Abridged English version
As early as 2001, the Intergovernmental Panel on Climate Change (IPCC) has pointed out that glaciers and ice caps are key variables of ongoing climate change [12]. Mountain glaciers are considered to constitute indicators for climate warming over the 20th century [15,18]. Historians have early emphasised the interest of a global study of climate and of its impact on human beings. They deduced from paintings, etchings, and archives successive glacier surges and retreats [24]. Observational strategies make abundant use of new technologies such as remote sensing, which is relevant to bridge the gap in scale, logistics, and resolution between detailed process studies at a few well-studied sites and the global coverage [5,16,21,27,28,30].
Basically, four parameters allow us to quantify the fluctuation of the glaciers:
- • the location of the snout of the glacial tongue, this parameter being applicable only for large valley glaciers such as the Aletschgletscher in Switzerland, where the retreat reaches 2 200 m in about 100 years [14,40];
- • the surface of the glacier, the evolution of which depends mainly on the thickness of the ice cap, its elevation and its orientation;
- • the mass balance, i.e. the input or output of water per year. This parameter deeply depends on the precipitation rate and is not necessarily a more precise indicator of climate change than the glacier's length is [12];
- • the Equilibrium Line Altitude (ELA), which is the physical limit between ablation and accumulation. The accuracy of mass balance and ELA's location depends on climate data such as temperature or snow precipitation. ELA could be estimated using empirical methods such as THAR (Terminus-to-Head Ratio) [25]. According to previous works [4,23,29,35], the value of 0.5 has been utilized. Time-series of mass balance do contain year-to-year variability reflecting short-term fluctuation in meteorological quantities, but the effect of changing glacier geometry is of concern on longer time scales. The total quantity of water remains frequently unknown with precision because of the lack of knowledge concerning the topography of the base of the glacier. The albedo plays also a particular part, and it can be indirectly given based on climatic data.
Whereas fluctuations of large alpine glaciers are deeply illustrated [14,34,40], a few data concern smaller mountain glaciers [10]. Previous works have emphasised the reactivity of the mountain glacier at any climate change, either temperatures or precipitations. The Rocher Blanc (2928 m a.s.l.), located at 45°14′N and 6°6′E, is one of the main peaks in the northern part of the Belledonne Massif (Fig. 1 and Table 1). The area is built with metamorphic rocks dominating the Sept-Laux granitic plateau. Belledonne is the westernmost glacier area in the Alps, i.e. the most oceanic from a climate point of view. No regular climate, snow, or firn measurements are made. The closer selected sites with detailed studies are the Saint-Sorlin Glacier and Sarennes Glacier, in the neighbouring Grandes Rousses Massif [36,37]. The major glacier is the Rocher Blanc one, with a typical niche-shape. The other glaciers constitute compound or single basins usually edged by accumulations at their base.
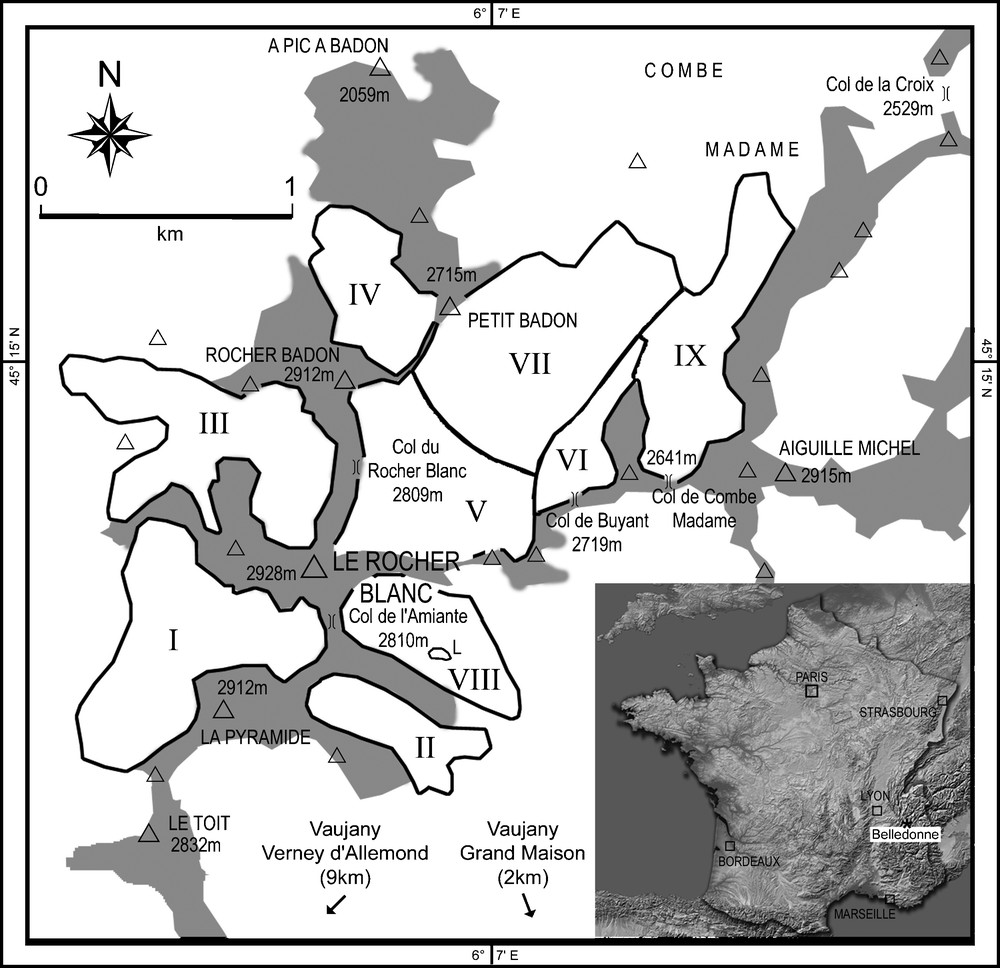
Les glaciers du Rocher Blanc (Belledonne). I à IX. Aire maximale des glaciers décrits Tableau 1. L (glacier no VIII), lac glaciaire temporaire. Grisés : principales lignes de crêtes. Triangles : sommets.
