Version française abrégée
L’Islande est dans une position clé pour comprendre les interrelations entre le climat et les changements de la circulation thermohaline. En Islande du Nord, l’ampleur de l’extension de la calotte au dernier maximum glaciaire (Last Glacial Maximum, LGM) et au Dryas récent (YD) est controversée. Généralement, il est admis que la dernière glaciation s’est étendue jusqu’au rebord de la plate-forme de l’île, au Weichsélien [24,27,38], sur la base de la position de moraines terminales non datées à l’ouest et au sud-ouest de l’île [12,28,29] (Fig. 1a) et de l’altitude atteinte par les volcans sous-glaciaires (móbergs) [38]. Nous attribuons indirectement un âge MIS 12 (446 ka) à cette extension maximale de la calotte (Tableau 1). La présence du tephra de Skógar dans la Fnjóskadalur (à l’est d’Akureyri, Fig. 1a) et sa corrélation avec le tephra de Vedde, daté à 10,2 cal. ka, constituent l’autre argument [38] utilisé pour justifier cette importante extension par rapport aux autres calottes de l’Atlantique nord [9,34,43]. La déglaciation est, de ce fait, attribuée au Dryas récent ou au Préboréal [27,28,38].

(a) Location of the sites; (b) dynamic of ice retreat during LGM and Late Glacial.
Fig. 1. (a) Localisation des sites ; (b) dynamique du retrait glaciaire pendant le dernier maximum glaciaire et le Tardiglaciaire.
K-Ar dating of basalts (LSCE, Gif-sur-Yvette, France)
Tableau 1 Datation K–Ar de basaltes (LSCE, Gif-sur-Yvette, France)
Sample ID | Experiment No. | Weight molten (g) | K* (wt%) | 40Ar*(%) | 40Ar* (10−13 mol g−1) | 40Ar* ± 1 σ (weighted mean) | Age ± 2 σ (ka) |
ISLN-22 Karahnjukur: (tunnel). | 6844 | 1.56899 | 0.589 ± 0.006 | 0.531 | 2.356 | 2.459 ± 0.057 | 241 ± 12 |
Elevation: 525 m. | 6868 | 1.14643 | 0.544 | 2.673 | |||
ISLN-21 Ytrikarahnjukur | 6923 | 1.06213 | 0.365 ± 0.004 | 1.367 | 4.795 | 4.684 ± 0.073 | 740 ± 27 |
Base of recent ridge: | 6957 | 1.06165 | 1.686 | 4.481 | |||
ISLN-35 Basal lava flow of Snaefell. | 7127 | 1.18148 | 1.112 ± 0.011 | 2.315 | 4.927 | 4.927 ± 0.062 | 255 ± 8 |
Elevation; 677m | 7143 | 1.69880 | 3.583 | 4.927 | |||
ISLN-35 Basal lava flow of Snaefell. | 7128 | 1.15370 | 1.187 ± 0.012 | 3.585 | 5.520 | 5.649 ± 0.062 | 274 ± 8 |
Elevation; 682m | 7144 | 1.53762 | 4.481 | 5.736 | |||
SN-10 Top lava of Snaefell | 7196 | 1.07774 | 3.686 ± 0.037 | 3.665 | 16.951 | 16.193 ± 0.092 | 253 ± 6 |
Elevation: 1533m | 7211 | 1.45909 | 3.271 | 15.805 | |||
ISLN-24 Sandfell | 6887 | 1.79824 | 0.631 ± 0.006 | 0.414 | 1.674 | 1.638 ± 0.046 | 150 ± 9 |
6903 | 1.72547 | 0.391 | 1.616 | ||||
ISLN-43: Moberg Vatnafell | 7219 | 1.22993 | 0.639 ± 0.006 | 5.922 | 4.722 | 4.583 ± 0.044 | 414 ± 11 |
7233 | 2.28773 | 4.293 | 4.535 |
Des observations récentes et datées à terre, dans le Sud [16] et au large de la côte nord-ouest [4,5], ont permis de mettre en évidence une extension glaciaire au dernier maximum glaciaire plus limitée, conforme aux observations anciennes [13,26]. Une reconnaissance géologique effectuée lors des travaux du barrage Halslón (Kárahnjúkar Hydropower Plan, Islande orientale), à 20 km au nord-est du Vatnajökull, a permis l’observation d’un complexe lacustre ancien, puissant de 90 m, dans un secteur actuellement à pergélisol discontinu (550 m d’altitude [23]). Ce complexe paléolacustre (Fig. 2a) a été attribué à une sédimentation en amont d’un barrage créé par le volcan récent Kárahnjúkur et résulterait de la déglaciation finale (Préboréal) [31]. Cette interprétation n’est pas confortée par les observations de terrain.
