1 Objectives and previous data: borehole HTM 102
Biostratigraphical, sedimentological, and mineralogical studies performed on the ANDRA (French nuclear waste disposal agency) HTM 102 borehole throw light on the origin of clay minerals and on the evolution of clay assemblages (Fig. 1) [42]. The mineralogy of the bulk rock and the clay fraction was studied using X-ray diffraction. Samples were taken from the cores at ∼0.5-m spacing throughout the Callovian and Oxfordian argillaceous formations. The determination and relative abundance of the clay minerals were obtained following the procedure described by Moore and Reynolds [39]. The relative abundance of clay minerals is estimated at from the area of the main diffraction peaks [4,39,46] using the MacDiff software [44].
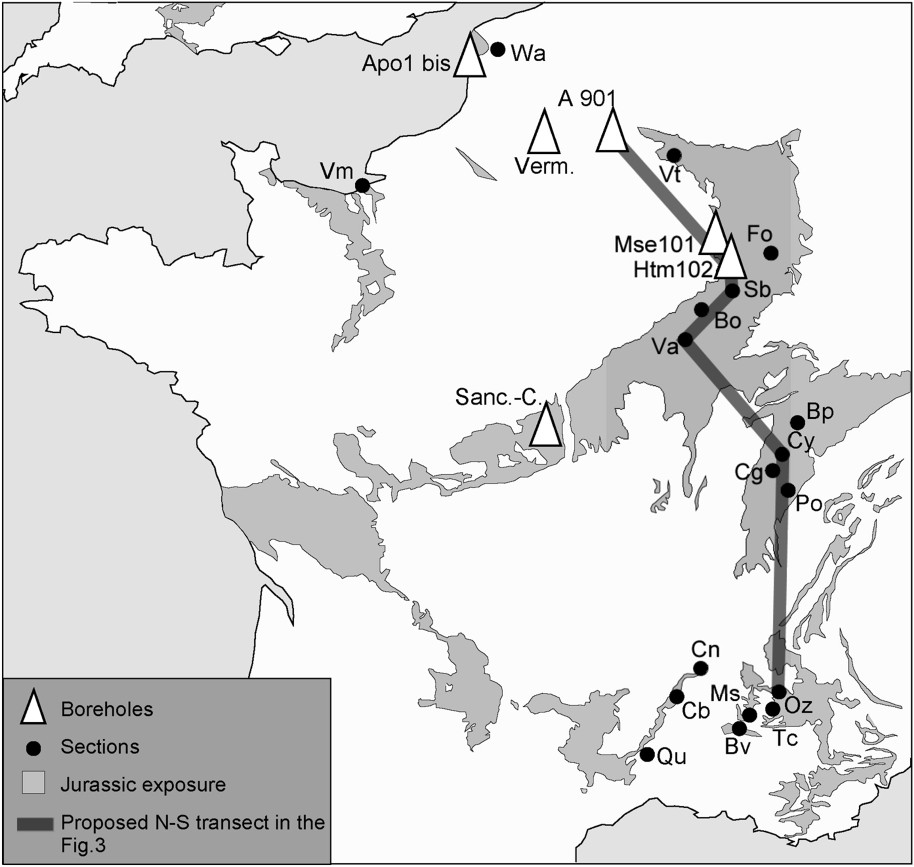
Location of the studied boreholes and outcrops location. Vm: Villers-sur-Mer, Wa: Le Wast, Verm.: Vermandovilliers, Vt: Villers-le-Tourneur, Fo: Foug, Sb: Saint-Blin-Semilly, Bo: Bologne, Va: Veuxhaulles-sur-Aube, Sanc.-C: Sancerre-Couy, Bp: Bonnevaux-le-Prieuré, Cy: Crotenay, Cg: Creux-du-Giron, Po: Le Pontet, Oz: Oze, Tc: Trescléoux, Bv: Beauvoisin, Ms: Montréal-les-Sources, Cn: Le Chenier, Cb: Chanabier, Qu: Quissac, A901, MSE 101, HTM 102: ANDRA boreholes, Apo1 bis: borehole near Boulogne-sur-Mer.
Localisation des forages et affleurements utilisés dans le cadre de l'étude. Vm : Villers-sur-Mer, Wa : Le Wast, Verm. : Vermandovilliers, Vt : Villers-le-Tourneur, Fo : Foug, Sb : Saint-Blin-Semilly, Bo : Bologne, Va : Veuxhaulles-sur-Aube, Sanc.-C : Sancerre-Couy, Bp : Bonnevaux-le-Prieuré, Cy : Crotenay, Cg : Creux-du-Giron, Po : Le Pontet, Oz : Oze, Tc : Trescléoux, Bv : Beauvoisin, Ms : Montréal-les-Sources, Cn : Le Chenier, Cb : Chanabier, Qu : Quissac, A901, MSE 101, HTM 102 : forages Andra, Apo1 bis : forage à proximité de Boulogne-sur-Mer.
The clay minerals identified include kaolinite, chlorite, illite, random smectite-rich illite–smectite mixed layers (R0, 45 to 75% of smectite layers estimated using the saddle index [31] and here called smectites) and ordered illite–smectite mixed layers (R1, containing 25 to 45% of smectite layers). Two mineralogical units are roughly distinguished by their clay mineralogy; the lower part of the argillaceous formation is dominantly composed of illite and kaolinite, while the upper part is characterised by smectite-rich clay assemblages. A transitional Lower Oxfordian zone (Mariae Zone, Scarburgense subzone) separates the two units.