Fig. 1. The glaciers of the Rocher Blanc (Belledonne). I to IX. Maximal area of each glacier described in Table 1. L (glacier No. VIII) corresponds to a temporary glacial lake. Grey: major crest lines. Triangles: summits.
Aerial photographs have been used (Table 2). Aerial oblique views taken by the French Army acquired in September 1926 illustrate the early setting of the glaciers from the western flank of the Rocher Blanc massif. Nine campaigns by the ‘Institut géographique national’, the so-called IGN, have been exploited, respectively from 1948, 1956, 1980, 1981, 1993, 1996, 1998 (two acquisitions), and 2003. Only campaigns carried out in July or August have been selected. Moreover, satellite data have been used, respectively a Landsat-MSS (Multi Spectral Scanner) scene acquired in July 1975 and a Landsat-TM (Thematic Mapper) scene acquired in August 1992. All these data have been geometrically corrected and orthorectified. A database has been built, where the elementary pixel represents 20 m2. For years 1926 and 1993, the total surface or grand total (Table 2) results from an extrapolation, based on the basic hypothesis that the surfaces of the Rocher Badon and Petit Badon glaciers are well correlated with the total surface of the area (R2 = 0.98). Regular field missions are carried out for in-situ observations and GPS measurements.
The retreat or surge of mountain glaciers results from a balance between winter accumulation and summer ablation. The sensitivity of glacier mass balance to climate change is widely recognized. These fluctuations depend on the elevation, whereas the sensitivity to temperature decreases with the altitude. For the Rocher Blanc Massif, the glacier surface evolution has been compared to climate parameters (Fig. 2). Mean summer daily temperatures (April to September) and winter precipitations (October to March) have been analyzed on the period 1948–2006. The two meteorological stations used are located at Vaujany, only a few kilometres from the Rocher Blanc. Data have been complemented by those from the Lyon-Bron meteorological station for the very last years. This station may appear far from the Belledonne glaciers, but temperature fluctuations have been shown to be well correlated over large areas [6,36]. Accurate balance determination needs the knowledge of local snow precipitations [31,38,39]. Such data are not available for the area under investigation.
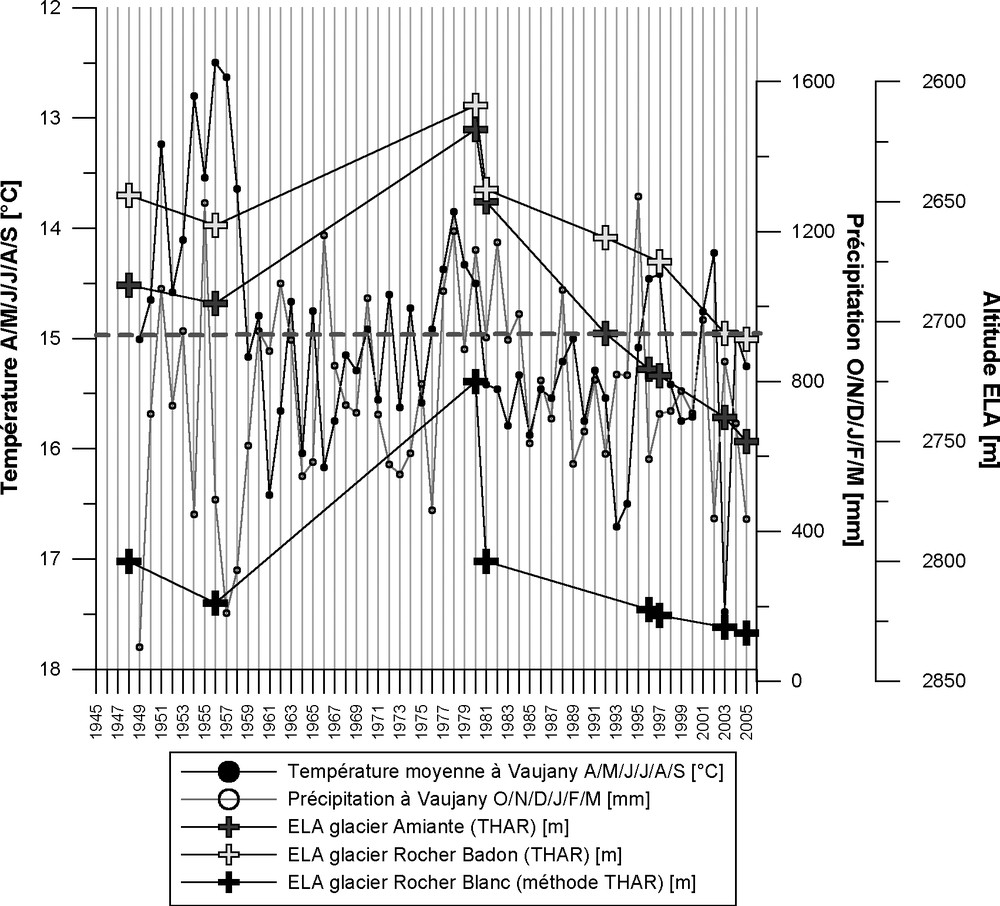
Relation entre la surface des glaciers, l’ELA de trois glaciers et les paramètres météorologiques (pluviométrie et température journalière moyenne). Les températures et l’ELA ont des valeurs croissantes vers le bas, alors que les précipitations ont des valeurs croissantes vers le haut. Ainsi, au-dessus du tireté horizontal, les conditions sont favorables au développement du glacier ; au-dessous, elles sont défavorables.
