1 Introduction
Karst processes, which deeply transform the initial aquifer properties of carbonate rocks, classically result from dissolution by groundwater flowing through the matrix and/or fracture porosity. Several conditions must be fulfilled, corresponding in fact to what Mangin named ‘potential for karst development’ [19]. Groundwater has the power to dissolve carbonates, generally due to soil CO2; the relief and geometry of the aquifer formation must allow the infiltration water to flow at depth rather than on the surface, i.e. a high enough hydraulic gradient is essential to renew the water dissolving the rock and discharging the dissolved solutes contained in the groundwater [6]. However, the study of karst shows that some cave systems may have a different origin, due to the action of deep CO2 and groundwater flow driven by high-temperature gradients [4]: they are known as hydrothermal karsts, mainly from the study of caves [17].
The hydrogeological study of the Saida area, Algeria, done by K. Djidi in the framework of his doctorate thesis, shows, thanks to geochemical and isotopic tools, that the large carbonate aquifer is subject to conditions of complex karst development, classical as well as hydrothermal. These new results prompt a reconsideration of karst aquifers developing in active orogenesis conditions. The study is based on regional geological data to define the hydrogeological setting. Because of the lack of reliable hydrological data, aquifer characterisation is mainly based on means of chemical and isotopic data, collected during the 1991–1994 hydrological years by a consulting company [10] and not interpreted.
2 Objective of the hydrogeological study
Groundwater from an alluvial aquifer and mostly from carbonate aquifers is the main resource for supplying water in the Saida area. However, in recent years, the spring flow rate has decreased significantly. Moreover, the groundwater quality has deteriorated, particularly because of the use of fertilizers and infiltration of untreated wastewater. The situation has worsened with droughts that have favoured the overexploitation of the groundwater resource. Groundwater pollution and overdraft result from inappropriate management, a consequence of a lack of knowledge of the regional aquifers and their water budget. The lack of data and the obvious karstic characteristics of the aquifer make it difficult to understand their functioning and consequently their appropriate managing procedure. The hydrogeology of the Saida area was then studied to assess the groundwater resource, its storage, and the aquifer functioning and vulnerability, to finally define the best conditions of its exploitation and protection in order to lay the foundations for a sustainable development.
The present study is founded on regional geological data in order to define the hydrogeological framework. The few existing hydrological data for the 1970–1972 period are not sufficient to determine the aquifer characteristics and functioning. In fact, groundwater flow and karst development conditions can be characterised only by means of the hydrochemical and isotopic data collected during two hydrological years between 1990 and 1994 by a consulting company [10]. Our work presents the interpretation of these data, which had not been done so far.
3 Physical and geological setting
3.1 Physical and climatic framework
The study area, located 180 km southeast of Oran, covers an area of about 2500 km2, with more than 80,000 inhabitants in the town of Saida. The area is made up of the Saida plateau and on its western flank the Saida River valley oriented north–south (Fig. 1) with an average elevation of 700 m, and a maximum at 1300 m. The depression of Chott Chergui is situated to the south. The study area covers the basins of the Saida, Taria and El Abd Rivers, and the northern part of the Chott Chergui catchment area. The central part of the plateau is drained by the Rhar Amira swallow hole, where significant discharge is observed during floods. The convex shape of the plateau generates several catchments with perennial rivers, tributaries of the Saida River, which flow northwards. No perennial river flows towards the Chott Chergui.