In detail, five zones are distinguished in the HTM 102 borehole following their clay mineralogy (Fig. 2):
- (1) the first one, characterised by abundant kaolinite (45 to 50%) and illite (25–30%), coincides with the Lower Callovian oobioclastic limestones (Calloviense zone);
- (2) from 472.1 to 418.7 m, the sediments show relatively homogeneous clay assemblages composed of illite (35–50%) and kaolinite (15–35%);
- (3) the thin transitional zone (8 m) between 418.23 and 410.1 m corresponds to the major mineralogical change. The proportions of kaolinite and illite decrease abruptly from 30 to 5% and from 50 to 30%, respectively. The decreasing proportions of these minerals are balanced by increasing proportions of illite–smectite mixed layers. Upwards, the main reflection of the mixed layers shifts from 14–16 to 17 Å after ethylene-glycol solvation indicating that R1 mixed layers decrease and are progressively replaced by R0 smectite-rich mixed layers. This major observation shows that the mineralogical change had a detrital rather than a diagenetic origin. The following thermometric data measured on organic compounds [35], and the isotopic studies performed on the clays [49] have confirmed the low diagenetic influence on clay minerals, which cannot therefore be held responsible for the clay mineralogical evolution of the argillaceous formation;
- (4) a homogeneous zone from 409.63 to 373.43 m, with high proportions of I–S R0 (60–80%) shows weak fluctuations;
- (5) the uppermost part shows abrupt fluctuations of the relative proportions of illite and illite–smectite mixed layers.
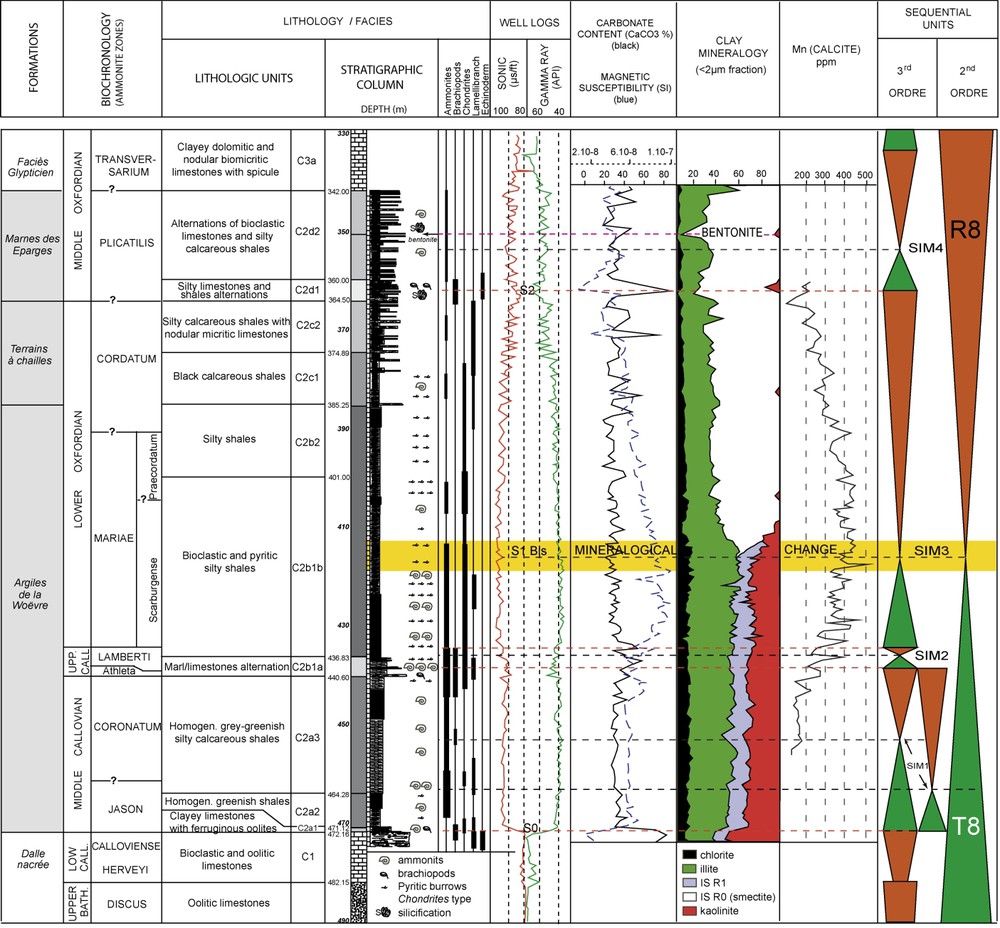
Biostratigraphical and lithological data, facies description, sonic and γ-ray data, mineralogical and geochemical data, sequential interpretation from ANDRA HTM 102 borehole. Lithology, sonic and gamma-ray data are mainly from ANDRA [2]. Manganese data on calcite from [3].
Données biostratigraphiques, litho-faciologiques, diagraphiques, minéralogiques, géochimiques et séquentielles du sondage Andra HTM 102. Lithologie et diagraphies en partie issues de données Andra. Données de manganèse sur phase carbonatée issues de [3].
A single horizon, composed of almost pure smectite (K-rich, AlFe dioctahedral beidellites), occurs at 351.42 m. Detailed petrographic and geochemical investigations have shown that it corresponds to a pyroclastic horizon (bentonite) containing volcanic ash transformed into smectite during early diagenetic processes. The occurrence of this horizon reveals the influence of an explosive volcanism described for the first time in French sedimentary basins [42].