Fig. 2. Relation between glacier surface, ELAs, and meteorological parameters (precipitation and mean daily temperature). For temperatures and ELAs, values are increasing downwards. For precipitation, values are increasing upwards. Thus, above the dashed line, climate conditions are favourable to the development of the glaciers.
In 1926, the glaciers appear as extended as in 1981. The late 1920s are known as the last maximal surge for the 20th century in the Mont Blanc massif [31,38], and in Switzerland. Between 1948 and 1956, half the surface of the glaciers is retreating. The mean daily temperature decrease reaches about 1 °C. Therefore, in spite of a relatively favourable period between 1949 and 1954, the glaciers shrink, due to an insufficient accumulation. Then, between 1956 and 1981, the glacier surface increases with a maximum in 1980. During that period, precipitations and temperatures are relatively stable. Winter accumulation strongly increases in 1975–1980. 1978 and 1980 are cold years. Precipitations mainly explain the 1980 surge. March and April 1981 are warm, whereas May to July is a rainy period. A lack of winter accumulation followed by an important spring melting explains the retreat of about a quarter of the glacier surface between 1980 and 1981. The large 1981 retreat slowly continues until the early 1990s. It is abruptly interrupted in 1993, which is a very cold and humid year. Abundant precipitations during the autumn 1992 make punctually the glaciers greater. From 1993 onward, the retreat prevails, notably during the warm year 1994. The year 1997 is the first of a series of very warm years, especially in summer, and particularly in August. Summers become warmer and longer. The comparison of July and August 1998 illustrates high temperatures during mid-August. High albedo and temperatures explain the retreat of the glacier. At the end of the famous heat wave of 2003 [33], the glacier surface is only 10% of that measured in 1980. Unusually high temperatures are registered in June and August 2003. Since 2003, the retreat is confirmed. Field data from August 2005 indicate a retreat of about 60% by reference to 2003. This shrinkage is particularly obvious at the base of the Rocher Blanc glacier and within the Rocher Badon glacier. It has been confirmed by the last field campaign carried out in August 2006, the shrinkage being accelerated by the very high temperatures observed in July 2006. During the 1980–2004 period, the temperature increases by about 1.8 °C. This trend is not homogenous. Detailed studies have shown that the minimal temperature increase is greater than that of the maximal temperature [9,11,20,22,25,32].
Some glaciers are currently surging, for example in Tibet [8], New Zealand, and Norway [34]. Nevertheless, the prevailing trend is a large retreat of glaciers, particularly for the small mountain glaciers [7,13,17,27]. Whereas the continued shrinking of mountain glaciers has also become obvious in the Alps [1–3,40], detailed observations of small mountain glaciers of the Belledonne Massif show quantitative fluctuations at the century time-scale. Local surges have been emphasised in the late 1920s and around 1980.
1 Introduction
Réaffirmée en 2007, la sensibilité des glaciers de montagne au climat a été reconnue dès 2001 par l’IPCC (Intergovernmental Panel on Climate Change) comme l’un des paramètres principaux à suivre pour étudier les changements climatiques globaux [15,18]. Les historiens avaient déjà souligné l’importance de ces fluctuations et leurs conséquences sur les populations humaines [24]. Mais, si des variations qualitatives peuvent être argumentées à partir des anciens documents, notamment des gravures et des peintures, il est plus difficile de quantifier les modifications de dimension des glaciers, surtout pour les glaciers locaux de montagne d’extension limitée.
Fondamentalement, quatre paramètres sont indicatifs de l’évolution d’un glacier :
- • la position du terminus de la langue glaciaire, ce paramètre ne s’appliquant par définition qu’aux glaciers de vallée, dont les variations considérables ont été illustrées sur le glacier d’Aletsch en Suisse – 2200 m de retrait entre 1900 et 2000 [14,40] – ou, avec une amplitude plus modeste, sur le glacier des Bossons (massif du Mont-Blanc) – 600 m de retrait entre 1900 et 2000 [31,40] – ;
- • la surface du glacier, dont l’évolution dépend de l’épaisseur de glace mise en jeu, de l’altitude et de l’orientation ;
- • l’altitude de la ligne d’équilibre, ou ELA (Equilibrium Line Altitude), qui marque pour chaque saison la limite physique entre deux processus : l’alimentation liée aux précipitations au-dessus de l’ELA, l’ablation due à la fonte et la sublimation au-dessous ;
- • enfin, le bilan de masse, c’est-à-dire la perte ou le gain en eau par cycle annuel.