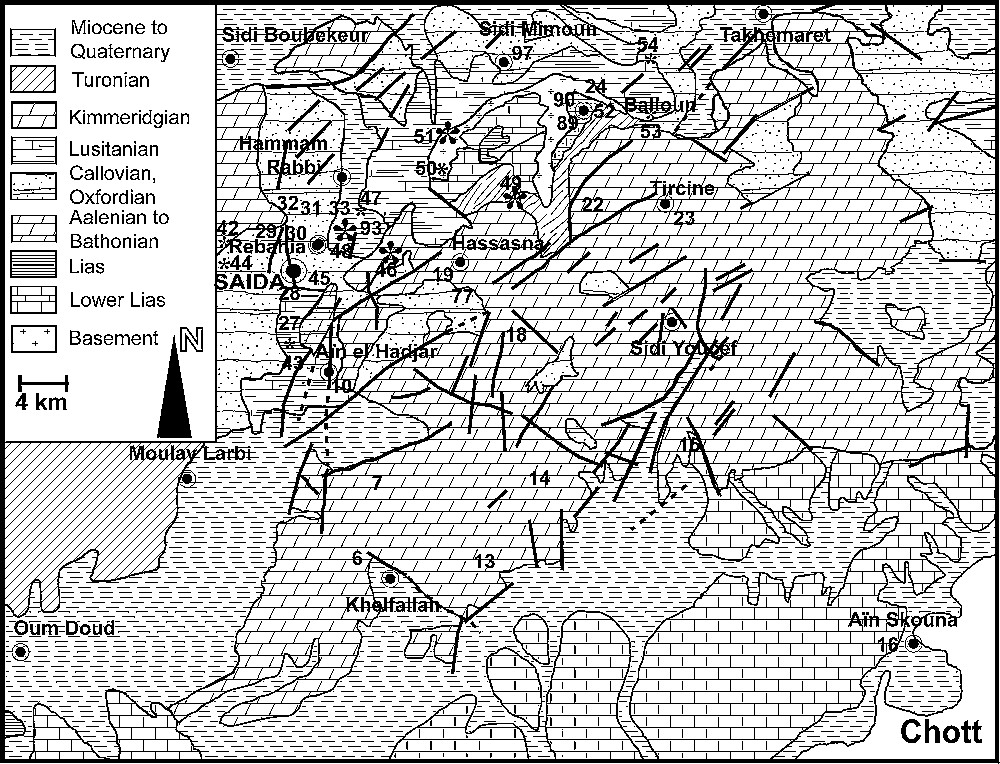
Geological map of the study area and location of sampling sites.
Fig. 1. Carte géologique du secteur étudié et localisation des sites de prélèvement.
The climate is Mediterranean, continental and semi-arid, with a 425-mm average annual rainfall (1930–1970) at Saida and Aïn El Hadjar [12]. As a transition zone between rainfall regimes of the Tell (500 mm) and high plateaus (270 mm), the region is subject to a high spatial and temporal variability. In the last two decades (1980–2000), the average rainfall has decreased from 425 mm/yr to 360 mm/yr at Saida.
3.2 Geological and structural framework
The plateau (Fig. 1) is made up of Mesozoic and Tertiary sediments overlying the Palaeozoic basement, and composed of (i) a thin impermeable Triassic series, (ii) a 400-m-thick Jurassic carbonate series, covered with a 400-m thick detrital formation, and 100-m-thick Kimmeridgian carbonates, and (iii) Tertiary continental formations, with a conglomerate and Miocene marls.
The regional structure developed during the Oligocene. Since the Miocene, the orogenesis has consisted in a brittle deformation of carbonate formations. Numerous faults structure the area into horsts and grabens, creating two main low-lying zones: the collapse depression of the Saida River valley and the subsiding region between the tectonic axis Saida–Oum Djerane–Tiffrit and that of the djebel Sidi Youssef (Figs. 1 and 2). Two fault systems create a plexus at the centre of the plateau. The geological formations plunge northwards and southwards from the djebel Sidi Youssef axis. During the uplift, the erosion of the ridge produced thick sedimentary deposits in grabens and basins.
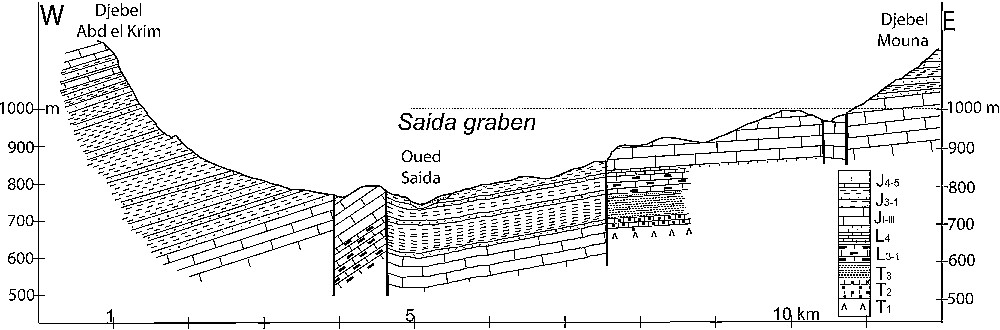
Geological cross section showing the graben structure of the Saida River valley.