The major mineralogical changes within the Callovian and Oxfordian sediments, identified by preliminary studies of the HTM 102 borehole, imposed further study of the lateral evolution of clay minerals around the ANDRA site, in the entire Paris Basin, and in the French Subalpine Basin, where these argillaceous formations are particularly well exposed. Lateral studies would also provide more detailed information about the bentonite layer, thus helping to identify active volcanic centres.
Several additional sections and boreholes were therefore studied in the East of the Paris Basin (from the Burgundy carbonate platform to the Lorraine platform), in the North (Boulonnais, and Ardennes platform), and in the West (close to the Armorican Massif), including for this final section other studies [17,18]. Sections from the Jura Mountains and the Southeast of the Massif Central were studied, including those considered within the frame of the ‘Oxfordian transect’ from the ‘French Group for Jurassic study’ [24,25]. Subalpine Basin sections were studied in the Diois area, the Baronnies, and the Serres–Laragne zone.
The comparison of Callovian to Oxfordian clay assemblages deposited in various environments ranging from inner platform to distal outer environments, allows the origin of terrigenous fluxes to be studied and the relative influence of eustatism, climate and tectonics to be deciphered.
Sedimentary marker beds (bentonites), preserved in the Callovian/Oxfordian interval, indicating explosive volcanism, were studied thoroughly [43], as explosive palaeovolcanism was previously unknown in Europe and on Tethyan borders. Bentonites, which are instantaneously deposited over wide areas, allow section correlation over long distances. Within the biostratigraphical framework based on ammonite fauna [52], bentonites allow inter-basin correlations with the highest possible resolution. The occurrence of preserved pyroclastic minerals (including apatites, biotite and K-feldspar) allows calculation of radiometric age. Finally, the authigenic origin of clays (mainly smectites) derived from the weathering of volcanic ash gives some information on the diagenetic overprint of the whole sedimentary series. Various stratigraphic, palaeovolcanic and diagenetic applications are discussed in this paper.
2 New data on the distribution of clay minerals in the Callovian/Oxfordian deposits
2.1 Paris Basin
The ANDRA boreholes provide access to new information that could not otherwise have been obtained, as good quality outcrops of argillaceous formations are rare in the eastern part of the Paris Basin. Sedimentological, mineralogical, geophysical and geochemical analyses performed on the deep boreholes [2] allow a precise interpretation of the sedimentary environments from the Lower Callovian oobioclastic facies (carbonate platform of the Dogger) to the Middle Oxfordian transitional facies (more carbonated), announcing the development of a new carbonate platform [10,20]. A sequence stratigraphic framework is proposed within the homogeneous argillaceous formation based on log data, facies analysis, microfacies, grain-size analyses, carbonate percentages, magnetic susceptibility, mineralogy (bulk rock and clay fraction) and chemical analyses of the carbonates (Fig. 2) [20,41,42].
At a regional scale (ANDRA borehole EST 103/104, HTM/102, located 3 km to the south, and MSE 101, 12 km to the north), the clay assemblages show the same mineralogical evolution within the correlation framework based on well-logging and biostratigraphy (ammonite faunas and the occasional occurrence of brachiopods). The transition from carbonate to clay is synchronous and dated to the Middle Callovian [52]. The major mineralogical change occurring in the Scarburgense Subzone (Lower Oxfordian) can easily be correlated; it seems synchronous, from the available biostratigraphic data provided by the ammonite fauna.
The outcrop of coeval formations located about 20 km to the south close to Saint-Blin-Semilly allows a comparison with the data from the borehole. This section is particularly well dated by abundant ammonites [9]. Despite a reduced sedimentation rate, the sedimentary evolution is the same as in the boreholes (Fig. 3). In particular, the same major clay mineralogical change identified in the Scarburgense Subzone also occurs. The more precise biostratigraphic framework available for this section allows this mineralogical change to be located more precisely at the transition between the Scarburgense and Woodhamense horizons. No diachronism in clay evolution between the boreholes and this section can be detected from the biostratigraphic data.
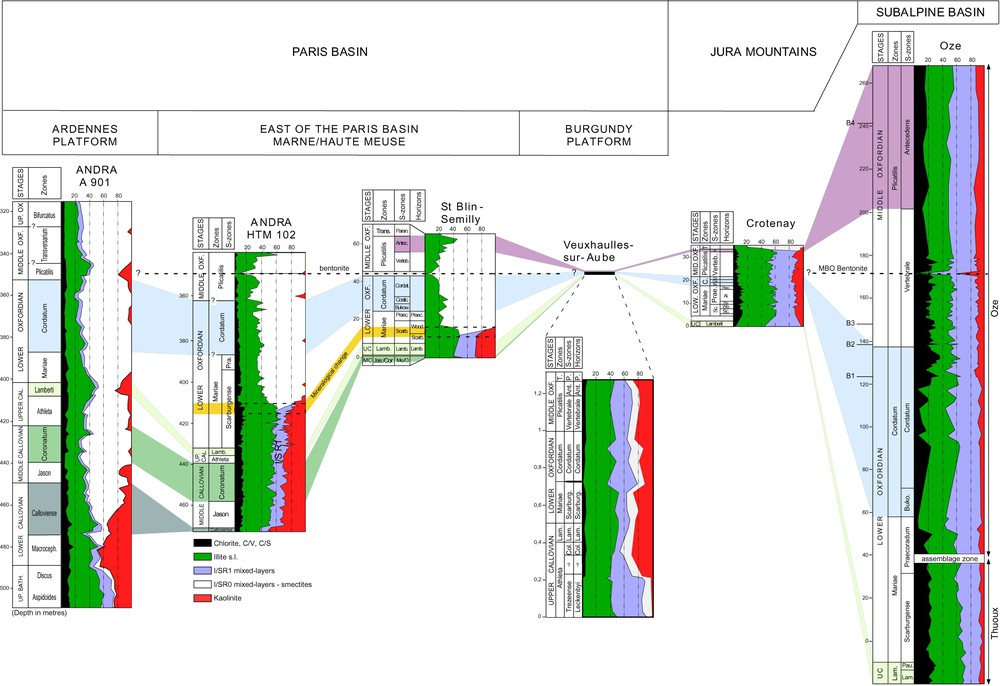
Clay mineralogy data from the Paris Basin, the Jura Mountains and the French Subalpine Basin outcrops and boreholes according to a north–south transect. The bentonite layer from the Paris Basin equivalent to the Mbo layer from the Subalpine Basin is taken as an isochrone.
