1 Introduction
Active tectonic studies carried out during the last 20 years have permitted to complement the short time-window of instrumental seismicity providing key data on both the lithosphere deformation and seismic hazard in high-seismicity regions. In such regions, many source parameters have been estimated on seismogenic faults. Even if the seismic behaviour of such faults is still unconstrained, several models have been put forward [22]. In striking contrast, active tectonic studies have been only developed during the recent years in moderate to low-seismicity regions. Several key questions arise when analyzing seismogenic faults within slowly deforming regions; among them are the methodologies and models established for high seismicity regions appropriate for low to moderate-seismicity regions? To address these questions, a significant effort has been made by European scientists in the framework of the SAFE (Slow Active Fault in Europe; EVG1-2000-22005) European project [33]. One of the test areas of this SAFE project was the Moroccan High Atlas. Although outside of Europe, Moroccan High Atlas has three main interests: (1) to illustrate how is working presently such kind of intraplate mountainous system, (2) to determine the amount of shortening that is accommodated south of the African–Eurasian plate boundary region, and (3) to analyze a desert analogue of the Western European compressional seismogenic provinces; namely, Pyrenees and Western Alps. The aim of this paper is then to present a general overview of the active tectonic studies and first results that have been recently developed in the Moroccan High Atlas. It is based on both the reinterpretation of the existing literature and a synthetic presentation of newly acquired data.
2 Present-day geodynamic setting, seismicity, and GPS data
Moroccan seismicity is mostly localized in northernmost Morocco, in the Rif and Alboran Sea (Fig. 1). This agrees with the fact that this region corresponds approximately to the boundary between African (or Nubian) and Eurasian plates. According to Nuvel 1A and GPS results, plate convergence in the Alboran Sea Region trends roughly NW–SE, with a rate of motion calculated between 4–6 mm yr−1 [4,8]. This convergence trend is thus oblique with respect to the plate boundary, indicating that some right-lateral component of slip has to be accommodated in this boundary region. Even if the pattern of shallow focal mechanisms in the Alboran Sea is complex, showing reverse and normal oblique solutions as well as strike-slip ones (Fig. 1), it agrees with an oblique convergence characterized by transpressive deformations.
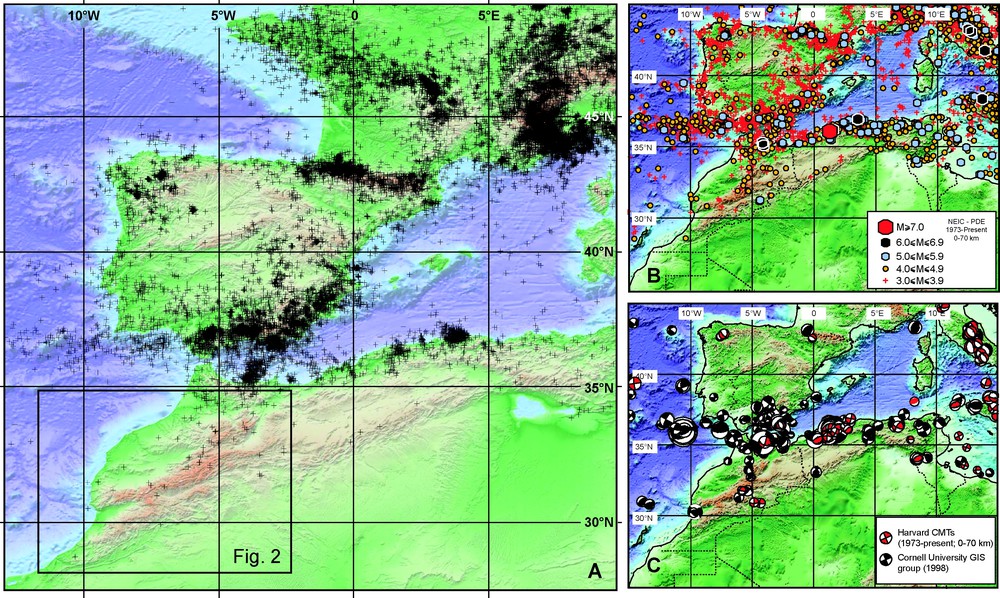
Present-day kinematics of the Western Mediterranean. A, B and C: Shallow seismicity maps of the Western Mediterranean using teleseismic data from NEIC (U.S. Geological Survey) catalogue (http://neic.usgs.gov/neis/epic/epic.html), Harvard's Centroid Moment Tensor Solutions (http://www.seismology.harvard.edu/CMTsearch.html), and focal mechanisms from other sources ([7] and references herein). Background digital topography: Shuttle Radar Topographic Mission composed image from Shuttle Radar Topographic Mission (ftp://e0mss221u.ecs.nasa.gov/srtm/srtm30) and bathymetry [35].
Cinématique actuelle de la Méditerranée occidentale. A, B et C : Cartes de la sismicité superficielle de la Méditerranée occidentale, basées sur les données télésismiques du catalogue NEIC (http://neic.usgs.gov/neis/epic/epic.html), Mécanismes aux foyers CMT de Harvard (http://www.seismology.harvard.edu/CMTsearch.html), et mécanismes aux foyers d'autres sources ([7] et références incluses). Fond topographique numérique : données SRTM (Shuttle Radar Topographic Mission, ftp://e0mss221u.ecs.nasa.gov/srtm/srtm30) et bathymétrie [35].
South of the Rif, Moroccan seismicity is lesser. However, a diffuse activity is still observed in the mountainous Atlas Region that spreads southward over the Middle Atlas, the High Atlas, and the Anti Atlas (Fig. 2). Further south, in the Sahara region, no seismic activity is observed and interestingly, seismicity also decreases eastward along the High Atlas, so that there is only very few seismicity along the Sahara Atlas in Algeria. As a consequence, the regional distribution of the Atlas epicentres appears to highlight a rough NE–SW trend, which is parallel to the Middle Atlas, but oblique to the WSW–ESE strike of the High Atlas (Fig. 2). According to the worldwide teleseismic catalogues, this Atlas seismicity corresponds mostly to events. Indeed, two events, which are localized on the eastern Anti-Atlas termination, have only been recorded during the last 40 years (Fig. 2). Considering a larger time-window, the largest earthquake ever recorded within the Atlas Region corresponds to the destructive 29 February 1960, Agadir earthquake, which produced 12 000 casualties with an [5,9,17]. The Atlas region is thus characterized by a moderate seismic activity, as most seismic areas of Western Europe.