Contrairement à une opinion répandue, le group I de l’IPCC a indiqué que le bilan de masse – qui intègre une forte variabilité interannuelle – ne serait pas nécessairement plus précis que la surface des glaciers en tant que marqueur des variations du climat [12]. Les variations de surface seraient d’ordre décennal, alors que les variations de longueur des langues glaciaires seraient d’ordre centennal [19]. Les principaux glaciers suivis dans les Alpes sont de grands glaciers, pour lesquels des bilans de masse peuvent être calculés [39], ce qui n’est pas le cas des glaciers locaux. En revanche, si le bilan de masse nécessite la connaissance des niveaux de neige, des précipitations, des températures, etc., l’ELA peut être évaluée par des méthodes empiriques, telles que THAR (Terminus-to-Head Ratio) [26]. Cette méthode considère que la position de l’ELA peut être approchée en utilisant un rapport entre les altitudes du front glaciaire et du sommet du glacier. Selon les régions du globe, ce rapport empirique s’échelonne entre 0,3 et 0,55. Pour les glaciers de montagne, des valeurs de 0,4 à 0,5 [4,35], voire 0,55 [23], ont été proposées dans différents contextes. Dans la suite de l’article, la valeur de 0,5 est utilisée, conformément à la moyenne la plus fréquemment retenue (par exemple, [29]). La présente étude porte sur l’analyse des surfaces de glaciers locaux de montagne sur les 80 dernières années.
2 La zone d’étude
Le rocher Blanc (2928 m) est l’un des principaux sommets au nord du massif de Belledonne (45°14′N, 6°6′E), aux confins des départements de l’Isère et de la Savoie. Il est constitué, pour l’essentiel, de gneiss et d’amphibolites appartenant au rameau interne de Belledonne, dominant de près de 800 m le plateau granitique des Sept-Laux. Belledonne est la chaîne la plus occidentale des Alpes, et donc la plus soumise aux influences océaniques. Le glacier majeur du massif du Rocher-Blanc se développe au nord-est ; il est de type niche (Fig. 1 et Tableau 1). Les autres glaciers locaux sont principalement des bassins composés ou des bassins simples, plutôt orientés au nord-ouest ou au nord-est. De nombreux névés isolés jalonnent la partie inférieure des glaciers, surtout ceux exposés à l’ouest. Les morphologies sont typiques de glaciers chauds, avec la présence de stries glaciaires sur le substratum rocheux, de roches moutonnées et de bourrelets morainiques.
Caractéristiques topographiques des glaciers du massif du Rocher-Blanc. Types de glaciers : COB = bassin composé, NIC = niche, SB = bassin simple. Nos. I à IX, voir Fig. 1
Table 1 List of the glaciers of the Rocher Blanc with their topographic characteristics. Glacier types: COB = compound basin, NIC = niche, SB = single basin. Nos. I to IX, see Fig. 1
Localisation (latitude et longitude) | Surface (ha) | Élévation max. (m) | Élévation min. | Exposition (m) | Type | Pente moyenne | |
I. Amiante | 45°13′33″N | 42,0 | 2800 | 2480 | WNW | COB | 22° |
6°5′38″E | |||||||
II. S Amiante | 45°13′12″N | 11,8 | 2750 | 2430 | SE | SB | 26° |
6°6′32″E | |||||||
III. Rocher Badon | 45°14′46″N | 41,1 | 2800 | 2330 | NW | COB | 23° |
6°6′16″E | |||||||
IV. Petit Badon | 45°15′4″N | 18,6 | 2700 | 2290 | NNW | COB | 32° |
6°6′35″E | |||||||
V. Rocher Blanc | 45°14′36″N | 33,2 | 2900 | 2700 | NE | NIC | 17° |
6°6′41″E | |||||||
VI. Buyant | 45°14′45″N | 12,3 | 2710 | 2430 | NNE | COB | 19° |
6°7′8″E | |||||||
VII. Nord rocher Blanc | 45°14′52″N | 55,2 | 2720 | 2360 | NNE | SB | 20° |
6°6′58″E | |||||||
VIII. Sud rocher Blanc | 45°14′21″N | 19,7 | 2780 | 2520 | SE | SB | 18° |
6°6′41″E | |||||||
IX. Combe Madame | 45°14′57″N | 31,0 | 2640 | 2210 | NE | COB | 20° |
6°7′32″E |
3 Matériel et méthodes
Les techniques de la télédétection aérospatiale [5,16,21,27,28,30] permettent de reconstituer, au moins pour les dernières décennies, l’évolution des aires englacées de zones non instrumentées, ce qui est le cas général des glaciers locaux de montagne des Alpes occidentales françaises. Les plus proches glaciers suivis régulièrement sont ceux de Saint-Sorlin [36] et de Sarennes [37], respectivement situés au nord et au sud du massif des Grandes-Rousses, à une quinzaine de kilomètres au sud-est de Belledonne.
Des anciens documents photographiques ont été consultés, intégrant des photographies prises par des guides de haute montagne dans les années 1910–1920 et des vues aériennes militaires obliques de septembre 1926, montrant l’allure générale des glaciers du flanc ouest du rocher Blanc (Petit Badon, rocher Badon et Amiante). Parmi les campagnes de l’Institut géographique national, toutes consultées, neuf campagnes ont été retenues : 1948, 1956, 1980, 1981, 1993, 1996, 1998 (deux dates) et 2003. Certaines campagnes des années 1960–1980 et de 2001 n’ont pas été exploitées, car elles ont été acquises, soit en dehors de la période juillet–août (campagne aérienne du 25 mai 1982 par exemple), soit après de fortes chutes de neige (cas des photographies du 22 juillet 2001). Les émulsions sont panchromatiques ou en couleur. Une scène satellitale Landsat Multi Spectral Scanner (MSS), acquise en juillet 1975, et une scène Landsat Thematic Mapper (TM), acquise en août 1992, ont également été utilisées. Une difficulté dans l’analyse des images spatiales est représentée par les zones d’ombre, souvent plus saturées que sur les photographies aériennes, ce qui ne permet pas de suivre précisément les névés dans les zones hautes ou sous des aplombs.