Évolution des séquences argileuses de coupes et forages du bassin de Paris, du Jura et du bassin subalpin méridional, du Bathonien supérieur à l'Oxfordien moyen, selon une transversale nord–sud (cf. Fig. 1). La bentonite du forage corrélée à la bentonite Mbo du Sud-Est constitue l'isochrone de référence.
In the ANDRA borehole A901 located on the Ardennes border, about 160 km to the north, the clay assemblages are mainly composed of smectites as early as the Macrocephalus zone of the Lower Callovian, while these minerals are absent elsewhere in the Paris Basin. The mineralogical change dated from the Mariae Zone is much less visible along the Ardennes border [13].
2.2 Burgundy platform and Jura Mountains
The highly condensed deposits (Middle Callovian to Middle Oxfordian) of the Burgundy platform are characterised by abundant iron-rich oolites (Fig. 3). At Veuxhaulles-sur-Aube, the well-dated section comprises Fe-oolite bearing marly and calcareous units. Frequent erosional surfaces, evidence of reworking and bored surfaces indicate shoreface to lower offshore environments [8]. The clay assemblages include abundant illite, I–S mixed layers (R1 and R0), and kaolinite. The Athleta zone is characterised by the appearance of kaolinite and small amounts of chlorite. The major mineralogical change occurring in the Mariae Zone, close to the ANDRA site, is not recorded in this section. In addition, the occurrence of kaolinite in the Upper Callovian suggests that this area belonged to a different mineralogical province.
In the external part of the Jura Mountains, the Crotenay section (Fig. 1) shows Upper Callovian (Lamberti Zone) to Middle Oxfordian (Transversarium Zone) sediments, including the upper part of the ‘Calcaires d'Arnans’, the ‘Marnes à Renggeri’, the ‘Couches de passages’ and the ‘couches de Birmensdorf’ [11]. The argillaceous facies with limestone beds of the Lower Oxfordian, and the sponge-rich marls and limestones with Disaster indicate open offshore environments from the outer platform. The clay assemblages similar to those of the Burgundy platform contain more chlorite. A mineralogical change characterised by the disappearance of kaolinite and increasing proportions of smectites occurs in the Antecedens Subzone (Fig. 3).
2.3 Subalpine Basin and Southeast of the Massif Central
From Veynes–Sisteron to the east, and the Diois and the Baronnies to the west, several sections from the Subalpine Basin were studied, mainly to identify ash layers around the Lower Oxfordian/Middle Oxfordian boundary. Because the stratigraphic series is expanded close to the Callovian/Oxfordian boundary (Fig. 3), and in the upper part of the Middle Oxfordian, analyses were performed at less regular intervals. The outcrops did not allow the study of the whole stratigraphic interval from the Upper Callovian to the Middle Oxfordian on the same section. Therefore, a composite section was built from the Thuoux section, for the Callovian/Oxfordian boundary, and from the Oze section, for the Praecordatum to the Plicatilis zones. The relatively homogeneous clay assemblages are composed of iron-rich chlorite, illite, I–S R1, and kaolinite. The bentonites are enriched in kaolinite and sub-regular I–S R1 (Fig. 3).
From the Middle and Upper Oxfordian (Transversarium to Bifurcatus zones), the marly and calcareous series showing the Argovian facies studied on the Trescleoux sections in the eastern part of the Subalpine Basin, and in the Beauvoisin section in the western part, show very similar clay assemblages to those from the underlying ‘Terres noires’ Formation (Fig. 1) [24,25].
On the southeastern border of the Massif Central, the clay mineralogy was studied on three sections: Quissac, Chanabier, and Chenier (Fig. 1) [24–26]. In the Middle Callovian, the clay assemblages in the Quissac section and in the Subalpine Basin Formation are similar. From the Athleta Zone upwards (Upper Callovian), the R1 I–S mixed layers (25% in average) are progressively replaced by R0 I–S; their proportion increases from 30 to 75% up to the Mariae Zone (Lower Oxfordian). The sections of Chenier and Chanabier (Middle and Upper Oxfordian) show the same clay assemblages as sections located in the centre of the basin, but the absence of kaolinite characterises the interval between the Plicatilis and the Parandieri subzones (Transversarium zone).
The Subalpine Basin is therefore characterised by the absence of major mineralogical changes from the Middle Callovian to the Middle Oxfordian, except for the Quissac section, where significant proportions of smectites occur as early as the Upper Callovian.
3 Preservation of volcanic markers
Volcanic ash layers occurring in the sedimentary series are instantaneously deposited from pyroclastic ash fall. In marine environments, volcanic glass is replaced by smectites at the seawater/sediment interface during early diagenetic processes. Therefore, bentonites are composed of thin argillaceous layers displaying distinct mineralogical, geochemical and rheological properties from the enclosing shales [21].