Fig. 2. Coupe géologique transversale montrant la structure en graben de la vallée fluviatile de Saïda.
3.3 Hydrogeological consequences
Outcropping on 40% of the area, the Jurassic carbonate series form the main regional aquifer. It lies on an impermeable basement hardrock and Triassic clays. Its thickness of some hundred metres across its regional extent allows a hydraulic continuity, which is scarcely disrupted by faults. Its impermeable, thick cover of the Saida clay formation makes it a confined aquifer in all the low zones, especially in the Saida graben (Fig. 2). It locally discharges through recent low-permeability formations. The Upper Jurassic and Lower Cretaceous sandstones may contain local aquifers, but are generally totally drained downwards to the underlying carbonate aquifer given their perched position. Tertiary and Quaternary formations, poorly permeable on the whole, may act as local aquifers recharged by upward leakage from the Jurassic aquifer.
The brittle deformation concerns the whole Jurassic carbonate formation, with faults oriented NE–SW, structuring the horsts and grabens, linked to NW–SE thrust faults. The many faults combined with fractures and cracks give a high initial permeability to the aquifer formation. Existing documents unfortunately do not allow a detailed study of the fracturing, which could help to determine its fragmentation in several more or less independent aquifer systems.
Only the Saida unit with its surrounding horsts is presented here because of its large groundwater resources and typical hydrogeological characteristics. It covers an area of about 1220 km2, among which there are 720 km2 of non-carbonate formations with surface stream flow recharging the aquifer through swallow holes.
The Saida aquifer discharges groundwater from the Jurassic carbonate at many springs, mainly in the northern part of the graben. The wide distribution of the springs can be interpreted as the result of a poor development of the karst. It is well known that when the conditions for karst development exist [2,19], a drainage or conduit system is rapidly created, organising the groundwater flow towards a single spring with a large, but seasonally variable discharge. Apart from classical surface landforms, such as the Rhar Amira swallow hole, few karstic phenomena are reported. Speleological explorations show the existence of some caves, which never reach the phreatic zone.
However, total mud losses and tool falls are systematically reported during well boring, indicating the existence of karst cavities. Moreover high pumping rates at some wells, of several tens of L/s, with very low drawdown indicate a good connectivity between deep phreatic karstic features. These inconsistencies between apparently poor and also typical karstic characteristics were then compared to geochemical and isotopic data in order to understand the origin of karst development.
4 Characterisation of the aquifer
4.1 Analysis of the functioning from spring hydrographs
Some hydrographs from the nine springs and five from the rivers, among them the Saida River at PK.50 and Pont gauging stations, were already analysed in 1973 [23]. The authors concluded that, despite the lack of accuracy, each spring or group of springs seemed to have its own flow regime, depending on the characteristics of the aquifer discharging there. In fact, it appears that only the Pont station hydrograph of the Saida River shows a recession that can be interpreted with a classical method [19]. All other data, of low quality, cannot be interpreted.
The Pont station recession follows the classical Maillet's rule. The recession coefficient (α = 0.017) is quite high, which means that the Saida aquifer discharges its stored groundwater quite rapidly. This shows that the aquifer is well karstified. The dynamic storage, calculated from the integration of the recession, is on the order of 10 millions cubic metres, which is a relatively low volume for such a large hydrogeological unit. This interpretation cannot be confirmed, because it assumes that the Saida River drains a limited portion of the aquifer, which discharges upstream from the Pont station, and that the other springs of that basin are the discharges of independent aquifers. The existing data on the geological structure of the basin are however insufficient to verify this assumption.
4.2 Analysis of the functioning from natural tracing
4.2.1 Material and methods
Rainwater, river water and groundwater were collected for chemical and isotopic content measurements. Rainwater was sampled every month from November 1991 until January 1994. Groundwater was sampled during four field surveys: October 1991; May 1992; November 1992; May 1993. Waters were sampled and analysed according to classical procedures, as described in the report [10]. Rainfall data were compared to the 1930–1996 record, in order to determine their hydrologic and climatic context. The annual rainfalls are lower than normal (420 mm). However, the 1991–1992 hydrological year, with 376 mm, was wetter than the 1992–1993 year, with 205 mm.
