimentary pyrites (after [7]); (2) pyrites from massive sulphide ores, Turkey, Kastamonu 7 samples, Ergani 6 samples, Giresun 12 samples, Siirt 8 samples [23]; (3) Tuscany massive sulphide ores, Italy, Gavorrano 8 samples, Niccioleta 9 samples, Ritorto 107 samples, Ballerino 89 samples [7]; (4) Normetal, Canada, 12 samples (after [7]); (5) Yanahara, Japan, 19 samples (after [7]); (6) Derince, Turkey, 30 samples [6]; (7) Agnese, Italy, 6 samples (after [7]); (8) vein-type ores, Turkey, Horzum 32 samples, Bolkar 56 samples [62]; (9) West Tasmania, Australia, West Lyell, 8 samples, Que River, 9 samples [34].
Relations Co:Ni dans les teneurs moyennes des pyrites de minéralisations variées. (1) Moyenne des pyrites sédimentaires (d'après [7]) ; (2) pyrites d'amas sulfurés massifs, Turquie, Kastamonu 7 échantillons, Ergani 6 échantillons, Giresun 12 échantillons, Siirt 8 échantillons [23] ; (3) amas sulfurés massifs de Toscane, Italie, Gavorrano 8 échantillons, Niccioleta 9 échantillons, Ritorto 107 échantillons, Ballerino 89 échantillons [7] ; (4) Normetal, Canada, 12 échantillons (d'après [7]) ; (5) Yanahara, Japon, 19 échantillons (d'après [7]) ; (6) Derince, Turquie, 30 échantillons [6] ; (7) Agnese, Italie, 6 échantillons (d'après [7]) ; (8) minerais filoniens, Turquie, Horzum 32 échantillons, Bolkar 56 échantillons [62] ; (9) Tasmanie occidentale, Australie, West Lyell, 8 échantillons, rivière Que, 9 échantillons [34].
7 Discussion and conclusions
Pyrite assumes mainly framboidal or euhedral forms in sedimentary environments. Framboids form under acidic conditions [38] through precipitation [60] of micron-sized pyrite crystals. On the other hand, the euhedral pyrite comprises micron-sized crystals [41,42] that develop under neutral-alkali conditions [38]. Framboidal pyrites develop indirectly from ferrous monosulphides (amorphous FeS, mackinawite, greigite, pyrite) [53,60,64]. Such conversions occur through rather rapid processes [46]. Conversion from greigite into pyrite can occur either via addition of sulphur [4] or by loss of ferrous iron [20]; euhedral pyrites are formed from pore water saturated with respect to ferrous monosulphides [39,48]. Indirect crystallisation occurs via high activity of (dissolved) sulphides, and with the kinetic activity of ferrous monosulphides; framboidal pyrite develops in the early-diagenetic stage [17]. On the other hand, euhedral pyrite precipitates directly [21,45,53]. The euhedral pyrites are saturated with respect to pyrite, but are precipitated from pore water, which is not saturated with respect to monosulphides [47,63]. Euhedral pyrites form from pore water having depleted sulphide ratios in a late stage of early diagenesis [14], but form during early diagenesis when the sulphate reduction rate is low [61].
Framboidal pyrites have not been observed in the study area. However, it is thought that concretionary pyrite occurrences in the study area may have initially precipitated as framboids. Sawlowicz [51] points out that there are many similarities between concretions and framboids. According to that worker, probably in the early stages of concretion formation, the components (crystals) that make up the concretions have framboidal textures (polyframboids), or at least framboidal nuclei. The dimensions of framboids increase via the formation of pyrite euhedra.
A close relationship between pyrite formation and organic material was observed in the study area, as demonstrated by the presence of biogenic materials, such as animal macrofossils and microfossils, and fossilised tree branches and leaves, in bioturbated claystones and marls of the area. Indeed, organic materials in natural sedimentary environments are critical to pyrite crystallisation. The main source of sulphur for development of ferrous sulphides in sedimentary environments is H2S or HS− derived from bacterial sulphate reduction (Fig. 9) [4,51]. Round–oval masses observed in concretions are evidence of local sulphate reduction [52]. The bacteria that reduce sulphate do not precipitate sulphides directly; their role is probably limited to production of bisulphide ions [2]. Pyritised fossils, widespread in the study area, may be interpreted as the replacement of organic material by pyrite [10]. The replacement of soft parts occurs in sediments near the sediment–water interface during diagenesis, in which bacterial sulphate reduction is relatively slow and ferrous iron is dominant [13,43]. Moreover, few anaerobic environments are the most suitable for pyritisation of fossils [18].

Diagramatic representation of the process of pyrite formation (after [4]).
Diagramme représentant le processus de formation de la pyrite (d'après [4]).
The types of trace fossils that may have played important roles in the formation of pyrites in the study area could not be determined unequivocally. Ekdale and Bromley [17] pointed out that Upper Cretaceous marine-shelf chalks are rich in trace fossils; the most abundant were crustacean burrows (e.g., thalassonoids), but feeding/dwelling burrows of worms and other organisms (e.g., chondrites, zoophycos and planolites) were also quite widespread. Those researchers also reported that secondary mineralisation (replacement) of trace fossils is a typical characteristic of marine self chalks, and that pyritised trace fossils occur much more characteristically in marl–chalk facies (i.e., those rich in clay). However, Stow et al. [59] indicated that burrow types in deep water typically include zoophycos, planolites, and chondrites (ichnogenera). The sedimentary facies and trace-fossil contents of the Germav Formation suggest that it was deposited in a deep-sea environment.
The Co contents of the studied concretionary pyrites are rather low, but the Ni contents are high. The Co:Ni ratios of the pyrites are approximately 0.06. This value is rather characteristic of sedimentary pyrites. Furthermore, these data indicate that no magmatic or volcanic activity was operative in the formation of the pyrites. Moreover, there is no other evidence for magmatic or volcanic activity in this region.
In conclusion, we suggest that – on the basis of the sedimentological and stratigraphic features of the Germav Formation and the evaluation of the microscopic and geochemical data – the pyrite occurrences formed under anoxic conditions, through direct and indirect precipitation of seawater in association with organic material. The layered and lensoidal pyrites are syngenetic, the concretionary and authigenic pyrites are early diagenetic, and the fracture-fill pyrites are of epigenetic origin.
Acknowledgements
This study was funded by the Scientific Research Fund of Firat University (FÜBAP, Project No. 664). We are grateful to Prof. Dr. Izver Özkar (Istanbul University) for fossil determinations. We thank Dr. Steve Mittwede for critical reading and suggestions for improving early version of the manuscript.