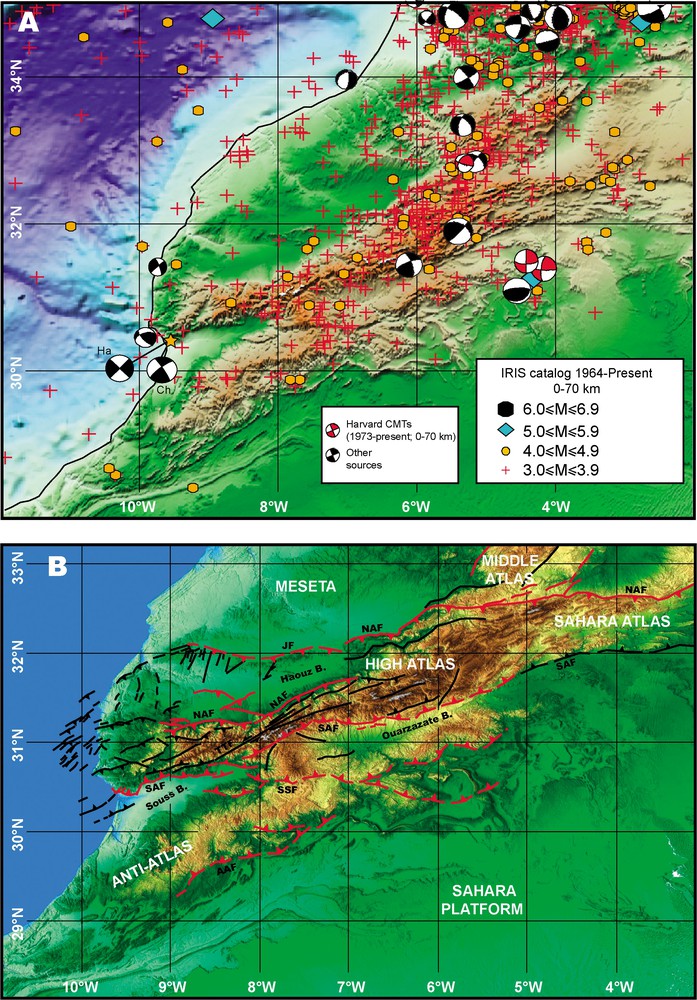
Seismotectonic model for the High Atlas Mountains. A: Shallow seismicity map of Morocco using teleseismic data from International Seismic Centre (ISC, 2001) catalogue, Harvard's Centroid Moment Tensor Solutions (see Fig. 1) and focal mechanisms from other sources [10,17,23]. Background digital topography: SRTM composed image and bathymetry (see Fig. 1). B: Structural map of southern Morocco. Active faults (solid lines) and supposed active (dotted lines) are mapped in red using heavy lines. AAF, Anti Atlas Fault; SSF, South Siroua Fault; SAF, South Atlas Fault; TTF, Tizi'N'Test Fault; NAF, North Atlas Fault; JF, Jebilet Fault. Background image SRTM90 digital topography (see Fig. 1).
Modèle sismotectonique de la région du Haut Atlas. A : Carte de la sismicité superficielle du Maroc, d'après les données télésismiques du catalogue ISC (International Seismic Centre, 2001), Mécanismes aux foyers CMT de Harvard (voir Fig. 1) et mécanismes aux foyers d'autres sources [10,17,23]. Fond topographique numérique : données SRTM et bathymétrie (voir Fig. 1). B : Carte structurale du Maroc méridional. Failles actives (lignes continues) et supposées actives (lignes pointillées) sont cartées en rouge vec un trait épais. AAF, faille de l'Anti-Atlas ; SSF, faille Sud Siroua ; SAF, faille Sud-Atlasique ; TTF, faille de Tizi'N'Test ; NAF, faille Nord-Atlasique ; JF, faille des Jebilet. Fond topographique numérique : données SRTM (voir Fig. 1).
Due to the above-mentioned characteristics, very few accurate focal mechanisms are available on the Atlas seismicity; overall, they indicate a present-day compressional regime with average NNW-trending P axes [6,23]. Nevertheless, depth uncertainties do not permit to relate easily these events with specific fault zones. Historical seismic data are scarce and highly incomplete for the High Atlas Region [11] and then, they do not permit to complement instrumental data to precise the active fault zones. Consequently, active tectonics and quantitative geomorphology are the only suitable approaches that may provide such time extension and allow us to locate and characterize the major active fault zones in the Atlas Region.
3 Structural and geophysical constraints
The High Atlas and part of the Middle Atlas correspond to an intra-continental mountainous system that developed on a former set of WSW–ENE and NE–SW striking Mesozoic grabens. These grabens were approximately initiated contemporaneously with the Trias to Lias rifting [20] that led to the opening of the Atlantic and Tethys Oceans. This area of Mesozoic thinned crust appears then to have behaved as a weakened lithosphere that has subsequently localized the compressional deformations during the Cenozoic inversion resulting from the Africa–Eurasia convergence. In addition, a younger weakening may be superimposed and caused by asthenospheric upwelling (see below); this would localize the rough NE–SW distribution trend of the present-day Atlas seismicity (Fig. 2), as discussed in the last section. The major High Atlas inversion occurred during Neogene and Quaternary and developed a bi-vergent mountain chain [13,15,19,36].
Geophysical studies have pointed out for many years that the topography of the Moroccan Atlas is not isostatically compensated by a significant crustal root [1,21,30,31,39]. Indeed, considering the High Atlas, which topography is locally over 4 km, its crustal thickness is no more than 34–39 km, with comparatively a 30–35-km-thick crust on both sides of the High Atlas; i.e., the Meseta to the north and the Anti Atlas to the south, respectively. In fact, a long wavelength component in the order of 600 km can be identified in the northwestern African topography [16]; this suggests that the Atlas topography should partly result from mantle processes as asthenospheric upwelling, which agrees with high heat-flow values [27,29]. On going numerical modelling aims to define the geometry of this mantle upwelling and to precise its contribution to the Atlas topography [37,40].
Shortening estimates based on structural studies also indicate that the Atlas topography cannot only result from crustal shortening. Indeed, shortening in the High Atlas, which is mainly localized on its southern and northern border faults, is overall small and ranges between 10 and 25% [2,3,26,36,41]. Moreover, no major Cenozoic compressional deformations are known in both the Anti Atlas [18] and the western Middle Atlas that might explain their nearly 2-km-high topography and even if some Cenozoic shortening may have taken place in these areas, it should be insufficient with respect to the existing topography.
Our own structural observations were carried out on both sides of the High Atlas from the Midelt–Errachidia transect (W) to the Atlantic coast (W) with the aim to study the main structures that were accommodating the present-day active deformation. We used available topographical, geological, and seismological maps and/or databases as well as satellite images (LANDSAT and SPOT). During our fieldwork, we revised five transects more or less orthogonal to the High Atlas: Midelt–Errachidia (W), Marrakech–Ouarzazate (W), Marrakech–Tizi n'Test (W), Argana (W), and Atlantic Coast (W). This transect revisions confirmed that most of the High Atlas shortening is accommodated by its southern and northern border faults and is controlled by two main décollement levels (see [1,2,14]): a shallow one, 1–4-km deep, at the base of the Mesozoic cover within the Triassic beds and a mid crustal one at a depth of about 10–20 km. From the seismotectonic point of view, the mid-crustal décollement should be the only one to have sufficient potential to generate destructive earthquakes, the cover–basement interface being too shallow to have such seismogenic potential. Our observations along strike on both sides of the High Atlas also confirmed that shortening is overall dissymmetric, the South Atlas Front having generally accommodated more Cenozoic shortening than the North Atlas one. Similarly, the southern front of the High Atlas also displays more evidence of active tectonic deformations than the northern one. Overall, it is also climatically more arid and consequently more favourable for performing active tectonic analyses.