Around 110 sites were sampled, corresponding to 14 springs, 50 traditional wells and 40 boreholes. Most boreholes, mainly drilled in the Saida valley, pump groundwater from the Jurassic carbonate aquifer. The wells, commonly close to boreholes, with a depth of up to 20 m, allow comparisons of groundwater from shallow and deep aquifers; some wells are fed by deep groundwater through alluvial sediments, the others by shallow groundwater from Tertiary or Quaternary formations. Spring samples characterise the predominant flow conditions in the aquifer or a large part of it, while samples from boreholes and wells are often more representative of local characteristics. Groundwater from wells is mainly influenced by hydraulic connections with rivers through the alluvium, contrary to boreholes which collect deep flow.
4.3 Chemical and isotopic characteristics of rainfall
Chloride, sulphate and sodium concentrations (Table 1), mainly related to marine aerosols, show that the rain stations are organised according to north–south and west–east directions. The Ain Zerga station is at the centre, with the lowest concentrations, which increase in both directions on either side. This distribution shows that the solute origin is not only oceanic.
Main chemical and isotopic characteristics of rainwater for each station [11]. Concentrations are in mg/L
Tableau 1 Principales caractéristiques chimiques et isotopiques de l’eau de pluie pour chaque station [11]. Les concentrations sont en mg/L
Stations | P (mm) | T (°C) | z (m) | pH | C (μS/cm) | Cl | SO4 | Na | SO4/Cl | Na/Cl | Na/SO4 | δ18O ‰ vs SMOW |
Daoud | 182.6 | 16.1 | 670 | 6.72 | 315.0 | 1.09 | 0.56 | 0.61 | 0.51 | 0.56 | 1.09 | −5.08 |
PK50 | 191.5 | 18.4 | 605 | 7.10 | 492.0 | 1.23 | 0.78 | 0.89 | 0.63 | 0.72 | 1.14 | −4.86 |
S. Mimoun | 187.5 | 16.1 | 680 | 7.48 | 164.0 | 0.87 | 0.48 | 0.32 | 0.55 | 0.37 | 0.67 | −4.51 |
Aïn Zerga | 185.1 | 14.5 | 980 | 7.13 | 136.0 | 0.72 | 0.32 | 0.36 | 0.41 | 0.50 | 1.13 | −5.63 |
Tamesna | 335.5 | 13.7 | 1115 | 7.63 | 211.0 | 0.98 | 0.31 | 0.68 | 0.32 | 0.69 | 2.19 | −5.88 |
Khelfellah | 316.6 | 13.7 | 1125 | 7.36 | 206.0 | 0.85 | 0.50 | 0.53 | 0.59 | 0.62 | 1.06 | −6.19 |
Oxygen-18 contents, in monthly and yearly weighted averages, do not vary much from one station to another. However, seasonal variations are high. The highest contents are observed during July and August, the hottest and driest months. The lowest contents are measured from November to April with the minimum in February (−7.6‰). The more enriched rainfalls were collected at the furthest stations to the north. The exceptional August 1993 rainfall, with 27 mm, is not in the range of the most enriched rainfalls. Some very negative rainfalls, lower than −8.0‰ at all stations, are related to a cold, humid air mass intrusion, of oceanic polar origin. The average shows an obvious increase in 18O contents and in mineralization during the driest period, from May to October, which is partly due to partial evaporation during showers in a dry atmospheric humidity profile, which is the dominant effect in summer showers. The classical elevation effect (Fig. 3) gives a gradient of −0.25‰ /100 m, in the classical range [7,21] observed in Mediterranean regions (−0.15 to −0.30‰/100 m). The 18O content distribution in rainfall is quite simple: it decreases in both north–south and west–east directions, as does the chemical composition. This shows that the mechanisms determining the 18O contents in rainfall are the distance from the sea, the elevation, the air temperature and, to a lesser degree, the orientation of the stations, all well known effects around the Mediterranean [14].