3.1 Bentonites from the Paris Basin
A 12-cm-thick horizon essentially composed of dioctahedral well-crystallized smectites was identified within the Plicatilis ammonite zone (Middle Oxfordian) in the HTM 102 borehole (Fig. 2). The particular mineralogical and geochemical signature, in comparison with the enclosing shales, and the occurrence of volcanic minerals, all show that it corresponds to a volcanic horizon. A search was conducted for the correlation of this horizon, and also for other potential volcanic horizons, in boreholes EST 103, 104 and MSE 101, and equivalent outcrops, but no other horizon was evidenced, either in sections from the East of the Paris Basin (except for the Saint-Blin-Semilly section), or in sections from the North and West of this basin.
The bentonite identified in the Plicatilis Zone of HTM 102 is confidently correlated in the EST 103 and 104 boreholes, and in the main access shaft of the underground laboratory, as well as in the Saint-Blin-Semilly section located 20 km to the south. These correlations are also evidenced by lithology, well logs and biostratigraphy, and by the mineralogical and geochemical characteristics of the bentonite horizon.
Biostratigraphical data from the different boreholes indicate a Middle Oxfordian age (lowermost part of the Plicatilis Zone), compatible with that of the bentonite in the Saint-Blin-Semilly section, identified close to the Cordatum/Plicatilis zones.
The clay fraction of the bentonite comprises 90 to 95% of dioctahedral smectite (K-rich AlFe beidellite) with a cation exchange capacity (CEC) ranging from 30 to 50 Cmol+/kg. The clay fraction also contains 5 to 10% of illite and kaolinite, this latter mineral being absent from the enclosing shales.
The geochemical analyses show a significant enrichment in Nb, Ta, Hf, Y, Ti, Th, Zr, U, and Pb, an enrichment in light Rare Earth elements, and a depletion in Cr, Rb, and Cs by comparison with the enclosing shales and by comparison with standard shales [33].
3.2 Bentonites from the Subalpine Basin
Ten sections from the Subalpine Basin (Oze, Montréal-les-Sources, Savournon, Trescléoux, Sigottier, Ribier, Barsac, Les Sias, Beauvoisin and Buis-les-Baronnies) have permitted the recognition of five bentonites. All five horizons can be observed in the Oze section, in part because of exceptional outcropping conditions (Figs. 1 and 3). A bentonite called MBO (Mineralised Bentonite of Oze), ranging in thickness from 1 to 15 cm, is systematically underlain by a carbonate–sulphate mineralisation. This easily correlated bentonite has a regional extension of at least 2000 km2 [43].
The millimetre-thick horizons B1, B2, B3 and B4 are only identified on some sections (Fig. 4).
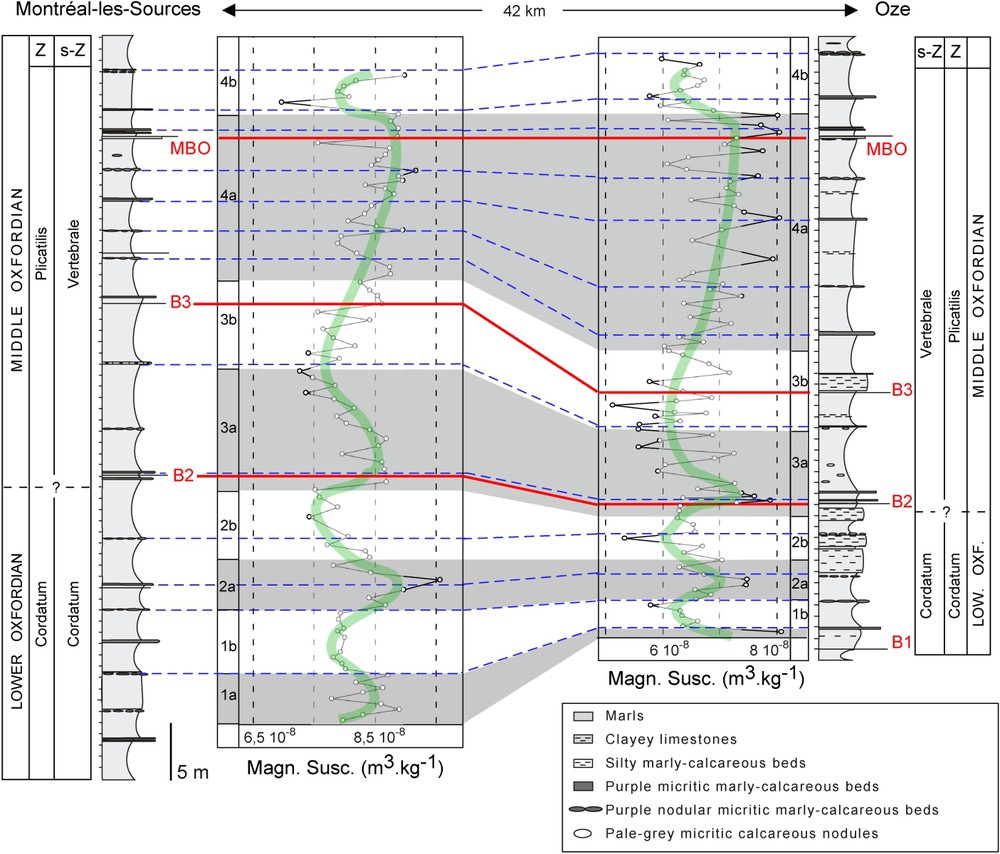
Location and correlation of bentonites in the Terres noires formation from the French Subalpine Basin. Comparison between lithology, biostratigraphy and magnetic susceptibility signal.