Taking into account all the above-mentioned observations, we focused our detail analyses on the active tectonics of the South Atlas front within two areas where Cenozoic foreland basins were developed: the Souss Basin and the Ouarzazate Basin (Fig. 2). We focused our observations on the two existing foreland basins because the occurrence of Neogene and Quaternary deposits allowed us to analyse the cumulative deformation on a time scale large enough to account for the suspected slow deformation rates (see Section 2). These two foreland basins correspond to rather dissymmetric depressions with a gently northward warped southern side and a thrust bounded northern side. These basins are poorly developed as their Cenozoic sedimentary fill is of about 1 km. This relatively thin sedimentary aggradation indicates that the flexural subsidence was rather limited.
4 The Souss Basin
The Souss Basin is the westernmost depression that is located between the High Atlas and the Anti Atlas (Fig. 3). It extends on some 150 km from the Atlantic Coast to the western side of the Siroua Plateau and displays a rather flat topography, which increases progressively from sea level to 700 m. Its main drain, the Souss River, is localised in the middle of the depression due to alluvial aggradation that results from the erosion of both the High Atlas and Anti Atlas. The topographical dissymmetry is the consequence of tectonic activity, it is characterized by a steep northern border along the High Atlas and a gently north-dipping slope along the Anti Atlas. The northern edge of this Souss Basin is bounded by the 150-km-long South Atlas Front. This WSW–ENE-striking fault zone is structurally formed from west to east by four main fault tracts (Fig. 3): the WNW-striking, 30-km-long Agadir–Tagragra fault tract, the WSW-striking, 35-km-long Oued Issen fault tract, the east–west-striking, 45-km-long North Taroudannt fault tract, and the east–west to WNW-striking, 40-km-long Oulad Berrhil fault tract. These four fault tracts provide a kind of first-order structural segmentation and displays different structural characteristics. The westernmost tract corresponds to a blind fault, where thin-skinned propagation of the South Atlas front is associated with anticline ranges at the surface. In striking contrast, the Oued Issen tract is a relatively linear fault that should correspond to an emerging ramp of the main South Atlas Front, partly hidden by upstream parts of alluvial fans. The North Taroudannt tract is a 10-km-wide thick-skinned propagation within the Palaeozoic of the South Atlas Front displaying ENE-striking folds that are oblique to the main fault strike. Conversely, the Oulad Berrhil tract is the easternmost propagation of the Oued Issen fault zone to the east of the North Taroudannt fault propagation. In fact, structural data, satellite imagery, and fieldwork showed that these four main fault tracts are made of segments that are approximately 15-km long.
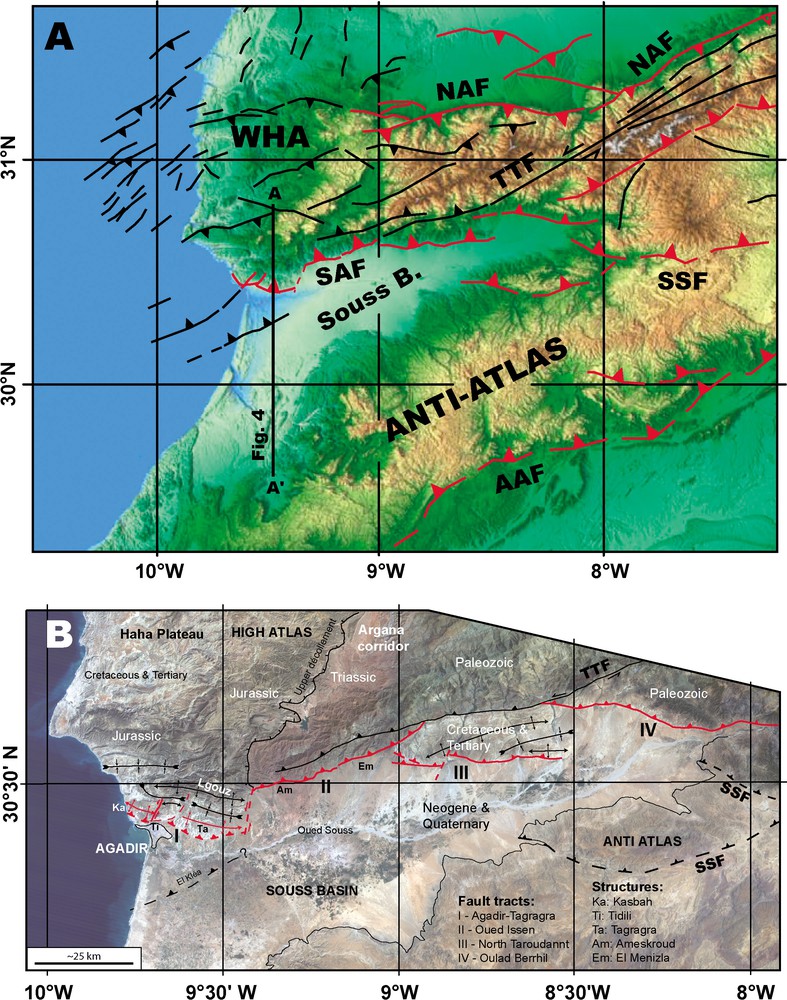
Structural style of the southern High Atlas Front in the Souss Basin. A: Neotectonic sketch map, active faults are mapped with red heavy lines (see Fig. 2). Background image: SRTM90 digital topography. B: Map of the studied structures overlain on a LANDSAT TM image extract.
Style structural du front sud-atlasique dans le bassin du Souss. A : Carte néotectonique schématique, les failles actives sont cartographiées en lignes continues épaisses rouges (voir Fig. 2). Fond topographique numérique : données SRTM (voir Fig. 1). B : Carte des structures étudiées drapées sur un extrait d'image LANDSAT TM.
Careful observations of surface structural data permit generally to determine similar segment lengths along the border faults on both sides of the High Atlas (Fig. 3). Considering that one of these 15-km-long fault segments has the ability to rupture entirely during an earthquake, maximum potential magnitudes may be estimated. Indeed, using statistical correlations [38] or assuming realistic estimates for fault seismogenic depths (i.e., between 8 and 15 km; Fig. 4) and coseismic displacements (i.e., 0.3 to 0.5 m) for calculating moment magnitudes yield Mw between 6.1 and 6.4 for ∼15-km-long faults. In comparison, the 1960 Agadir earthquake is characterized by and whatever the fault source of this destructive earthquake, either the lateral Tildi Fault or the frontal ramp fault of the Kasbah Anticline, this smaller magnitude indicates a smaller fault area, which agrees both with the determined shallow seismic focus [5,9,17] and a shorter fault length that makes sense with the 8-km-long Kasbah Anticline. Consequently, the 1960 Agadir earthquake ruptured only the superficial part of the South Atlas fault zone, such shallow seismogenic location may be explained by the thicker sedimentary pile of the westernmost Souss Basin, so that the thin-skinned deformation has the ability to produce destructive earthquake. Thus, from the seismic hazard perspective, the Agadir area appears exceptional with respect to the remaining High Atlas domain, where thin-skinned deformations have no significant seismogenic potential. We performed detail analyses on two different cases: the Ameskroud Fault, which is an emerging ramp of the South Atlas Fault, and the Tagragra Anticline, which is an actively growing anticline associated to a thin-skinned propagation of this South Atlas Fault Zone. We give below the main obtained results on these two areas; detailed results will be published in other papers.