Les photographies aériennes ont été orthorectifiées. Le résultat est un peu moins précis pour les données de 1948 et 1956, car les paramètres d’acquisition des missions n’ont pas pu être tous identifiés. Ainsi, une base de données a été constituée, permettant de comparer l’extension des glaces aux différentes dates pour les neuf bassins du massif du Rocher-Blanc. Les surfaces ont été mesurées avec une précision de 20 m2 (taille du pixel dans la base), puis additionnées pour aboutir à la couverture totale des glaciers (Tableau 2). Pour les années 1926 et 1993, la surface totale résulte d’une extrapolation des résultats partiels des glaciers du rocher Badon et du Petit Badon. Cette extrapolation est possible, dans la mesure où la surface de ces deux glaciers (Total III–IV only, Tableau 2) est très fortement corrélée à la surface totale (grand total), avec un coefficient de détermination R2 = 0,98. Depuis 2002, les glaciers du massif du Rocher-Blanc ont fait l’objet de visites sur le terrain, destinées notamment à des mesures par GPS des surfaces des principaux glaciers.
Évolution des surfaces des glaciers du massif du Rocher-Blanc, de 1926 à 2006
Table 2 Surface of the glaciers of the Rocher Blanc between 1926 and 2006
Surface (ha) | 1926 | 1948 | 1956 | 1975 | 1980 | 1981 | 1992 | 1993 | 1996 | 1998 | 1998 | 2003 | 2005 | 2006 | |
sept. | 26/08, | 13/08, | 14/07, | 17/07, | 27/08, | 05/08, | 28/07, | 17/08, | 30/07, | 30/08, | 02/08, | août | août | ||
I. Amiante | 42,0 | 22,2 | 18,6 | 10,9 | 27,8 | 33,1 | 25,3 | 8,0 | ** | 7,2 | 9,0 | 8,2 | 2,7 | 2,5 | 1,5 |
II. S Amiante | 11,8 | ** | 3,9 | 2,5 | 11,4 | 11,7 | 10,5 | 4,1 | ** | 2,6 | 3,0 | 0,6 | 0,9 | 0,3 | ** |
III. Rocher Badon | 41,1 | 21,4 | 18,9 | 11,1 | 20,3 | 38,6 | 24,1 | 9,0 | 21,1 | 7,4 | 8,4 | 2,8 | 5,7 | 3,5 | 2,5 |
IV. Petit Badon | 18,6 | 13,7 | 8,4 | 3,5 | 11,1 | 15,4 | 15,6 | 4,0 | 13,8 | 1,0 | 4,5 | 1,5 | 0,4 | 0,3 | ** |
V. Rocher Blanc | 33,2 | ** | 28,7 | 11,6 | 29,6 | 32,7 | 29,5 | 24,7 | ** | 19,5 | 16,3 | 9,1 | 7,0 | 4,5 | 3,3 |
VI. Col de Buyant | 12,3 | ** | 9,2 | 6,1 | 10,4 | 11,3 | 11,0 | 7,3 | ** | 8,3 | 7,0 | 4,5 | 1,2 | 0,8 | 0,8 |
VII. Nord rocher Blanc | 55,2 | ** | 21,8 | 6,8 | 47,6 | 51,2 | 30,5 | 3,6 | ** | 3,0 | 3,5 | 0,4 | 0,8 | 0 | 0 |
VIII. Sud rocher Blanc | 19,7 | ** | 17,7 | 6,3 | 19,7 | 19,5 | 18,6 | 7,1 | ** | 5,1 | 4,8 | 1,1 | 1,5 | 1,0 | ** |
IX. Combe Madame | 31,0 | ** | 22,0 | 11,1 | 19,7 | 29,5 | 23,9 | 12,1 | ** | 9,9 | 11,2 | 8,0 | 3,8 | 3,0 | 2,7 |
Grand total | 264,9 | 169,6* | 149,2 | 69,9 | 197,6 | 243,0 | 189,0 | 79,9 | 168,7* | 64,0 | 67,7 | 36,2 | 24,0 | 14,1 | ≈12 |
% de la surface | 64,0 | 56,3 | 26,4 | 74,6 | 91,7 | 71,4 | 30,2 | 63,7 | 24,2 | 25,6 | 13,7 | 9,1 | 5,3 | 4,5 | |
Total III–IV seulement | 59,7 | 35,1 | 27,3 | 14,6 | 31,4 | 54,0 | 39,7 | 13,0 | 34,9 | 8,4 | 12,9 | 4,3 | 6,1 | 3,8 | ≈3,6 |
% des surfaces III–IV | 58,8 | 45,7 | 24,5 | 52,6 | 90,5 | 66,5 | 21,8 | 58,5 | 14,1 | 21,6 | 7,2 | 10,2 | 6,4 |
Les précipitations hivernales en altitude peuvent être jusqu’à trois fois plus importantes que celles enregistrées dans les vallées [36]. Il est donc difficile d’extrapoler des données de pluviométrie mesurées dans les vallées, ce qui nous a poussés à rechercher les données météorologiques les plus proches. En revanche, à l’échelle des Alpes occidentales, les variations de température semblent corrélées sur de grandes distances [6]. Un suivi régulier de l’enneigement de certains massifs, notamment celui du Mont-Blanc, a montré l’importance des facteurs locaux, qui nécessitent, dans l’absolu, la connaissance précise de chaque secteur, en fonction de son orientation, connaissance souvent inaccessible, même pour les grands glaciers. Les résultats du suivi des glaciers du Rocher Blanc ont été confrontés aux données journalières de température moyenne (Tmax + Tmin/2) et de précipitations sur la période 1948–2006 (Fig. 2). Deux postes météorologiques ont été utilisés. Ils sont situés sur la commune de Vaujany, respectivement au Verney-d’Allemond (45°09′00″N, 06°02′42″E, 772 m) et au barrage hydroélectrique de Grand-Maison (45°09′24″N, 06°04′36″, 1520 m), ce dernier étant à seulement 3 km du sommet du rocher Blanc (Fig. 1). Les données de température de Lyon–Bron ont été utilisées – en appliquant un coefficient altitudinal de 6 °C/km, comme pour l’étude du glacier de Saint-Sorlin [36] – pour compléter la chronique sur la période 2001–2006 non acquise à Vaujany.