Position et corrélation des niveaux de bentonites dans les Terres noires du bassin subalpin méridional. Confrontation avec les données lithologiques, biostratigraphiques et de susceptibilité magnétique.
Using sedimentological and palaeontological data, the correlation of the bentonite horizon is relatively easy, even on 40 km-distant sections, e.g., those of Oze and Montreal-les-Sources (Fig. 4). In the basin, accurate biostratigraphic data based on ammonite zonation allow a high resolution, at horizon scale [22,37], except in the topmost part of the ‘Terres Noires’, where ammonites are less frequent, allowing only calibration at subzone scale.
Other correlation tools include nodular carbonate beds and siderite-rich, rust-coloured carbonate horizons. Magnetic susceptibility (using Kappabridge KLY 2 in the laboratory) studied at the Lower Oxfordian/Middle Oxfordian boundary shows synchronous fluctuations from one section to another (Fig. 4) [41]. The consistency of the various signals validates the correlations and shows that, despite weak fluctuations, magnetic susceptibility is a reliable and useful tool for long-distance correlation in homogeneous formations such as the ‘Terres Noires’.
The five bentonite horizons are comprised between the Cordatum (Cordatum Subzone, B1) and Plicatilis zones (Antecedens Subzone B4). The MBO horizon occurs in the Plicatilis Zone, Vertebrale Subzone. The clay mineral composition is very different from the mineralogy of the bentonite from the Paris Basin and consists of well-crystallised Md kaolinite, and illite–smectite mixed layers including R0 and R1, in proportions that vary from one section to another.
The bentonite geochemistry reveals similar anomalies to those recorded in the Paris Basin. The thin volcanic horizons (B1 to B4) show an intermediate composition in comparison with the enclosing marls. This is usual in millimetre-thick horizons that have been submitted to either bioturbation or deep-current action [21]. Burial diagenesis and fluid circulation may have played a significant role in the bentonite composition and in the precipitation of sulphates and carbonates [43].
4 Discussion
4.1 Origin of terrigenous flux variations
The geographical and stratigraphic distribution of clay minerals from the Lower Callovian to the Middle Oxfordian (Fig. 5) allows the supply, direction, and flux variation of terrigenous sediments to be examined.
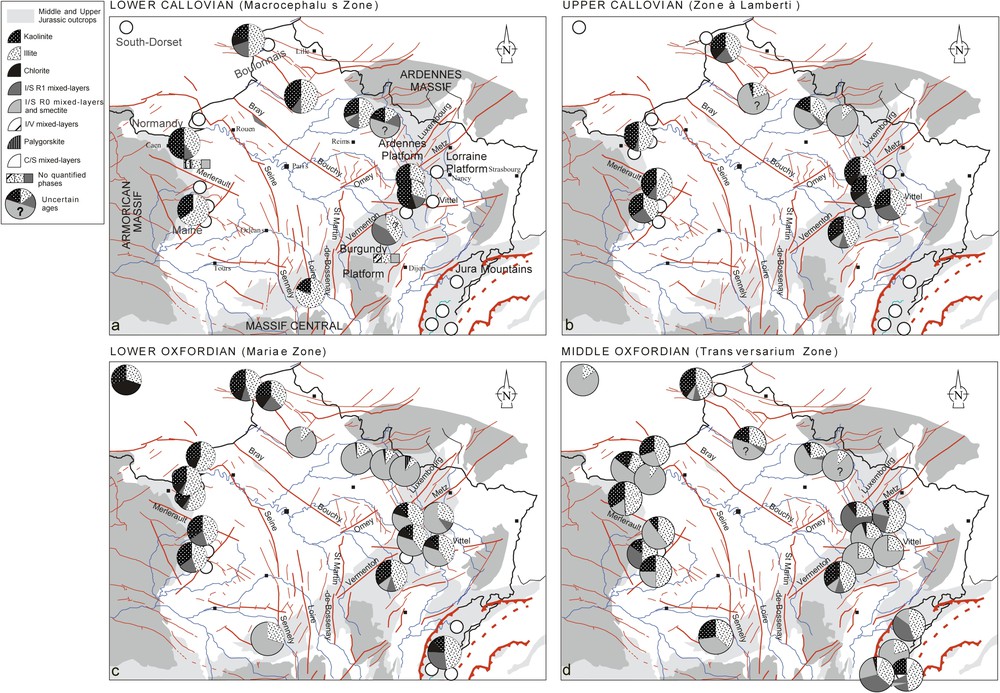
Clay minerals repartition maps in the Paris Basin and the Jura Mountains from the Lower Callovian to the Middle Oxfordian. Data from the Normandy border except Villers-sur-Mer outcrop are from [17].
Carte de répartition des minéraux argileux du Callovien inférieur à l'Oxfordien moyen à l'échelle du bassin de Paris et du Jura. Les données de la bordure normande en dehors de Villers-sur-Mer proviennent de [17].
In the Paris Basin, Upper Bathonian to Lower Callovian terrigenous fluxes are mainly composed of kaolinite and illite (Fig. 5a) because the wide extension of carbonate platforms provided suitable environments for the deposition of these clay species [6]. Warm hydrolysing climates may have favoured the formation of kaolinite on emerged land bordering the Paris Basin (Armorican and Bohemian Massifs, and perhaps even some temporarily emerging limited zones of the Massif Central). Illite, chlorite and I–S mixed layers (R1) are probably inherited from the Palaeozoic basement, as indicated by the occurrence in Callovian sediments of accessory minerals such as zircon, tourmaline, and rutile. The area close to the Ardennes massif is the only one showing a smectite-dominated clay sedimentation from the Macrocephalus and Calloviense zones. This regional distribution suggests that the smectites originated, at least until the Upper Callovian (Fig. 5b), from the London-Brabant massif located to the north. This massif was most likely characterised by low relief favourable to the formation of smectites.