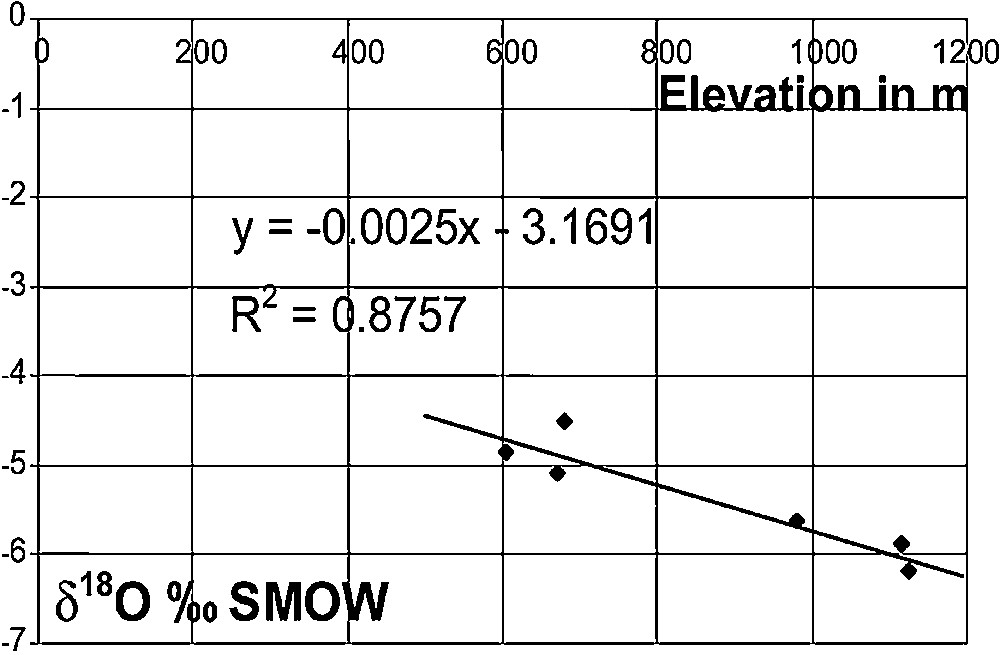
Relationship between the mean annual rain 18O content and the elevation of rain gauge stations in the Saida region.
Fig. 3. Relation entre la teneur en 18O de la pluviosité moyenne annuelle et des stations de récupération de l’eau de pluie, dans la région de Saïda.
During the 1991–1993 period, the mean annual tritium content in rainfall was in the range 8.8–15.1 TU, depending on the stations, with monthly contents between 7.3 and 19.1 TU. The seasonal variation shows the classical spring peak and winter minimum. Spatial variations are complex, different from what was observed for chemical and 18O contents.
4.3.1 Chemical and isotopic characteristics of groundwater and river water
4.3.1.1 Brief description and interpretation of physical and chemical data
Only the two 1992 field surveys are considered here: in May (Table 2) during the end of the recharge period (about 265 mm since September), and in November (Table 3), at the end of the low-flow period (around 111 mm since June). The two other surveys, with less data, strengthened the interpretation. The May study concerns 105 sampling sites for physical and chemical data, major ions and 18O, 2H, 3H contents, and 30 sites for carbonate isotopic species. The November one comprises 110 sites for physical, chemical, major ions and 18O contents.
Mean chemical contents for samples collected during May 1992 (concentration in mg/L)
Tableau 2 Principales concentrations en éléments chimiques dans les échantillons recueillis pendant le mois de mai 1992 (concentration en mg/L)
Boreholes | Springs | Wells | Average | |||||||
Min | Max | Average | Min | Max | Average | Min | Max | Average | ||
T°C | 18.1 | 44.7 | 24.2 | 16.8 | 19.5 | 17.98 | 13.5 | 23.2 | 16.3 | 19.35 |
pH | 6.52 | 8.2 | 7.2 | 6.81 | 7.41 | 7.17 | 6.77 | 8.6 | 7.35 | 7.27 |
C (μS/cm) | 273 | 2820 | 784 | 392 | 629 | 487 | 343 | 4800 | 1098 | 897 |
pCO2 (10–2 atm) | 0.15 | 9.6 | 2.44 | 0.76 | 5.8 | 2.08 | 0.06 | 6.5 | 1.34 | 1.85 |
Ca | 4.0 | 116.2 | 40.7 | 8.0 | 32.1 | 18.6 | 6.0 | 32.1 | 18.6 | 39.7 |
Mg | 12.2 | 138.8 | 65.0 | 49.6 | 80.0 | 61.8 | 17.3 | 637.2 | 94.1 | 78.8 |
Na | 6.0 | 920.0 | 97.8 | 6.0 | 32.0 | 15.6 | 0.9 | 730.0 | 83.0 | 79.4 |
Cl | 35.9 | 862.7 | 146.6 | 23.1 | 123.9 | 49.0 | 29.1 | 1371.0 | 207.0 | 162.9 |
SO4 | 51.8 | 1516.8 | 289.0 | 21.1 | 125.8 | 76.8 | 15.4 | 5041.9 | 392.6 | 311.0 |
HCO3 | 115.9 | 378.2 | 288.5 | 225.7 | 372.1 | 277.6 | 128.1 | 530.1 | 269.6 | 278.2 |
NO3 | 3.1 | 60.1 | 22.9 | 18.6 | 62.0 | 33.5 | 3.7 | 140.7 | 55.8 | 40.3 |