4 Interprétation
Deux paramètres physiques agissent sur le volume des glaciers locaux de montagne. Le premier est l’ablation liée à la fonte des névés, principalement de juin à septembre, et à la sublimation. Le second est l’alimentation par les précipitations neigeuses qui ont lieu, pour les glaciers tempérés, d’octobre à mars. La prépondérance de l’un ou de l’autre de ces deux paramètres entraîne le retrait ou la progression du glacier et, par conséquent, nous considérons que la position et l’extension glaciaire sont pilotées par les conditions climatiques. Ainsi, les données climatiques de Vaujany ont été semestrialisées : les précipitations correspondent au total des précipitations des mois d’octobre à mars, tandis que les températures correspondent à la moyenne des mois d’avril à septembre. Sur la Fig. 2, le tireté horizontal correspond à la valeur moyenne des précipitations et des températures semestrielles. La partie haute du graphique indique des conditions météorologiques favorables à la croissance du glacier, alors que la partie basse indique des conditions défavorables. Par la suite, les notions de déficit ou d’excédent sont exprimées par rapport aux valeurs moyennes.
Aucune donnée précise d’écoulement glaciaire, paramètre important pour les grands glaciers, n’a pu être évaluée pour les glaciers du massif du Rocher-Blanc.
4.1 Avant 1948
Les aires de glacier pour les secteurs du versant occidental du massif du Rocher-Blanc, en fin d’été 1926, sont proches de celles mesurées en 1981. Des photographies de terrain acquises en juillet 1920 montrent déjà un glacier du Rocher-Blanc bien développé, avec une épaisseur de glace beaucoup plus importante qu’actuellement, comme en témoigne l’ennoiement de nombreux pics et crêtes rocheux, qui vont progressivement apparaître au cours du xxe siècle. La fin des années 1920 est connue pour l’avancée de certains glaciers, notamment en Suisse [1–3] ; elle correspond à l’extension maximale des langues glaciaires des glaciers du Mont-Blanc au xxe siècle [19,31,38].
4.2 1948–1956 : un recul
La chronique climatique débute l’année de la première acquisition aérienne par l’IGN (1948), après quelques années réputées froides (1941–1946) [9]. La période 1948–1956 est caractérisée par une baisse moyenne des températures estivales d’environ 1,5 °C. Dans le même temps, le régime des précipitations hivernales est irrégulier, des déficits importants étant observés en 1948 et 1956. Les années 1955 et 1956 – cette dernière étant connue pour ses froids intenses à l’échelle de l’Europe de l’Ouest – ont été les deux plus froides sur la période considérée (1948–2006). Le retrait des surfaces des glaciers du Rocher-Blanc entre 1948 et 1956 est supérieur à 50 %. Malgré des années plutôt froides et une période 1949–1954 a priori favorable au développement des glaciers, ce retrait a été favorisé par des précipitations insuffisantes et mal réparties.
4.3 1956–1981 : une progression culminant en 1980
Les températures estivales sont stables en moyenne sur la période 1956–1981, mais avec une forte variabilité, comme en témoignent les années 1955 à 1960, marquées par une hausse importante. De 1961 à 1977, les températures sont globalement proches de la moyenne, mais elles sont très basses en 1962 et 1971. La période 1976–1979 est favorable au développement des glaciers, avec des hivers neigeux (surtout en 1977 et 1979) et des températures estivales fraîches (surtout en 1977). Ces éléments expliquent l’extension des glaciers de montagne, qui atteint son paroxysme en 1980. Le faible enneigement hivernal et la fonte importante au printemps sont la cause du retrait brutal observé entre les étés 1980 et 1981, soit environ 25 % de surface en moins.
4.4 1981–1993 : le début du retrait
Le retrait observé en 1981 se poursuit lentement jusqu’au début des années 1990, avec une période 1984–1991 particulièrement défavorable aux glaciers. La progression ponctuelle des glaciers constatée à l’été 1993 semble liée à de très fortes précipitations neigeuses en octobre (les plus fortes précipitations pour un mois d’octobre sur la période 1948–2006, avec 346 mm) et novembre 1992. Malgré des températures estivales chaudes, les précipitations abondantes réalimentent temporairement les glaciers, un peu à l’image de l’alimentation actuelle des glaciers maritimes de Nouvelle-Zélande ou de Scandinavie, qui croissent malgré des températures en augmentation [12].