The heterogeneous distribution of clays in the Paris Basin probably results from the complex morphology, including swells and troughs inherited from local tectonics.
As early as the Middle Callovian, the decreasing proportions of kaolinite were balanced by increasing proportions of illite and I–S R1 (Fig. 5a and b). This change can be explained by carbonate platform demise [8,16], and by drier and colder palaeoclimatic conditions suggested by (1) oxygen isotope data from northwestern Europe and Russia [15,45,47], (2) the southern migration of boreal ammonite fauna [5,23,36], and (3) by palynomorph associations [1].
During the Lower Oxfordian, (Mariae Zone), a smectite-rich sedimentation (Fig. 5c) is recorded in the eastern part of the Paris Basin and the North of the Massif Central, indicating a major change in terrigenous fluxes. This change is recorded later, by the end of the Lower Oxfordian in southern England (Dorset) and at the Lower/Middle Oxfordian boundary on the Armorican border [17,18]. Consequently, the onset of the smectite-rich sedimentation in the Paris Basin is diachronous, but results most likely from the same palaeoceanographic event. A similar evolution of the clay assemblages is also recorded in the Central Atlantic [7] during the Oxfordian.
With a second-order maximum flooding occurring in the Mariae Zone (Scarburgense Subzone), the Lower Oxfordian corresponds to a period of high sea level. This maximum flooding recorded everywhere in northwestern Europe [32,40] coincides with the major mineralogical change recorded in the ANDRA boreholes in the eastern part of the Paris Basin. This high eustatic sea level probably played an important palaeogeographic role in connecting basins through opening seaways. We suggest that new terrigenous fluxes originating from the west (western Approaches) entered the Paris Basin as early as the Lower Oxfordian. In addition, the erosion of smectite-rich soils, developed on Atlantic margins (Laurentian–Greenland margin, Irish and Iberian massifs, and Hebrides plateau), may have enhanced the smectite-rich sedimentation. These emerged, relatively distant low-relief massifs, were probably the sources of smectites. These minerals entered the Paris Basin through the Peri-Armorican Trough that corresponded to a narrow subsiding structure favouring the transit of fine terrigenous particles and boreal fauna [18,19,42,48]. Farther to the east, this structure is prolonged by the Seine/Sennely fault system and by the ‘Loire Trough’, allowing smectite to be deposited in the South and the East of the Paris Basin. Therefore, the first connection between the young Atlantic Ocean and the Paris Basin probably occurred during the Lower Oxfordian. These palaeoceanographic changes may also explain the palynological differences between the central and eastern part of the Paris Basin [29].
The changes in terrigenous fluxes from the Lower Callovian to the Lower Oxfordian are recorded neither on the Burgundy platform, nor in the Jura Mountains, nor the Subalpine Basin, reflecting the presence of perennial palaeogeographical barriers at the South of the Paris Basin (Fig. 5a–c). However, straightforward communications between the Paris and Subalpine basins are indicated by migrations of boreal and Tethyan faunas [23,36]. In the Jura Mountains, another major mineralogical change, characterised by increasing proportions of smectites, is recorded during the Middle Oxfordian (Fig. 5d).
In the Subalpine Basin, there is a surprising absence of smectites, compared to the Paris Basin and, except for the eastern margin (Cevenol border), the clay assemblages are particularly homogeneous. The distinct mineralogy of marls and limestones, the bentonite mineralogical composition, the measured on organic matter, and the fluid inclusion temperatures [28] reveal the influence of burial diagenesis, which disturbs the terrigenous composition of the clay assemblages. However, the influence of diagenesis alone is not strong enough to erase the initial terrigenous information completely. The absence of major mineralogical changes in the Subalpine Basin indicates supplies different in origin from those in the Paris Basin. The homogeneous character of the sediments suggests more distal, heterogeneous supplies, for example, from the Bohemian massif, the Provence platform, and perhaps from parts of the Massif Central and sources further afield. The palaeoceanographical modifications recorded in the Paris Basin seem therefore to have little effect on the terrigenous fluxes in the Subalpine Basin. The Cevenol border, however, records an increase in smectites from the Upper Callovian, indicating a possible link with the major mineralogical changes in the Paris Basin, thus suggesting a connection between the two areas that merits further investigation.
4.2 Isochronous lines and palaeovolcanism
As Oxfordian bentonites correspond to instantaneous isochronous lines, they can be used to attempt correlations between the Paris and Subalpine basins. In the Paris Basin, the bentonite horizon from the ANDRA borehole and the Saint-Blin-Semilly section shows the usual smectite-rich mineralogical composition of most bentonite originating from the submarine weathering of volcanic ash beds [21], whereas the bentonite mineralogy of the Subalpine Basin is more uncommon, with a high level of kaolinite. Kaolinite usually characterises volcanic ash deposited in continental environments, where acid fluids originating from decaying organic matter, and a lower level of salinity are favourable to its formation [21,50]. Recently, however, kaolinite-bearing bentonites have been described in open-marine environments, where kaolinite crystals develop preferentially in oxygen-depleted environments, as organic matter decay authorises low pH [14,51].
Several features such as frequent septarias, or the occurrence of mineralisation below some bentonite layers and carbonate beds, indicate that significant fluid circulation occurred in the Terres Noires Formation. The chemical composition of such fluids may be responsible for the formation of authigenic kaolinite in the bentonites. Another difference is the occurrence of I–S R1 resulting from the transformation of smectites, previously formed by submarine weathering of volcanic ash, because of burial depth. The influence of thermal diagenesis is confirmed by high values of the index and by palaeothermometric data performed on organic matter and fluid inclusions [28].