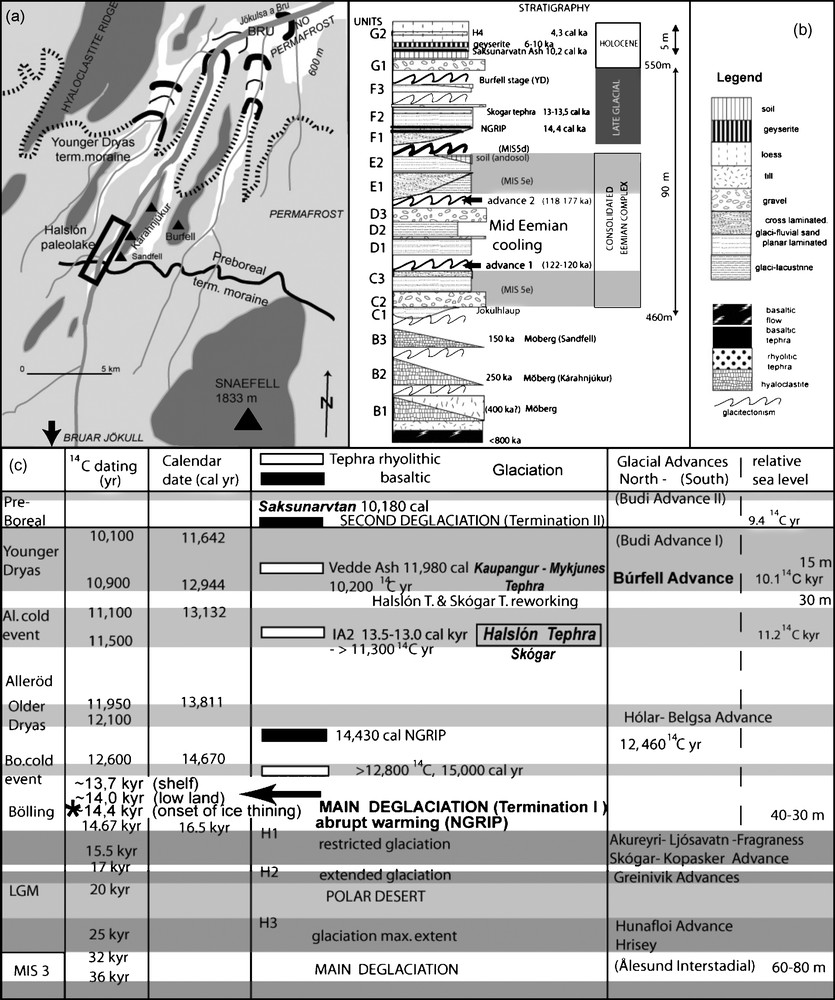
(a) Detail map of the site with, in black, the Búrfell terminal moraines, in dark grey, the hyaloclastite ridges; (b) compound stratigraphical log of the Halslón sedimentary record; (c) summary of the stratigraphical position of the main Late Glacial tephra. * With courtesy of J. Licciardi.
Fig. 2. (a) Localisation des sites avec les moraines terminales du Búrfell et en gris foncé, les crêtes de hyaloclastite ; (b) log stratigraphique composite de l’enregistrement sédimentaire d’Halslón ; (c) résumé de la position stratigraphique des principaux tephras tardiglaciaires. * Source : J. Licciardi.
L’âge de la formation volcanique basale du complexe (Kárahnjúkur) a été daté par la méthode K–Ar à 150 ± 9 ka (Tableau 1). Le complexe sédimentaire enregistré présente deux séquences de dépôts (Fig. 2b).
La séquence basale (C–D–E) est consolidée et admet trois unités fluvio-lacustres et glacio-lacustres, riches en sables hyaloclastitiques, tronquées et déformées à trois reprises par une glacitectonique puissante. Dans la vallée, les déformations glacitectoniques ont créé, par surconsolidation des unités précédentes, des massifs résistants, faisant office de barrages. Il n’y a pas d’évidence de barrage d’origine volcanique. L’intensité et le style des déformations glacitectoniques attestent une dynamique de glacier à base tempérée, uniquement compatible avec un contexte régional sans pergélisol, donc interglaciaire à cette altitude (550 m). La dernière unité (E3) présente des indices d’eaux claires (bioturbation, déglaciation complète en amont) et une pédogenèse marquée (andosol hydromorphe).
La séquence supérieure (F–G) est bipartite. L’unité F est constituée de trois séquences fluvio-glaciaires non consolidées (F1–2–3), relativement pauvres en hyaloclastites et déformées par la glacitectonique. La dernière déformation appartient au stade glaciaire de Búrfell, d’âge non défini, recoupant la vallée de la Jökulsa à Brú, au nord du Kárahnjúkur (Fig. 1b). Quelques moraines plus récentes affleurent à 4 km du front actuel du Búarjökull. Les unités G sont non déformées et consistent en un épandage dominé par le fluvio-glaciaire, surmonté par un tephra basaltique épais attribué au Saksunarvatn (10,2 cal. ka) – K. Grönvold in [23] –, une geysérite datée à 10 et 6,5 ka (U–Th, Tableau 2) et de lœss postglaciaires. L’unité inférieure (F1) remanie un tephra basaltique inconnu. La seconde (F2) remanie un tephra rhyolitique identique au tephra de Skógar, souvent assimilé à celui de Vedde (12 cal. ka [37,47], Tableau 3).