L’évolution des glaciers du massif du Rocher-Blanc s’inscrit dans un mouvement de retrait général, interrompu en 1993 au prix de conditions très particulières, qui illustrent la sensibilité des glaciers locaux de montagne aux moindres variations climatiques. Même si 1993 représente un doublement de la surface des glaciers par rapport à l’année précédente, il ne s’agit que d’environ 70 % de la surface observée en 1980.
4.5 1993–2006 : un retrait historique
Entre 1993 et 1996, le recul des surfaces de glaciers est net. Le glacier de Buyant s’individualise à la suite de l’émergence d’une crête de métapyroxénites, qui sépare en deux le grand glacier du rocher Blanc, connu depuis la fin du xixe siècle. Alors que les surfaces respectives des glaciers en 1956 et 1996 sont très proches, la crête rocheuse n’apparaît pas en 1956, l’épaisseur du glacier étant au moins de 15 m de plus. Les températures sont chaudes durant l’été 1994. Ainsi, malgré un hiver 1994–1995 qui est le plus humide sur la période 1948–2006, le recul des glaciers s’accélère.
L’année 1997 inaugure une période de trois années successives à été chaud, marquée par des mois d’août très supérieurs à la moyenne et des températures en août plus élevées qu’en juillet dans six cas sur dix entre 1997 et 2006 (seulement 17 fois sur 49 durant la période 1948–1996). Depuis 1997, les étés chauds sont donc aussi plus longs. Les photographies des 30 juillet et 30 août 1998 montrent un net retrait sur un mois. Cela est imputable à des conditions exceptionnelles d’ensoleillement et à des températures très élevées entre le 8 et le 18 août. De plus, ces conditions succèdent à une période de mai à juillet déjà chaude. Le faible albédo, lié à des chutes de neige peu importantes, et les températures élevées sont donc les causes conjointes d’une diminution importante de la surface des glaciers au cours de l’été 1998.
La canicule de l’été 2003, dont les effets régionaux ont été décrits par ailleurs [33], a eu un effet très important sur les glaciers du massif du Rocher-Blanc. En septembre 2003, ils n’occupent plus que 10 % de la surface observée lors du maximum de 1980. La période s’écoulant de novembre 2002 à août 2003 est anormalement chaude. Elle culmine en juin. Après un mois de juillet chaud, mais non exceptionnel, le mois d’août est à son tour extrêmement chaud, avec des moyennes journalières supérieures à 30 °C du 5 au 13. Le 11 août, au maximum de la canicule, les températures maximales ont été de 41 °C à Grenoble (donnée Météo France), de 32 °C à 1000 m et de 24 °C à 2800 m sur le massif de Belledonne (col de l’Amiante, données personnelles). Depuis 2003, cette tendance se confirme : les données recueillies sur le terrain durant l’été 2005 indiquent un retrait de 60 % par rapport à 2003, surtout sensible pour la base du rocher Blanc et le rocher Badon, qui étaient en 2003 les deux plus vastes aires englacées. Les données de 2006 marquent un recul sensible – par rapport à 2005 – pour les glaciers de l’Amiante, du rocher Badon et du rocher Blanc. Ce recul est moins sensible pour les autres glaciers situés à des altitudes plus basses. L’année 2006 est néanmoins l’année la plus humide depuis une décennie.
L’évolution d’un petit lac glaciaire apparu en 1996 dans le bassin du Sud rocher Blanc (L, Fig. 1) montre que la surface d’eau libre est respectivement de 320 m2 (1996), 740 m2 (juillet 1998), 3600 m2 (août 1998), 3400 m2 (2003). Ces données confirment les conditions très chaudes d’août 1998, la décrue en 2003 pouvant être interprétée comme la conséquence d’un tarissement des névés disponibles en amont.
L’analyse de l’évolution des glaciers du rocher Blanc de 1926 à 2006 montre l’importance de plusieurs facteurs, tels que :
- • l’altitude, car l’ablation est plus forte aux basses altitudes, ce qui est bien illustré sur les glaciers de la combe Madame et surtout du Nord rocher Blanc, qui a disparu en 2005 ;
- • l’orientation, les versants exposés au sud réagissant différemment des autres, comme on le voit pour les glaciers du Sud Amiante et du Sud rocher Blanc, peu affectés par la diminution entre 1980 et 1981, essentiellement liée à une baisse des précipitations, mais très affectés par les fortes chaleurs de 2003 et 2005 ; a contrario, durant la même période, le glacier du Buyant, orienté vers le NNE, fond moins vite ;
- • le volume mis en jeu : les petites surfaces associées à des faibles volumes, par exemple les glaciers de l’Amiante, du rocher Badon et du Petit Badon après 1998, disparaissent plus rapidement que le glacier du rocher Blanc, plus élevé et beaucoup plus compact. En revanche, la pente calculée pour chaque glacier, et qui varie de 17 à 32° (Tableau 1), ne semble pas devoir être un paramètre déterminant.