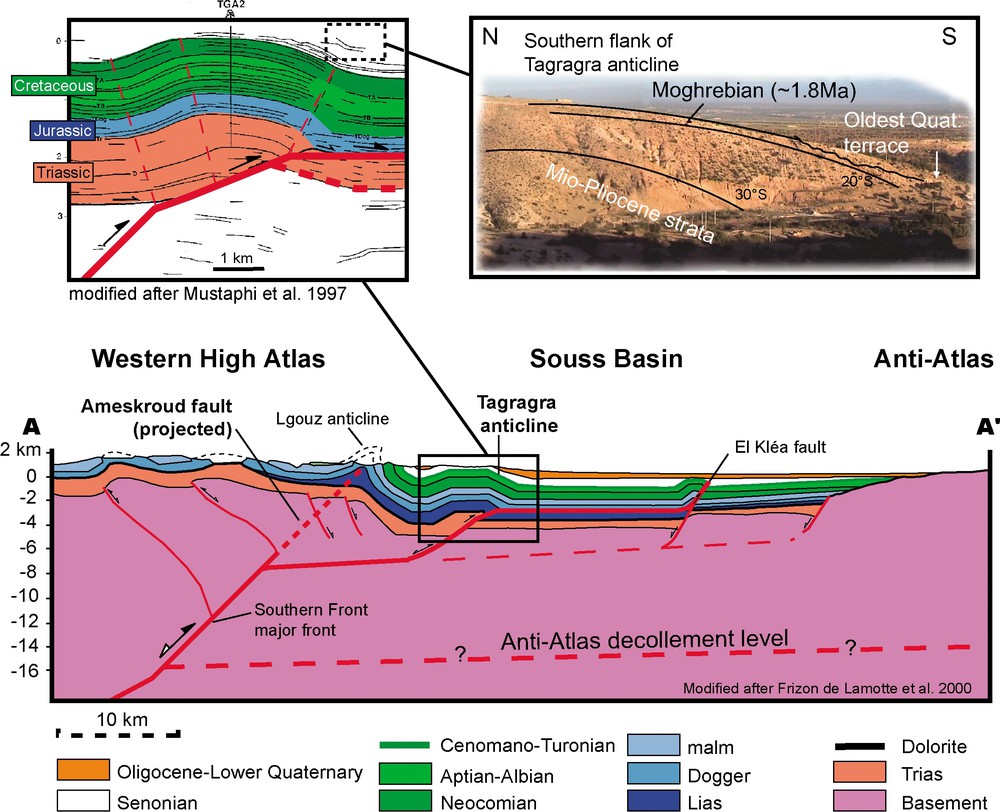
Crustal scale cross-section modified after [14]. Interpreted seismic line across the Tagragra Anticline is after [26]. The major former normal fault rooted at depth propagated upward thanks to two different geometries: (1) direct upward propagation to the surface yielding to the emergence of the fault at Ameskroud; (2) upward propagation on a décollement level at about 6–7-km depth, where the former normal faults may have rooted during the extensional period, and ramping off to a shallow décollement level up to the Tagragra fault bend fold. The panoramic view of the Tagragra southern flank along the El Arbaa River bed illustrates how the Plio-Quaternary Morghrebian surface, as well as the Late Quaternary deposits are also involved in the deformation.
Coupe à l'échelle crustale modifiée d'après [14]. Interprétation de ligne sismique à travers l'anticlinal de Tagragra [26]. Les anciennes failles normales majeures, enracinées en profondeur, se sont propagées vers la surface grâce à deux types de géométrie : (1) propagation directe de rampe vers la surface conduisant à l'émergence de la faille à Ameskroud ; (2) propagation sur un décollement de couverture vers 6–7 km de profondeur, sur lequel les anciennes failles normales devaient s'enraciner durant la période d'extension, puis propagation sur rampe et décollement superficiel qui produit le pli de rampe par cintrage de Tagragra. Vue panoramique du flanc sud de l'anticlinal de Tagragra le long du ruisseau El Arbaa, montrant que la surface Pliocène marine moghrébienne et les dépôts quaternaires sont affectés par le plissement.
4.1 The Ameskroud Fault
The WSW–ENE-striking Ameskroud Fault belongs to the westernmost extension of the Oued Issen fault tract; this is made of two segments: the 20-km-long El Menizla Fault to the east and the 15-km-long Ameskroud Fault to the west. The Ameskroud Fault separates the Triassic red beds of the Argana Corridor, to the north, from the Quaternary alluvial fans of the northern Souss basin and may be interpreted as an emerging ramp of the South Atlas Fault zone. This fault is relatively rectilinear at map scale; careful observations reveal it corresponds to a reverse fault associated with a south-dipping monoclinal fold, which is frequently hidden below the apexes of alluvial fans, so that it crops out at few localities. This is the case to the east of the Ameskroud village, where the incision of the Ameskroud River permits to analyse the Ameskroud Fault in detail (Fig. 5). The most conspicuous evidence of its tectonic activity is provided by the dramatic change of the Quaternary alluvial fans across the Ameskroud Fault (Fig. 5C). Indeed, the northern hangingwall is characterized by stepped strath terraces, which are about 2-m thick and lying onto the Triassic red beds, while the southern footwall displays only slightly inset alluvial terraces that are nearly merging southward. This arrangement shows thus that erosion has been present to the north, i.e., in the hangingwall block, while alluvial aggradation has been predominating to the south, i.e., in the footwall block. This demonstrates that the northern block is uplifting, while the southern one is subsiding and that consequently, the Ameskroud Fault has been slipping during the emplacement of the Quaternary alluvial fans of the northern Souss Basin.