Geyserite U–Th dating (GEOTOP, Montreal, Canada)
Tableau 2 Datation U–Th de la geyserite (Geotop, Montréal, Canada)
Sample | 238U (ppm) | ± | 234U/238U activity | ± | 230Th/234U activity | ± | 230Th/232Th activity | ± | U/Th age (yr) | ‘+’ | ‘−’ | U/Th corr. age * (yr) | ‘+’ | ‘−’ |
Geyserite- | 0.07001 | 0.00044 | 1.9924 | 0.02710 | 0.08920 | 0.00240 | 4.54 | 0.11 | 10 090 | 278 | 277 | 6418 | 270 | 270 |
Tephra normalized chemistry, EDX on single grain (UMR PBDS, Villeneuve-d’Ascq, France)
Tableau 3 Composition chimique normalisée des tephras, EDX sur grain unique (UMR PBDS, Villeneuve-d’Ascq, France)
Norm. | Halslón F2 | Halslón F2 1 sd. | Skógar Inf. | Skógar Inf. 1 sd | Kaupan. Rhyol. | Kaupan. Rhyol. 1 sd. | Mykjunes Rhyol. | Mykjunes Rhyol. 1 sd. | Vedde asha | Vedde ash 1 sd | Halslón F1 | Halslón F1 1 sd. | NGRIP 1595 mb | NGRIP 1595 mb1 sd. | Halslón G2c | Halslón G2 | NGRIP 1409 mb | NGRIP 1409 mb1 sd. |
SiO2 | 72.49 | 0.91 | 72.38 | 0.85 | 73.03 | 0.51 | 72.37 | 0.86 | 72.29 | 0.39 | 53.74 | 1.68 | 49.38 | 0.85 | 49,8 | ? | 48.80 | 0.54 |
TiO2 | 0.45 | 0.18 | 0.41 | 0.18 | 0.27 | 0.15 | 0.14 | 0.13 | 0.29 | 0.04 | 2.53 | 0.47 | 2.03 | 0.06 | 1,5 | ? | 2.77 | 0.09 |
Al2O3 | 12.30 | 0.41 | 12.27 | 0.54 | 13.82 | 0.57 | 11.91 | 0.86 | 13.64 | 0.19 | 13.59 | 0.68 | 14.09 | 0.60 | 14,7 | ? | 13.59 | 0.22 |
FeO | 3.95 | 0.47 | 4.14 | 0.67 | 4.00 | 0.93 | 3.40 | 0.58 | 3.84 | 0.13 | 15.33 | 1.27 | 13.10 | 0.32 | 11,0 | ? | 13.68 | 0.42 |
MnO | 0.20 | 0.19 | 0.15 | 0.10 | 0.21 | 0.57 | 0.52 | 1.22 | 0.15 | 0.04 | 0.19 | 0.12 | 0.23 | 0.02 | 0,2 | ? | 0.23 | 0.02 |
MgO | 0.54 | 0.23 | 0.35 | 0.13 | 0.32 | 0.62 | 0.14 | 0.13 | 0.23 | 0.03 | 4.31 | 0.61 | 6.01 | 0.65 | 7,9 | ? | 0.23 | 0.02 |
CaO | 1.46 | 0.22 | 1.26 | 0.25 | 1.23 | 0.14 | 1.44 | 0.56 | 1.31 | 0.07 | 8.14 | 1.03 | 9.81 | 0.30 | 13,6 | ? | 10.29 | 0.25 |
Na2O | 4.38 | 0.53 | 4.91 | 0.55 | 3.44 | 1.10 | 3.30 | 0.58 | 4.70 | 0.29 | 1.38 | 0.55 | 2.03 | 0.20 | 2,2 | ? | 2.44 | 0.10 |
K2O | 3.59 | 0.52 | 3.72 | 0.39 | 3.81 | 0.22 | 4.29 | 0.71 | 3.56 | 0.14 | 0.47 | 0.23 | 0.43 | 0.05 | 0,2 | ? | 48.80 | 0.54 |
Nb. spl | 45 | 55 | 48 | 25 | 100 | 32 | 7 | 7 | 13 | |||||||||
Stratigr | 12 ka = Skogar | Skogar loc. typ. Probably 12 ka | Kaupan. < Skogar Probably 10.3 ka | Kaupan. > 9.8 ka Probably 10.3 ka | Veddea10.3 ka | NGRIP 14.08 ka ?? | NGRIP 14.08 ka | Saksunarvatn 10.2 ka | NGRIP 10.2 ka |
De nouvelles analyses des tephras rhyolitiques régionaux dans leur contexte stratigraphique (Halslón, Skógar, Kaupangur, Mykjunes, Vedde ; Tableau 3, Fig. 2a) ont permis de repositionner le tephra d’Halslón vis-à-vis des données publiées, vers 13,5 cal. ka ou 12,0 14C ka [37] (Fig. 2c) et, par conséquent, de donner une signification stratigraphique logique au tephra basaltique F1, chimiquement très proche du 14,2 cal. ka de NGRIP [37].