In the Paris Basin, the smectite-rich composition of the bentonite confirms the negligible influence of burial diagenesis. The KAr dating of the horizon provides an age of 144.6 Ma (±4.9) that is significantly younger than the stratigraphic age (about 158 Ma) for the Lower Oxfordian/Middle Oxfordian boundary [27]. This younger age corresponds most probably to a discrete diagenetic event occurring some million years after sedimentation [49].
The geochemical fingerprint of the bentonites, based on trace-elements and immobile REE, allows correlation and provides information as to the type of volcanism [12,30]. The comparison of the geochemical profiles and discriminant function analyses shows that the bentonite from the Paris Basin and the MBO horizon in the Subalpine Basin are geochemically similar [43]. Consequently, we interpret these levels as resulting from a coeval explosive event and suggest a prominent stratigraphic marker for the Lower/Middle Oxfordian boundary along a 400 km line between the two sedimentary basins (Fig. 3). This correlation is coherent with the biochronological data.
The geochemical characteristics of the less-contaminated thick bentonites allow magmatic or volcanotectonic setting discrimination diagrams to be constructed. These diagrams plot a differentiated alkaline volcanism of trachyandesitic composition in a continental rifting context [43]. The origin of the active sources seems linked to the start of rifting in the North Atlantic domain, both from the bentonite geochemical fingerprint, and from what is known of the geodynamic contexts and active sources of the period under consideration. In the North Atlantic rifting context, the strato-volcano in Zuidwal (The Netherlands), near the North Sea margin, is a possible active source. This imposing strato-volcano, less than 500 km east of the Paris Basin, was subject to paroxysmal Jurassic explosive eruptions, probably corresponding to ultraplinian activity, as is shown by the volcano geometry, the thickness of the pyroclastic deposits and proposed radiometric data () from pyroclastic breccias [53].
The bentonite geochemical fingerprint is consistent with lavas and pyroclastic deposit geochemical features from the Zuidwal volcanic centre [34]. Because no other volcanic centre with the same geochemical characteristics is identified, the Zuidwal volcanic centre appears to be the best source for Oxfordian ash falls. Oxfordian bentonites from the two French basins are thus the sedimentary expression of huge ultraplinian explosive events, with distribution greater than 900 km for the Subalpine Basin. Such events are consequently of the same intensity than cataclysmal pre-historical or mega-eruption preserved in fossil record [38]. Such volcano-sedimentary levels correlated at the northeastern European scale are probably the visible remains of the paroxysmal phases of a more constantly active aerial explosive volcanism.
5 Conclusions
The progress made in the study of the mineralogical evolution of the Paris Basin, the Jura Mountains and the Subalpine Basin in the Callovo-Oxfordian brings to light an apparently complex image of clay assemblage distribution. The fragmentary data collected so far do not allow any detailed understanding of the mineralogical variations recorded in the basins or any certainty as to the supply sources involved. Nevertheless, they do allow some perception of the main evolutionary tendencies, the causes of which are chiefly climatic and eustatic. This climatic evolution, i.e. the Middle Callovian to Lower Oxfordian cooling, from isotopic and faunal data, is consistent with the terrigenous information indicating a decrease in kaolinite and an increase in illite and illite-rich mixed layers (R1) from the Middle Callovian onwards. The absence of kaolinite from the Lower Oxfordian and above could possibly be related to the maximum cooling that prevented extended hydrolysis. However, this evolution in terrigenous composition, related to the pedogenesis and erosion of the nearby emerged continental areas, is affected by major palaeogeographical modifications likely to affect the distribution of fine terrigenous particles. The smectite-rich sediments deposited in the South and the East of the Paris Basin during the Lower Oxfordian (Mariae Zone), or in the western basin margin (Normandy) at the Lower/Middle Oxfordian boundary probably arrive from distal sources. Diachronism in the smectite-rich deposit phase can be seen at the scale of the Anglo-Parisian Basin, indicating localised tectonic activity. The major mineralogical change associated with global high sea levels reflects modifications in the distribution of seawater masses, which facilitated connections to adjoining basins (northern basins and western Approaches to the young Atlantic domain). In contrast to the Paris Basin, characterised by complex clay assemblage distribution, the Jura Mountains, the Burgundy platform and the Subalpine Basin show an apparent homogeneity in the clay assemblages from the Lower Callovian to the Middle Oxfordian (Plicatilis Zone). Despite marked burial diagenesis, the mineralogical differences with the Paris Basin suggest different supply sources, at least for the Oxfordian. Such differences imply topographical barriers likely to stop terrigenous influx from the north.
This high-resolution study of the clayey deposits has allowed the identification of bentonites in the Oxfordian sediments of the Paris Basin and of the Subalpine Basin. These bentonites correspond to ultradistal plinian fallouts, probably from active sources on the western margin of the North Sea, such as the Zuidwal complex (The Netherlands). These layers are the sedimentary expression of paroxysmal explosive volcanic events, probably linked to a differentiated alkaline volcanism in a North Atlantic rifting context. They provide prominent stratigraphic markers that allow fine intra- and inter-basin correlation. Their mineralogical signature also allows the intensity of burial diagenesis to be measured.
Acknowledgements
We are very grateful to ANDRA for providing both financial support and access to data without which such detailed observations would have been impossible. We thank the two anonymous reviewers for their helpful and constructive criticism. Grateful thanks to Carmela Chateau-Smith for assistance with the English version.