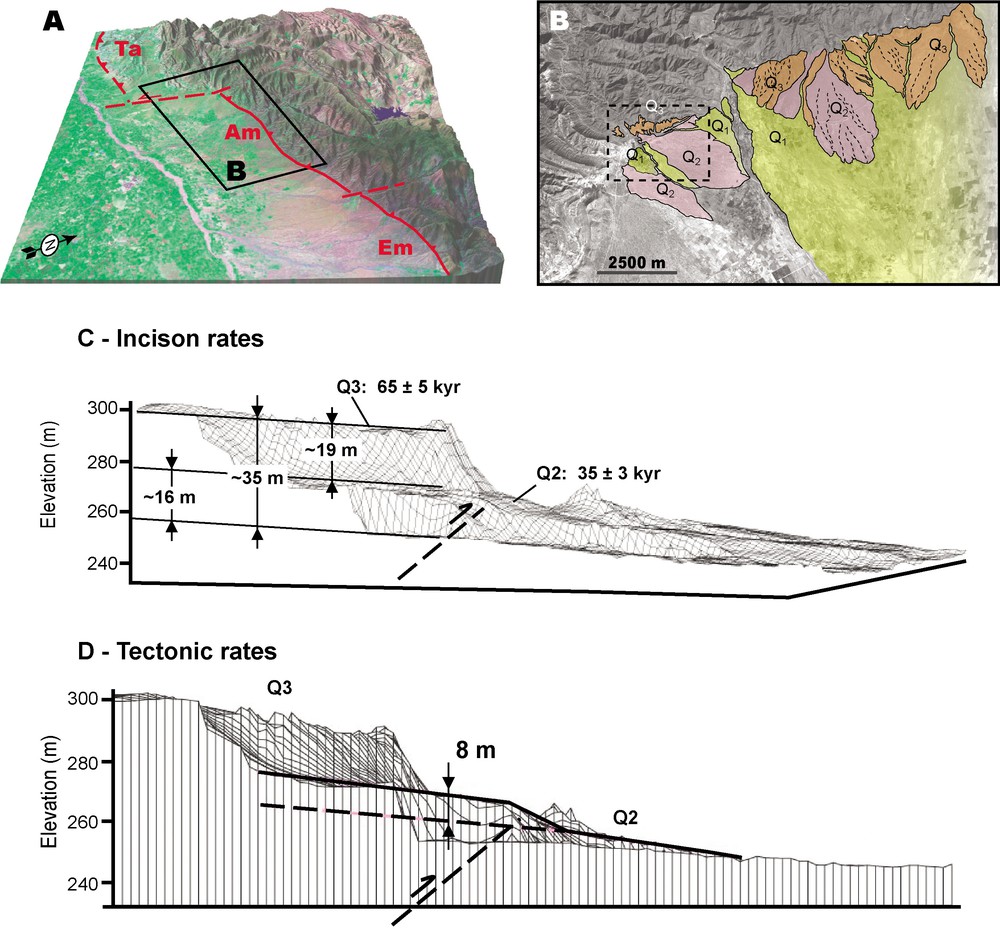
Geomorphic setting of the Ameskroud Fault. A: LANDSAT TM draped over SRTM90 digital topography locating the studied area along the Ameskroud Fault. B: Map of the Quaternary alluvial deposits overlain onto a SPOT image extract centred on the Ameskroud area. C: Quantification of the vertical river incision with respect to the alluvial terraces using the digital elevation model derived from kinematic GPS topographic data. Kyr numbers refer to cosmic ray exposures ages of the alluvial terraces. D: Quantification of the vertical tectonic displacement using the digital elevation model derived from kinematic GPS data.
Cadre géomorphologique de la faille d'Ameskroud. A : Image LANDSAT TM drapée sur la topographie numérique SRTM90 (voir Fig. 1) et situant la zone étudiée le long de la faille d'Ameskroud. B : Carte des dépôts alluviaux quaternaires, drapés sur un extrait d'image SPOT centré sur la zone d'Ameskroud. C : Quantification de l'incision fluviale verticale par rapport aux terrasses alluviales en utilisant un modèle numérique de terrain obtenu à partir de mesures topographiques par GPS cinématique.
As the detailed analyses and results of this locality are published in another paper [34], we just provide the main conclusions. If Quaternary tectonic activity is well demonstrated, there is no absolute evidence of very recent activity, as the younger, possibly Holocene, alluvial terrace appears neither faulted nor even warped. However, several river profiles exhibit a knick point where they are crossing the fault. Detail DEM obtained with differential-GPS measurements permitted to estimate terrace offset. These observations combined with cosmic ray exposure ages on the material of these alluvial terraces permitted to estimate a slip rate on the Ameskroud Fault in the order of 0.4 mm yr−1 for the last 35 kyr (Fig. 5). Although neither the percentage of coseismic slip nor the seismic behaviour of the Ameskroud Fault are documented, one may hypothesize the most unfavourable case where the whole slip rate is seismically accommodated by maximum potential magnitude earthquakes, i.e., events. With those extreme assumptions, such events would occur approximately every 1500 yr. Obviously, other assumptions on the percentage of aseismic deformation and occurrence of smaller events, which might probably be more realistic (but highly speculative!), would increase the average time interval for destructive event.
4.2 The Tagragra anticline
The WNW–ESE-striking, 15-km long, Tagragra Anticline is located just to the ENE of the Agadir city. According to sub-surface data the Tagragra Anticline is a fault-bend fold [26] that is controlled by a thin-skinned southward propagation of the South Atlas front to the east of Agadir city and to the southwest of the Ameskroud Fault (see previous section). These data also suggest that the Tagragra Anticline is the major recent or active structure able to accommodate displacement on the South Atlas Fault Zone between Ameskroud and Agadir. Its outcropping core is made of Cretaceous marly limestones and well data showed its subsurface core consists in Triassic. Its southern 70°-to-30°-dipping limb exhibits a Cenozoic series containing, from bottom to top, Oligocene (?) conglomerates, Miocene–Pliocene silty and gravely sands, Moghrebian (Upper Pliocene to Lowermost Quaternary) bioclastic limestones, and Quaternary conglomerates (Fig. 4). Those Quaternary conglomerates are highly cemented by calcrete process and pass laterally to the highest alluvial terrace of the northern bank of the Souss River. Thus, the Tagragra Anticline is clearly a Quaternary fold and we selected it to analyze the geological and geomorphological evidence of recent activity in slow deforming conditions.
Working on the detailed topography of the alluvial terraces located on the southern limb of the Tagragra Anticline, we detected both southward terrace warping and northward increase of drainage incision, as well as folding of alluvial deposits to the east of this anticline (Fig. 6C). Our results show thus that this structure is actively growing during the Quaternary both southward (frontal propagation) and eastward (lateral propagation). The surface envelope of the Moghrebian abrasion surface allowed us to estimate the amount of shortening (Fig. 6B), since the sedimentation of the Moghrebian limestones, i.e., since about 2–2.5 Myr, and consequently to calculate a slip rate in the order of 0.3 mm yr−1 for the blind fault that controlled the folding of the Tagragra Anticline. Taking into account the error bars, this slip rate is then close to the one determined for the Ameskroud Fault (see previous section). It is also similar to the 0.1–0.2 mm yr−1 uplift rate calculated for the Kasbah Anticline on the basis of dated, stepped, Quaternary marine terraces [24]. Therefore, the Quaternary, and probably active, strain rate of the South Atlas Fault Zone in the western Souss Basin is of some tenths of millimetres per year.