Le fait que le complexe d’Halslón, à seulement 20 km du bord de la calotte du Vatnajökull, soit encadré par des dates de 150 ka à la base et vraisemblablement 12 14C ka pour F2, suggère que la déglaciation principale a été beaucoup plus précoce que ce qui est généralement admis [27,28,38] et qu’elle est vraisemblablement effective à partir du Bølling (13,5 ka), donc en conformité avec la terminaison IA de l’Atlantique nord [6]. Le stade de déglaciation final de Búrfell, qui affecte F3, mais non le tephra de Saksunarvatn (10,2 cal. ka), pourrait donc tracer les limites du Dryas récent et du Préboréal (Fig. 2a). Cette déglaciation rapide est conforme (a) aux observations faites dans le rift, mais également dans le Nord-Est de l’île [46] et (b) à une extension réduite de la calotte nord LGM, avec désert polaire, comme l’avaient suggéré certains auteurs [22,41,44]. Elle dénote une aridité importante qui corrobore les observations faites dans le Sud [16,37] et le Nord-Ouest de l’île [4,5], ainsi que l’extension des glaciers rocheux fossiles [22]. La faiblesse des précipitations nivales enregistrées à cette époque au Groenland [2], associée à une forte activité éolienne [9], dénotent un fonctionnement analogue de cette calotte pour les mêmes périodes. Une déglaciation importante de l’île au Bølling, avec un Dryas récent très proche des fronts glaciaires actuels, est similaire aux observations faites au Spitzberg [32] et à ce qui est enregistré dans l’Atlantique nord [6,32].
Par conséquent, la séquence interglaciaire de base (unités C–D–E, <150 ka) ne pourrait donc appartenir qu’à l’Eémien, comme l’attestent la pédogenèse développée à son sommet en E3 et la bioturbation, témoignant d’une déglaciation complète du Vatnajökull. Les évènements climatiques enregistrés en son sein sous forme de dépôts proglaciaires et de plusieurs épisodes de glacitectonique reflèteraient, sous la forme d’avancées glaciaires, le refroidissement intra-Éémien ou MEEC, maintenant reconnu un peu partout [17,18,36,42,45] et aussi suspecté dans le Rift islandais du Nord-Est [23]. Cet événement est composé de deux coups de froids successifs à 122–120 et 118–17 ka [36], qui pourraient correspondre aux discontinuités C/D et D/E, avec l’installation probable d’un pergélisol en fin de D. Le fait que les glaciers semblent absents après ces événements (unité E3) montre aussi que la dernière discontinuité, E/F, correspondrait à l’extension véritable de la calotte islandaise vers 110 ka, donc au début MIS 5d.
La précocité de la glaciation et l’aridité au dernier maximum glaciaire en Islande confirment donc que les glaciers se développent surtout quand les précipitations et le niveau marin sont encore élevés [40]. En fait, l’Islande du Nord enregistre un désert polaire au dernier maximum glaciaire entre 25 et 15,5 ka (Fig. 1b). Par comparaison, la calotte scandinave se développe également à partir de 80 ka (MIS 5b [43]) sur la plateforme de Barents, avec une ré-extension réduite au début et en fin du MIS 2, comme au Groenland ou en Sibérie [15,18]. Cette aridité au dernier maximum glaciaire et une déglaciation précoce au cœur de l’Atlantique nord attestent une déflection vers le sud du Gulf Stream et du courant d’Irminger (IC), en réponse à l’extension de la banquise. Actif depuis le Bølling [24], le courant d’Irminger a été repoussé lors d’arrivées importantes d’eau de fonte dans l’Atlantique nord [2,14,29]. Cette dynamique se poursuit jusqu’au début de l’Holocène (Heinrich 0) [2,14,29,32], pour disparaître avec le redémarrage de la circulation thermohaline [1,3]. Au cours du dernier maximum glaciaire et des coups de froid du Tardiglaciaire, l’arrêt du courant d’Irminger, le froid et l’extension de la banquise au nord de l’île réduisent les précipitations sur le versant nord de la calotte islandaise. Pour conclure, ces observations montrent que, même au milieu de l’Atlantique, comme au nord de l’Islande, les glaciations se développent surtout au début du Glaciaire, alors que le maximum de froid voit le développement d’un désert polaire au nord de 55° de latitude nord.