Entre 1980 et 2004, l’augmentation de la température moyenne mensuelle est de 1,8 °C environ. Elle s’accompagne d’une remontée plus importante des minimales que des maximales, phénomène observé à l’échelle de la France [22,32]. Dessens et Bücher ont même noté sur la station pyrénéenne du pic du Midi (2862 m), située à une altitude proche de celle du rocher Blanc, une hausse de 2,5 °C des températures minimales sur un siècle, alors que les températures maximales baissaient de 0,5 °C [9]. Cette tendance est confirmée par les données météorologiques de Vaujany. Sur la période 1959–2000, la moyenne des températures minimales (période avril à septembre) a augmenté de 0,77 °C alors que la moyenne des températures maximales a diminué dans le même temps de 0,43 °C.
5 Synthèse et conclusion
L’analyse des données de télédétection aérospatiale sur le massif du rocher Blanc (Belledonne) montre que les glaciers locaux de montagne, moins suivis et instrumentés que certains grands glaciers alpins, sont des enregistreurs fidèles des variations climatiques. La faiblesse des volumes et des surfaces mis en jeu explique une moindre inertie, traduite du point de vue cartographique par une réaction plus rapide que les grands glaciers et une alternance de retraits (fin des années 1950 et depuis 1994) et progressions (événements des années 1920, 1980 et 1993). La confrontation aux données météorologiques les plus proches (stations de Vaujany) sur la période 1948–2006 indique que, si le volume des précipitations joue un rôle dominant jusqu’en 1980, c’est l’augmentation des températures qui est le facteur prépondérant pour les dernières années. La période exceptionnellement chaude d’avril à août 2003 a favorisé un retrait général, vérifié sur le terrain et confirmé jusqu’en août 2006.
L’évaluation de l’ELA par la méthode THAR a été réalisée pour trois des glaciers étudiés : rocher Blanc, Amiante, rocher Badon (Fig. 2). Les glaciers du rocher Badon et de l’Amiante présentent des variations très proches, tandis que celui du rocher Blanc présente toujours une ELA supérieure. Du fait de l’effet de fœhn, le rocher Blanc reçoit moins de précipitations que les deux autres secteurs, orientés vers l’ouest. La surface des glaciers et l’ELA apparaissent ainsi fortement corrélés avec les variations des paramètres météorologiques. Une perspective consistera à étudier en détail la morphologique du glacier du rocher Blanc, afin d’en estimer le bilan de masse.
Si les glaciers alpins sont en régression quasi générale [1–3], certains glaciers montrent, à l’échelle planétaire, une tendance récente à l’avancée, comme dans le Sud de l’Alaska, en Sibérie méridionale [10], dans l’île du Sud de la Nouvelle-Zélande, en Norvège, en relation avec l’oscillation nord-atlantique [34], ou encore dans la chaîne tibétaine du Kunlun [8]. Dans le cas du glacier d’Aletsch (Alpes suisses), le retrait actuel n’atteindrait pas ceux enregistrés au viiie et xve siècles [14]. La découverte de tourbe sous les glaces actuelles semble même indiquer une absence de glaciers dans les Alpes, ou au moins dans certains massifs, il y a 7000 ans. Dans les grands massifs montagneux, tels que les Alpes, l’Himalaya ou les Andes, le recul des glaciers est un phénomène général et la perte de masse est souvent considérable, phénomène toujours accentué pour les glaciers de taille réduite, comme l’illustre le Tien Shan [27]. Entre 2002 et 2003, sur les 111 glaciers suivis sur l’ensemble du territoire helvétique – intégrant de grands glaciers et des glaciers locaux de montagne –, 97 étaient en retrait de 2 à 157 m, un seul en progression, du reste infime (2 m), les 13 autres ayant été non observés, ou la valeur de leur terminus non déterminée [2]. Dans les Alpes italiennes et autrichiennes, plusieurs exemples de retrait de glaciers locaux de montagne, étudiés à partir des données de télédétection, ont été récemment illustrés [28]. Dans certains secteurs, le recul est tel que la plupart des glaciers de montagne disparaissent, comme dans le Glacier National Park au Montana [17] ou dans l’état du Washington [13], dans le Nord-Ouest des États-Unis d’Amérique. Là où les glaciers sont peu nombreux et de petite taille, la perte de masse et la disparition des glaciers locaux, notamment les glaciers de cirque, sont des phénomènes importants, par exemple dans les Pyrénées franco-espagnoles [7].
Qualitativement, les glaciers locaux sont d’excellents indicateurs des fluctuations du climat [10], avec une inertie de l’ordre de douze mois, alors que les glaciers plus importants réagissent à des échelles temporelles plus larges. Ces derniers peuvent aussi se fragmenter en glaciers locaux au fil de leur retrait. L’approche quantitative est plus délicate à conforter, en raison du recul limité des chroniques de mesures, tant sur le terrain que par télédétection aérospatiale. Outre les températures moyennes et les précipitations, l’amplitude thermique et, sans doute, l’albédo – qui joue un rôle fondamental sur l’ablation et le bilan de masse [37] – doivent être considérés. Il semble que l’augmentation des températures moyennes, responsables d’un recul spectaculaire des glaciers depuis 1980, soit le résultat d’une hausse des températures minimales, les températures maximales ayant tendance à stagner, voire à diminuer [11,20,25].
Remerciements
Nous remercions la photothèque de l’IGN (Saint-Mandé) pour ses conseils, Michel Gay (Grenoble) pour la communication d’informations et les discussions, ainsi que la famille Arribet de La Ferrière (Isère) pour les archives photographiques sur le massif d’Allevard. Les données météorologiques sont d’origine Météo France.
Vous devez vous connecter pour continuer.
S'authentifier