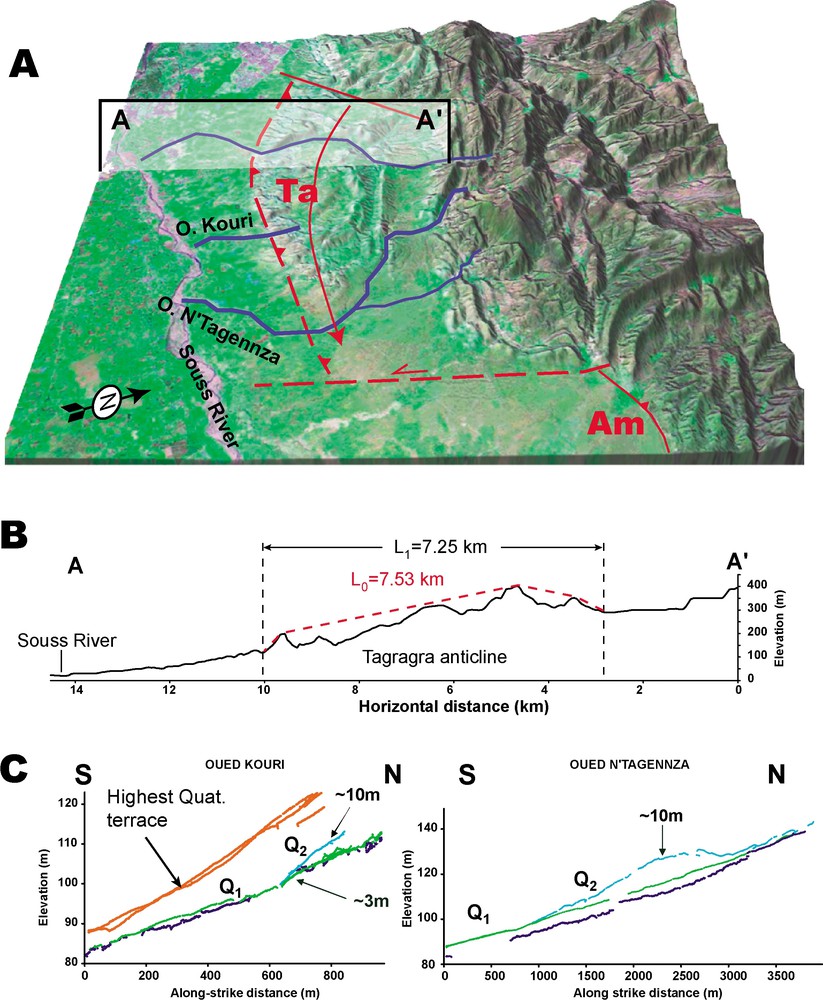
Geomorphic setting of the Tagragra Anticline. A: LANDSAT TM draped over SRTM90 digital topography showing the fold morphology and locating the river beds used to map the Quaternary terraces (thick blue lines). B: Estimation of the total horizontal shortening recorded by the folded Moghrebian surface. C: Plots of the vertical distribution of the alluvial Quaternary deposits along the Kouri and N'Tagennza temporary riverbeds from kinematic GPS survey.
Cadre géomorphologique de l'anticlinal de Tagragra. A : Image LANDSAT TM drapée sur la topographie numérique SRTM90 (voir Fig. 1) montrant la morphologie du pli et situant les lits de rivière utilisés pour carter les terrasses alluviales quaternaires (lignes épaisses blues). B : Estimation du raccourcissement total enregistré par la surface moghrébienne plissée. C : Représentation de la distribution verticale des dépôts alluviaux quaternaires le long des oueds Kouri et N'Tagennza, d'après les mesures topographiques par GPS cinématique.
5 The Ouarzazate Basin
The WSW–ENE-striking Ouarzazate Basin is the easternmost basinal depression that is located between the High Atlas and the Anti Atlas, locally known as Jbel Sarhro (Fig. 7). It extends on roughly 180 km from the eastern side of the Siroua Plateau to the outlet of the Todra canyon (5°30′W), with a maximum width of some 30 km and altitudes ranging from 1150 m, at Ouarzazate, to 1600 m on its northern edge at the contact with the High Atlas. This Ouarzazate Basin displays a rather incised and strongly dissymmetric topography, its main drain, the Dades River, is localised along its southern border at the contact with the Jbel Sarhro due to well-developed alluvial aggradation, which results from the strong erosion of the High Atlas. Similarly to the Souss Basin, it is characterized by a steep northern border along the High Atlas and a more gentle, north-dipping basement slope along the Anti Atlas. This basement slope exhibits conspicuous onlap geometry of the sedimentary deposits from Eocene to Miocene suggesting an actively flexural Cenozoic process related to basin development. Presently, this foreland subsidence appears no longer active, as the Ouarzazate Basin is dominated by erosion and sedimentation is only restricted to the thin aggradation of south-dipping alluvial fans.
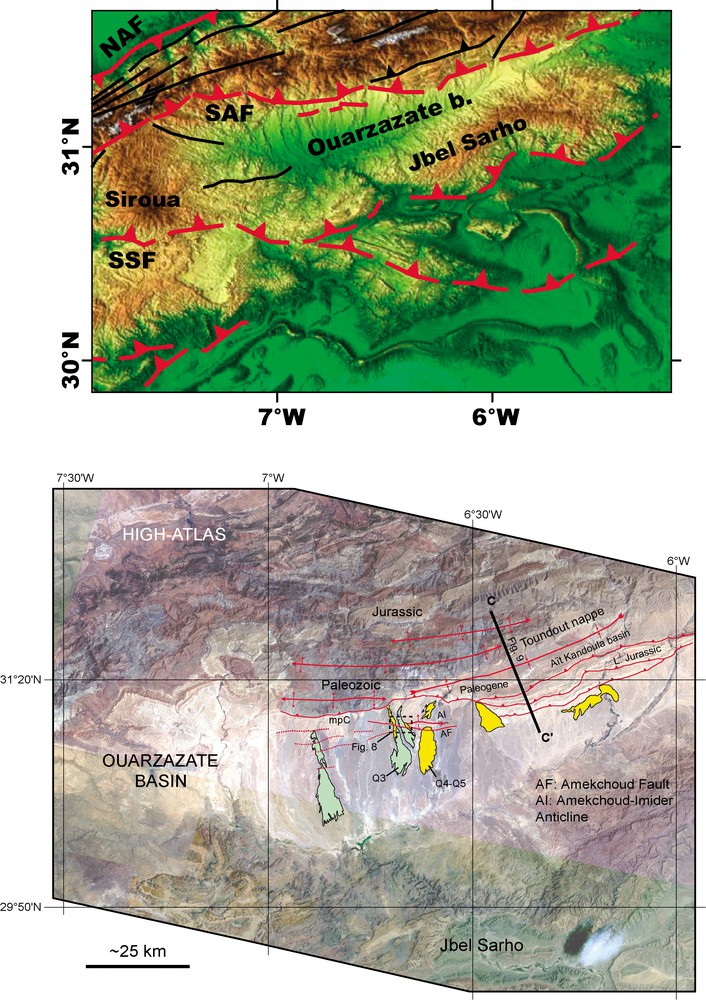
Structural style of the South Atlas Front in the Ouarzazate Basin. A: Neotectonic sketch map of the Ouarzazate Basin. Active faults are mapped with red heavy lines. Background image SRTM90 digital topography. B: Map of the analyzed structures together with the Quaternary alluvial units that are affected by cumulative deformation overlain on a LANDSAT TM image extract.
Style structural du front sud-atlasique dans le bassin de Ouarzazate. A : Carte néotectonique schématique du bassin de Ouarzazate. Les failles actives sont cartographiées avec des lignes épaisses rouges. Fond topographique numérique SRTM90 (voir Fig. 1). B : Carte des structures analysées avec les unités alluviales quaternaires qui sont affectées par la déformation cumulée et drapées sur un extrait d'image LANDSAT TM.
The northern edge of the Ouarzazate Basin is bounded by the South Atlas Fault Zone, this one displays a relatively linear WSW–ENE strike; however, it becomes more NE–SW to the west of the Ouarzazate Basin along the northern boundary of the Siroua Plateau. As in the Souss Basin, this South Atlas Fault Zone corresponds overall to a south-vergent reverse fault associated with a south-facing monoclinal fold. However, it can be locally much more complicated as gravitational nappes and related imbricated thrusts are observed [12,14]. Due to these structural complications, we did not pay much attention to define the segmentation of the South Atlas Fault Zone along the Ouarzazate Basin. Our main objectives were to analyze active tectonics within climatic conditions that are more desertic than that of the Souss Basin. For this purpose we concentrated our efforts on the South Atlas Fault Zone between approximately 6°30′W and 7°W, where the fault zone is relatively simple and displays southward progradation structures, which deforms Quaternary alluvial fans.