1 Introduction
Iceland is at a key position to understand the link between climate change and modification in the thermohaline circulation. In northern Iceland, the glacial extent at the Last Glaciation Maximum (LGM) and at the Younger Dryas (YD) is controversial. Some authors [24,27,28] argued for an extended glaciation reaching the outer border of the island platform or shelf (Fig. 1a) during the Weichselian. This hypothesis of large ice volume was deduced from the position of undated grounded terminal moraines, such as those located at the extreme West of Iceland, as on the Latra ridge (Fig. 1a) [28] or on both sides of the Reykjanes ridge, at the extreme West-Southwest of the island [12,39] (Fig. 1a). A late deglaciation at the coastline during the Younger Dryas (YD) or even the Preboreal (PB, [27,28,38]) is commonly proposed in the same manner. A tephra (Skógar) found in coastal sites and its possible correlation with the Vedde Ash (PB) are used to date this limited deglaciation [38]. Móbergs (subglacial table volcanoes) are also utilised as ice-sheet thickness indicators, and are used to map the LGM boundaries too.
Recently, inland geological observations in the South, supported by dating [16], and on the northwestern shelves [4,5] are in favour of a more limited extent of the LGM and of the YD [16], confirming earlier observations [13,26]. The tephra record in the North Greenland Ice Core (NGRIP [37]) also suggests a strong deglaciation in Iceland from the onset of the Late Glacial warming – known as the Bølling – onward. A more limited ice volume should modify the climate reconstruction for the northern Atlantic, especially during the LGM and the following deglaciation.
During the prospecting work for the construction of the Halslón dam (Fig. 2a; Kárahnjúkar Hydropower Project East Iceland), 20 km to the northeastern edge of the Vatnajökull, a 90-m-thick palaeo-lacustrine complex, apparently dammed by the recent Kárahnjúkur volcano, was discovered [23]. It is located today in a discontinuous permafrost environment (altitude: 550 m). It was first considered as the record of the last deglaciation [31]. The last terminal moraines, the undated Búrfell stages, are running from east to west immediately north of the Kárahnjúkur móberg (Fig. 1a), contouring the Snaefell volcano, and are considered as Holocene [31]. Some younger terminal moraines crop out closer to the Búarjoküll (Vatnajökull outlet) and the Little Ice Age terminal moraine crops out at 4 km from the present-day glacial front. The absence of organic deposits limits the dating possibilities.
Based on new field observations, new data interpretations and new radiometric ages, we propose an alternative interpretation of this record. For that purpose, we question the age of the maximal glacial extent in Iceland. In this contribution, we present the results of a 3D analysis, of tephrostratigraphy, and a collection of isotope data that do not support short-time span chronology, as published by Nordðhal [38]. Rhyolithic tephra, as the Skógar s.s. sampled in the Fnjóskadalur (northern Iceland), Mykjunes and Helka 4 (Ytri Ranga, southern Iceland) were sampled for the sake of comparison. We also refine the mapping of the terminal moraines from northern, southern and western Iceland.
2 Data
2.1 Description and sedimentary interpretation
The base of the sedimentary sequence (Fig. 2b) is formed by a subglacial volcano (or móberg) K–Ar dated between 274 ± 8 and 253 ± 6 ka (Table 1). This unit is truncated by glacial erosion and the deposits of a second móberg dated 150 ± 9 ka, which has also been also re-trimmed by glacial erosion.
2.1.1 The sedimentary complex
The sedimentary complex consists of two sequences of fluvio-glacial to glacio-lacustrine deposits.
The lower set (C–E) is usually consolidated and consists of three hyaloclastite-rich sand units, separated by unconformities triggered by strong glacitectonic truncation and deformations:
- • unit C is a sand body that represents a deglaciation complex, covered by a braided sandy plain towards the top. It is deformed by transpressive glacitectonic (lateral compression with a strike-slip component, leading to folding or faulting);
- • unit D corresponds to a short-lived glacial pulse that was followed by an ice-contact lacustrine fan with dropstones, also representing a deglaciation sequence. Emersion is recorded at the top in the form of oxidation and permafrost development before a major erosion and glacitectonic stretching (transtension);
- • unit E is a sequence of fluvio-glacial deposits forming a braided plain, locally dammed, and ending with a bioturbated sandy marshy soil (hydromorphic andosol). It is eroded and deformed by glacitectonics.
2.1.2 The upper sequence
The upper sequence is unconformable with the lower one, mostly unconsolidated, and consists of three shallow fluvio-glacial units, somewhat deformed by glacitectonics with locally preserved tills:
- • the oldest unit (F1) locally reworks at least one basaltic tephra (Table 3); close to its top, the second one reworks a rhyolitic tephra (F2), which is geochemically identical to the Skógar tephra. The youngest one (F3) is discontinuous and shows evidences of strong reworking by glacitectonics (transpression and transtension) during the last regional glaciation (undated Búrfell outer stage);
- • these units are capped in unconformity by recent fluvio-glacial deposits with some glacio-lacustrine deposits with beaches (unit G1) and loesses (G2), including at the base the Saksunarvatn basaltic tephra (Grimsvotn, (10.2 cal. ka, K. Grönvold in [23]), a U–Th geyserite dated between 10 and 6.5 ka (Table 2) and several rhyolitic tephras, as Hekla 4 (4300 yr BP [23]).
2.2 Dammings
Damming events, leading to the sedimentary accumulation of the lower complex (units C–D–E) are associated with strong glacitectonics and sediment overconsolidation, probably also with terminal moraines. Except for the 250-ka event (Table 1), collected in a work tunnel in the Kárahnjúkur móberg, evidences for other recent volcanic damming events are lacking. The strong intensity and the transpressive style (stretching and folding) of the glacitectonics are in favour of a temperate-based glacier. The important deglaciation formed in such close vicinity of the Vatna ice cap also supports an interglacial context. It is interpreted as a complex developed during the Last Interglacial (<150 ka). At the top of the infill, the foot of the Kárahnjúkur móberg presents three generations of periglacial screes. The oldest one is deformed by glacitectonics (undated Búrfell stage). The outer Búrfell terminal moraine (Fig. 2a) is responsible for the youngest temporary damming of the drainage (pre-Saksunarvatn basaltic tephra) north of the Kárahnjúkur volcano.
2.3 Tephrostratigraphy (Fig. 2c)
2.3.1 Rhyolithic tephra
The resedimented ‘Skógar’ tephra found at Halslón has been compared with others. The Kaupangur tephra has been sampled in situ at 15 m, on a buried beach truncating a glaci-marine fan containing the resedimented Skógar tephra [38]. The newly found Mykjunes tephra has been sampled on the top of the Younger Dryas terminal moraine (flow till) (Fig. 2a). The Halslón tephra is chemically identical to the basal tephra of the Skógar type locality and rather close in composition to the ‘standard’ Vedde Ash dated to 11 980 cal. yr BP (10 30014C yr BP) [37,47], as well as the in-situ younger Kaupangur tephra (Table 3; Fig. 1c) and the late YD Mykjunes tephra. The shape of Kaupangur and Mykjunes tephra is pellet-like, though the Skógar-and-Halslón one is mostly elongated. However, the Mykjunes tephra seems slightly poorer in Al and richer in Mn than the standard Vedde ash. Weathering can be advocated to explain these slight differences (Table 3).
2.3.2 Basaltic tephra
Below the Saksunarvatn basaltic tephra (10.2 cal. ka), and the Halslón tephra, the reworked basaltic tephra in unit F1 at Halslón is also chemically similar to the 14.2 cal. ka basaltic tephra of NGRIP [37] (Table 3).
Bond [7] already suggested the existence of another ‘Vedde-like ash’, older than 11 300 cal. yr BP. A recent observation of older Vedde-type tephras was done in NGRIP and dated by direct counting to 13 500 cal. yr BP [37]. In addition, a very old Vedde-type tephra was analyzed just above a peat yielding 12.9 14C ka BP (15 cal. ka BP) in western Norway [33]. Present work also suggests the existence of two ‘Vedde’ ashes in northern Iceland, one related to a sea level at around 35 m a.s.l., and the other one at 15 m a.s.l. With a rapid glacial rebound [28], this should only result in a ca 600–500-yr age difference [7], suggesting that the Skógar tephra may be 15 cal. ka old. Consequently, the Halslón tephra may be at least 15 cal. ka or 12 ka 14C yr BP. The correlation of the basaltic tephra F1 with a 14.2 cal. ka age is coherent. Stratigraphical data are summarized in Fig. 2c.
3 Implications
3.1 Last deglaciation
The sandwiching of the most part of the Halslón sedimentary record between min. 12.0 14C ka BP and the basal móberg dated to 150 ka, at only 20 km from the edge of the Vatnajökull, might suggest that the last deglaciation occurred much earlier than generally accepted. At Halslón, the outer Búrfell terminal moraine (Fig. 2a) and the undisturbed Saksunarvatn basaltic tephra (10.2 cal. ka) support a limited ice extent during the Younger Dryas for the northern side of the Vatnajökull. Moreover, the Kaupangur tephra, south of Akureyri, crops out above a beach level at 15 m a.s.l., marking the strongest glacial rebound prior to the Younger Dryas (YD) and an important sea level rise as well. In this valley, the YD terminal moraine crops out at Hólar, 40 km upstream from the mountain of Kaupangur, but only at some 10–20 km from the watershed divide. This implies that the YD was of very limited extent and that the Eyafjördur glacial system was independent of the main ice cap (Fig. 1b). This situation is very close to the deglaciation in southwestern Iceland [16,29]: the newly found Mykjunes tephra was deposited in the very late YD, prior to the Preboreal warming and the main Budí advance (Fig. 1a). This restricted ice cap from the Bølling should also support the hypothesis [37] according to which the higher frequency of ash deposition from the Bølling volcanoe's indicates that large ice-free surfaces existed in Iceland during the Bølling–Allerød, the southern face of the Icelandic ice sheet being probably more developed, as suggested by the extent of the Budí terminal moraines, attributed to the YD (outer) and Preboreal (inner; Fig. 1a) [16]. Our recent finding of the Vedde–Mykjunes ash at the top of the outer morainic ridge consolidates this view. A Bølling age for the main deglaciation in Iceland is thus in conformity with Termination IA, the major deglaciation event of the Northern Atlantic regions [6]. Late Glacial re-advances result from surging valley glaciers in zones submitted to a high geothermal gradient, not from an ice-cap development (Fig. 1b). The delay (Fig. 2c) between the onset of the warming recorded isotopically at NGRIP and the record of deglaciation on the shelf seems logical, because of the timing of thinning and retraction of the ice sheet edge. Moreover, the outermost Latra terminal moraines, at the edge of the shelf, has been indirectly dated, by means of the Vatnafel Móberg (Snaefelness), to circa 414 ka (Table 1), ageing this maximal extent to one of the most powerful glaciation in Quaternary, the MIS 12.