Our analyses were focused on the 17-km-long Amekchoud Fault. This fault is in fact a faulted ramp anticline, which is cored by reddish Miocene continental beds. The cumulative slip on this fault produced scarps on the flat-topped surfaces of the Quaternary alluvial fans that are overlying the Miocene red beds (Fig. 8). Scarp heights increase with the relative ages of the affected alluvial fans, indicating a continuous Quaternary activity on the fault. However, here also the younger fan terraces, possibly Holocene, do not display any clear evidence of faulting activity. To try to estimate the slip rate on the Amekchoud Fault, we constructed both a balanced cross section of the Miocene beds (Fig. 9) and a differential GPS-based DEM of the alluvial fan surfaces (Fig. 8).
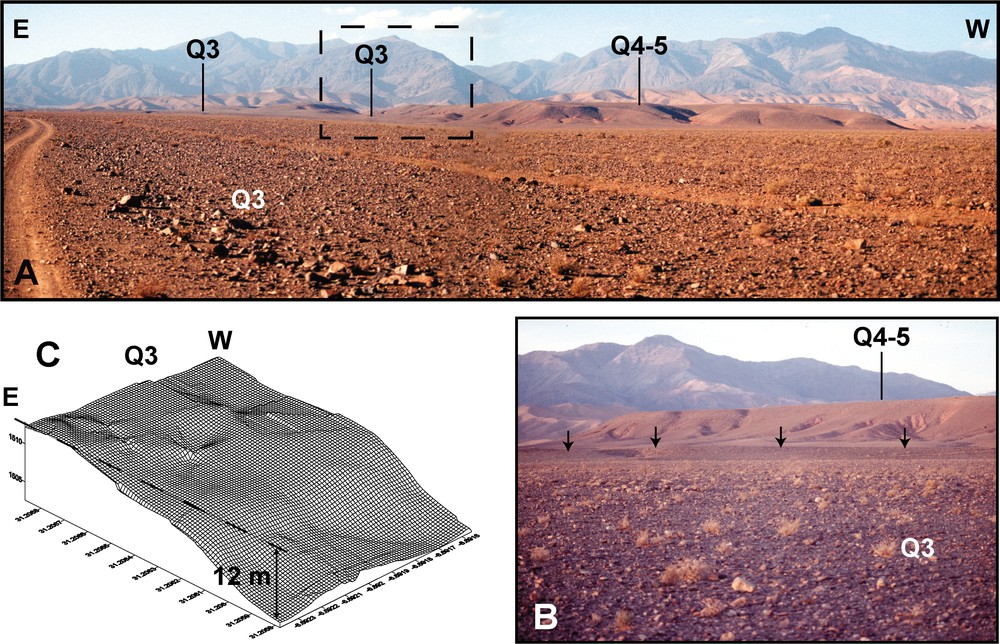
A: East–west panoramic view of the landscape at Amekchoud–Imider, showing the cumulated escarpment on Q4–5 and Q3 Quaternary alluvial levels. B: Close-up view showing the cumulative scarp recorded by the Q3 alluvial level. C: Site DEM, derived from kinematic GPS data, of the Q3 alluvial level showing a vertical offset of about 12 m.
A : Vue panoramique est–ouest du paysage à Amekchoud–Imider, montrant l'escarpement cumulé dans les niveaux alluviaux quaternaires Q4–5 et Q3. B : Vue de détail montrant l'escarpement (scarp) cumulé affectant le niveau alluvial Q3. C : Modèle numérique de terrain local, construit à partir des mesures de GPS cinématique, du niveau alluvial Q3, montrant un rejet vertical d'environ 12 m.
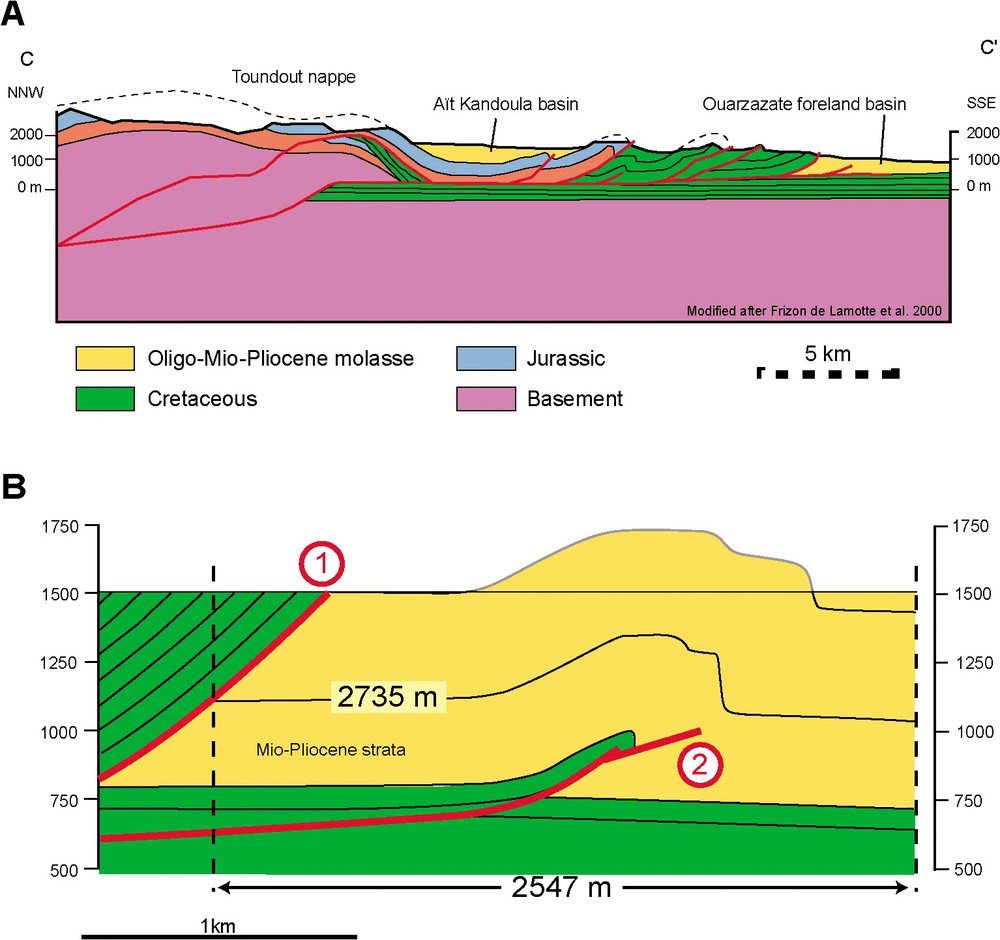
A: Cross-section through the southern part of the Central High Atlas (modified after [14]). B: Balanced cross-section of the Amekchoud–Imider Anticline, showing that 190–200 m of shortening have been accommodated by this structure.
A : Coupe à travers la partie méridionale du Haut Atlas central (modifiée d'après [14]). B : Coupe équilibrée à travers l'anticlinal d'Amekchoud–Imider, montrant que 190–200 m de raccourcissement ont été accommodés par cette structure.