3.2 Last Interglacial and Mid-Eemian Cooling (MEEC)
The Halslón record suggests the preservation of a MIS 5e interglacial complex in Iceland. Preservation of such complexes is compatible with a cold-based glaciation combined with a limited LGM ice extent in North Iceland [46]. The strong glacitectonics events near the Vatna ice cap and especially the Halslón D unit imply unequivocally a temperate-based glacier (no basal permafrost) and a rapid glacial surge in a moist ‘interglacial’ context. The transitions C/D and D/E may be the products of the mid-Eemian cooling [17,18,36,42,45], as also suspected already in the NE Rift Zone, where this ‘interglacial complex’ has been dated to earlier than 80 ka by K–Ar; it includes a high-stand doublet and three well-developed pedogenesis and loess [46]. Usually, this event in ocean and ice cores consists of two pulses, one at 122–120 ka, the other one at 118–117 ka [36] (Fig. 2b). The top of unit E (E3) shows evidences of clear water, the trace of a restored full deglaciation after the two consecutive glacial advances. As for the Holocene Optimum, the Vatna ice cap vanished during the Eemian Optima (127 ka and 116 ka; [36]). E/F transition probably represents the real extent of the main ice sheet at the onset of MIS 5d, circa 110 ka. The twinned glacial advances attributed to the MEEC at Halslón attest to a rapid reaction of ice sheets to orbital negative forcing in a moist climate, as stressed by Maslin [36].
3.3 Global climate: aridity in the central North Atlantic during the most part of the Weichselian
A glacier usually develops when precipitation and sea level are relatively high [40], in contrast to the conditions that prevailed during the LGM. A very early extent, starting 80 ka ago, of the Fennoscandian ice sheet has been clearly shown [43] for the Barents shelf, with a further retraction. Some minor re-extents during the early MIS 2 and another one a little more extended just before the Bølling main deglaciation are also documented. All these extents are recorded as glacial surge deposits. Offshore marine observations along the southwestern Icelandic coast [29] also suggest a similar evolution.
Deduced from our results, thermal deglaciation was extensive during the Bølling time over most of Iceland, and certainly by aridity during most of the LGM (between 25 and probably 17 ka) with large ice-free surfaces on the most part of the relieves. Recent results [4,5] also sustain our interpretation for the Northwest of Iceland, today in the rain shadow of the southern ice caps. This confirms earlier views [22,41,44] stating that large areas of northern Iceland have remained ice free during the Weichselian (Fig. 1b), with a pronounced aridity and marine observation off the northern coast [32]. A limited snow accumulation on Iceland during the Late Glacial is also suggested by the NGRIP record [2].
This aridity seems related to frequent southward deflections of the Gulf Stream and of the warm Irminger current (IC) since Bølling times [29]. The deflection of the IC-induced cooling and the precipitation starvation north of the ice sheets are associated with the sea-ice persistence on the North of Iceland. The IC vanished during the Late Glacial cold pulses as a consequence of major input events of cold freshwater in the Atlantic [2,10,14,29]. This pulsed cooling probably went on until the onset of Holocene (H0 event) and the starting of full thermohaline circulation [1,3,32], which resulted in a restored precipitation and the Preboreal extent of glaciers in southern Iceland [16]. The vicinity of the IC since Bølling times [29,32] implies a more limited and thinner ice cap than the usually accepted ones. At Halslón, the deglaciation is fast, implying the melt of a limited ice volume. This is also the case south of Thingvellir (Fig. 1a) and on the Reykjaness peninsula [16]. Moreover, at the outlet of the Thingvellir Lake, we observed a fluvio-marine terrace at +80 m a.s.l. This record evidences synsedimentary iceberg scouring prior to the Búdi glacial advances. This suggests that in this area deglaciation was already effective in the early Bølling times and prior to the Maximal Marine Flooding or ‘upper marine limit’ (MMF circa +110 m a.s.l. in the south, 9.7 14C ka [16]). In addition, the Mykjunes tephra is also found in conformity with sedimentary deposits circa 90 m a.s.l. prior the MMF. Late Glacial basaltic flows are glacially striated because of the Búdi advance in the Ytri Ranga valley (Fig. 1a). In the North, the deglaciation mapping based on the altitude of the lateral moraines in the valleys and on the low altitude of the last móberg suggests that the Late Weichselian ice cap was more extended, but just a little thicker than the Little Ice Age glaciers (LIA, Fig. 1b). The YD extent does not show large discrepancies with the present-day MAAT of 0 °C, southeastern Iceland excepted.
Also supporting this, on Svalbard, the YD advance was not as extended as the LIA one [34]. In the intervals between those Late Weichselian surge events, polar desert conditions prevailed over the Arctic region (>55°N), with the development of periglacial diagenesis, rock glaciers [22] and aeolian sedimentation [9]. A similar situation also prevailed in northern Greenland [15] and in Siberia [18]. The maximal surface of the Icelandic ice sheet (Fig. 1a) during the Weichselian was circa 126 000 km2, and not 400 000 km2, as generally assumed [25,27,38]. It was probably closer to 70 000 km2, prior to Bølling times. Based on móberg (subglacial volcanoes) heights, a thickness higher than 2000 m for the LGM ice sheet is generally accepted [25,38]. Our observations suggest values closer to 700 m inland, supporting the deductions of Mortensen [37]. 3He isotopic dating of emerging móberg in northern Iceland also supports this interpretation [35]. This would imply that the generally accepted values for the Icelandic ice sheet (thickness and extent), close to the LGM, are at least 50% in excess. Following our interpretations, the Icelandic ice sheet would have therefore exerted much less influence on Atlantic climate conditions than previously had been claimed (synthesis in [25]).
4 Conclusion
From our study, we conclude:
- • despite a central position in the North Atlantic, which should imply higher precipitation, the Icelandic ice sheet behaves similarly to the other ice sheets in the same area. This first conclusion gives a more global extent to the concept of Ruddiman and McIntyre [40], who stated that glaciations develop preferentially when the sea level and precipitation were high;
- • the main deglaciation occurred at least at H1 and with the Bølling warming. The Younger Dryas ice cap was very limited in extent;
- • the development of aridity in Iceland during the LGM sea level implies precipitation starvation that limited the growth of the Weichselian ice sheet. Aridity originates (a) during the LGM, from the southern extent of the sea ice and from the southward deflection of the Gulf Stream and Irminger Current, (b) from the onset of the Bølling warming to the onset of the Preboreal, from the input of large volumes of melt water into the North Atlantic Basin and from the retroactive extent of the sea ice. Our chronology of deglaciation is coherent with that of Knudsen [32] on the northern shelf;
- • around LGM, when glaciers developed in tropical regions, ice sheets receded above 55°N because of precipitation starvation controlled by the maximal extension of the sea ice.
Acknowledgements
This work has been funded by IPROCI research Programme from the ‘Institut Paul-Émile-Victor’. We also thank Joe Licciardi for fruitful discussions on deglaciation of móbergs.
Appendix A Addendum: dating and tephra
For the new K–Ar dating at Halslon, only rocks without traces of alteration were selected and prepared following the method described in [8,19] at the LSCE laboratory, France. K determination was carried out by atomic absorption, with a relative precision of 1%. The isotopic composition and concentration of Ar were determined, on groundmass-separated aliquots, using an unspiked technique that has been successfully applied to date Quaternary volcanic rocks [20,21]. This technique is not suitable to check whether the initial 40Ar/36Ar ratio has a value equivalent to the modern atmospheric value. Consequently, errors given in Table 1, which are analytical, may be underestimated.
The Geyserite sample was analyzed at the GEOTOP laboratory, Canada, first dissolved with fluorhydric acid and spiked with a 229Th–236U–233U mixture. Uranium and thorium fractions were separated on anion exchange columns using standard techniques. Both uranium and thorium were loaded onto graphite-coated Re filaments and analyses carried out using a VG Sector mass spectrometer. The latter is equipped with an electrostatic analyser and an ion-counting Daly detector. Errors were propagated from the in-run statistics and the uncertainties on the spike isotopic composition. Ages were calculated using the standard equation, and the decay constants used for 234U, 238U, 230Th and 232Th were 2.835 × 10−6, 1.55125 × 10−10, 9.1952 × 10−6 and 4.9475 × 10−11 yr−1, respectively. Given the low uranium concentration and the young age of this sample, the relatively high 230Th/232Th ratio cannot be fully representative of an important detrital contamination; consequently the corrected age [11,30] must be considered with caution. This is why we prefer to give an age window whose limits are a corrected age and an uncorrected one. Tephras were analyzed by SEM with an EDX microprobe analyzer on single clean grains, at the PBDS laboratory, Lille, France. Please notice that our interpretation of the Skógar tephra is different from the traditional interpretation [38].