The balanced section yields some 190–200 m of shortening, while the DEM permits to calculate 12 m of vertical offset for a Q3 alluvial fan terrace along the Imider River. As the Miocene beds contain Uppermost Miocene fossils [12], we estimated that the obtained shortening was realized in a maximum time-span that covers Pliocene and Quaternary, i.e., during the last 5 Myr, giving a minimum shortening rate of ∼0.04 mm yr−1 and consequently, on a 45° north-dipping fault, the resulting long-term slip rate on the Amekchoud Fault should have a lower bound in the order of 0.05–0.06 mm yr−1. An upper bound slip rate can be estimated to be in the order of 0.15 mm yr−1, considering that folding started by the end of Pliocene, i.e., by 2 Ma, as a younger choice would be very unrealistic because the Quaternary alluvial fans appear much less folded than the Neogene red beds. Thus long-term slip rate on the Amekchoud Fault should be bracketed between 0.05 and 0.15 mm yr−1. As the Q3 dating is still under process, any slip-rate estimate remains very speculative. Nonetheless, two reasonable age choices may be tested for the Q3 fan terrace at Imider: either we consider the same 65 ka as the one determined at Ameskroud in the Souss Basin, or we consider that the well-developed Q2 fan terrace is coeval with the last interglacial, i.e., , then the Q3 should correspond roughly to the well-developed penultimate one, i.e., roughly 300 ka. The 12 m of vertical offset for Q3 would consequently end up with a Quaternary slip rate on the order of either 0.26 or 0.06 mm yr−1, respectively. Obviously, this last estimate is highly speculative and will have to be clarified thanks to CRE dating of the Q3 level. Therefore, taken all together, these estimates suggest that the slip rate on the Amekchoud Fault should be very roughly in the order of 0.1 mm yr−1. However, comparing the strongly eroded Amekchoud–Imider Anticline with the relatively well-preserved Tagragra one of the Souss Basin (see previous section) does not favour similar slip rate for their associated faults, more especially because the erosion rates are probably higher in the western Souss Basin than in the Ouarzazate one. Consequently, the lower bound estimates, which are below 0.1 mm yr−1, are more likely than the upper bound ones.
Estimating a potential magnitude for the Amekchoud Fault proves even more complicated than estimating its slip rate. Indeed, the balanced cross section favours a very shallow décollement level, probably lower than 1 km (Fig. 9). Then, the thin-skinned Amekchoud Fault should have no seismic potential to produce damaging earthquake. Nonetheless, this fault should be rooting, some 10 km northward, on the major thick-skinned, boundary fault of the High Atlas, i.e., the South Atlas Fault Zone. The length of the basement anticline, which is located to the north of the boundary fault, is in the order of 40 km, suggesting that this may be the actual length of the hidden thick-skinned ramp at depth. If so, this ramp would have the potential to produce earthquake. Therefore, two extreme cases may be considered: (1) the Amekchoud Fault has no seismic potential and the observed deformation is mostly aseismic or (2) this fault is the surface partial expression of a major hidden ramp fault that has the potential for earthquakes. Whatever the truth, there should be a major hidden fault at depth, with an unknown segmentation. Further study should be performed to determine to which extent the analysis of the Amekchoud Fault may provide us with information on a major hidden ramp fault. In other words, what rupture percent of the major fault is transmitted to the thin-skinned deformation that may be analysed at the surface? Is the geometry of this thin-skinned deformation indicative of the segmentation of the deep hidden fault, i.e., would the maximum potential earthquake produce a 15- () or 40- () km-long rupture?
6 Discussion and conclusions, potential maximum Mw, and deformation rates
Continuing Quaternary activity along the South Atlas Fault Zone is demonstrated at several sites on fault segments that are characterized by lengths of about 15 km. These typical sizes allow estimating maximum potential magnitudes, Mw, ranging between 6.1 and 6.4, while associated fault slip rates are estimated to be in the order of ca 0.1 mm yr−1. Until now, there are no available data on these fault segments that would permit to document their seismic behaviour; in particular, the percentage of active strain that might be accommodated by creeping slip is unknown, so that the actual magnitudes and return periods for destructive earthquakes are not accurately determined and then, only upper bound values may be estimated.
Although continuing Quaternary activity is well documented along the South Atlas Fault Zone, we did not observe any clear evidence showing that the youngest terrace, Q1, (Holocene?) might be faulted or even warped. Data on instrumental seismicity show obviously that compressional deformation is still going on along this fault zone, as in the remaining Atlas Mountains. This apparent lack of Holocene deformation might result from a non-detailed survey of the entire fault zone; nevertheless, at several sites where Quaternary activity is observed, no Holocene one can be demonstrated. Then, the low estimated fault slip rates appear more likely to explain such a lack of Holocene deformation; indeed, a slip rate of 0.1 mm yr−1 will cumulate 1 m of displacement during the Holocene and produce a maximum of 2 to 3 earthquakes with . Taking into account that reverse slip ruptures do not usually propagate entirely toward the surface, a couple of meters of displacement during a time-span of 10 kyr is probably close to the deformation threshold that can be detected directly (faulting) or indirectly (warping) within alluvial deposits. Moreover, this cumulated deformation will be even more difficult to determine on blind fault segments. Therefore, to detect evidence of activity on slow active faults, which are located in compressional tectonic domains as the Atlas region, the observation time-window must cover the whole Quaternary.
Is the South Atlas Fault Zone the southernmost front of active deformation? If so, why seismic activity is observed further south in the Anti Atlas? Does this seismicity correspond to the southernmost propagation of compressional deformation or to platform deformation? The southernmost unarguable evidence of Neogene compressional deformation is provided by the South Siroua Fault (Fig. 3) where Upper Miocene and Pliocene volcanic to volcanoclastic formations are seen faulted. Further south, along the southern slope of the Anti Atlas, some monocline folds as the Tata flexure, which are affecting Precambrian and Palaeozoic rocks, have a topographical signature that suggests they have had Cenozoic tectonic activity. However, we did not observe any Cenozoic deposits affected by such structures. Therefore, additional data are necessary to address the above questions, as in the other part of the Atlas Mountains these should focus on accurate seismicity studies and quantitative geomorphology analyses using the new SRTM topographical data.
Finally, from the geodynamic perspective, the rough NW–SE trend featured by the regional distribution of Atlas epicentres (see Section 2 and Fig. 2) appears localized above the oval-shaped asthenospheric upwelling that is modelled below the Atlas Mountains [25]. Thus, the lithospheric location of active deformation, as imaged by seismicity, seems controlled by the peculiar rheology resulting from the weakening of the Atlas lithosphere by this mantle upwelling. However, the occurrence of intermediate seismicity below the Atlas [28] is problematic in such a geodynamic setting. Indeed, lithospheric delamination [32] is unlikely as the small amount of Atlas shortening could not form a significant cold lithospheric root. Consequently, additional seismological studies are needed to determine what process triggers this intermediate seismicity. Does it correspond to deep lithospheric brittle necking of a still strong lithospheric mantle? Or to thermally-driven phase transition in the asthenospheric upwelling mantle?
Acknowledgements
This work has been realized within the SAFE (Slow Active Faults in Europe) European project (EVG1-2000-22005) and thanks to the field support of CNR Rabat. We thank Prof. Azelrab El Mouraouah and Pr Aomar Ibenbrahim for their help and fruitful discussions about the Moroccan seismicity.