Mean chemical contents for samples collected during November 1992 (concentration in mg/L)
Tableau 3 Principales teneurs en éléments chimiques pour les échantillons recueillis pendant le mois de novembre 1992 (concentration en mg/L)
Boreholes | Springs | Wells | Average | |||||||
Mini | Maxi | Average | Mini | Maxi | Average | Mini | Maxi | Average | ||
T°C | 16 | 44 | 22.9 | 14.3 | 19 | 17.04 | 10.4 | 19.5 | 15.69 | 18.42 |
pH | 6.94 | 7.8 | 7.32 | 6.97 | 7.56 | 7.35 | 6.96 | 8.06 | 7.5 | 7.41 |
C (μS/cm) | 446 | 4500 | 1238 | 328 | 1031 | 721 | 383 | 7680 | 1608 | 1340 |
pCO2 (10−2 atm) | 0.3 | 2.9 | 1.11 | 0.51 | 2.8 | 1.18 | 0.12 | 2.23 | 0.76 | 0.95 |
Ca | 12.0 | 292.6 | 49.5 | 12.0 | 62.1 | 35.7 | 8.0 | 314.6 | 66.7 | 54.1 |
Mg | 42.5 | 111.8 | 69.3 | 32.8 | 77.8 | 62.4 | 20.7 | 736.7 | 92.0 | 79.4 |
Na | 9.2 | 556.6 | 75.0 | 9.2 | 57.5 | 22.5 | 4.6 | 418.6 | 87.6 | 73.6 |
Cl | 32.0 | 852.0 | 157.6 | 32.0 | 134.9 | 47.6 | 28.4 | 1356.1 | 213.0 | 168.6 |
SO4 | 9.6 | 1099.2 | 153.1 | 14.4 | 62.4 | 39.8 | 9.6 | 1953.6 | 184.8 | 152.2 |
HCO3 | 73.2 | 402.6 | 245.2 | 128.1 | 451.4 | 276.9 | 79.3 | 494.1 | 255.6 | 255.0 |
NO3 | 5.6 | 74.4 | 27.9 | 18.6 | 71.3 | 38.4 | 6.2 | 607.6 | 93.6 | 151.9 |
Generally, the dissolved solid concentrations increase from the high-flow to the low-flow season, as commonly observed in karst aquifers [3]. This increase, shown by the EC, is related either to higher contents in sulphate and calcium in some waters, or in chloride, sodium and magnesium in others. Taking into account the high observed concentrations, in both cases these dissolved solids are typical of evaporites leached by groundwater. The gypsum saturation index SIg is always lower than 0, except for groundwater from the Sidi Aissa and Kerkab boreholes, saturated in November. This shows that the proportion of water flowing for a long time in contact with sulphate evaporites in the aquifer system is very low, except locally.
Water temperatures vary in the range of 10.4–44.7 °C, with an average around 23.0 °C in boreholes, 17.5 °C at springs and 16.0 °C in shallow wells. Temperatures lower than or equal to the mean annual air temperature at Saida (15.7 °C) observed in some wells and springs characterise local thermal conditions and possible exchanges with rivers. Boreholes and springs are on the whole marked by the participation of deep, thermal groundwater, which does not occur in wells. This thermal groundwater, discharging in the Hammam Rabbi and Sidi Aissa area, and at Kerkab and Sidi Amar, has a high temperature in the range of 26 to 45 °C.
4.3.1.2 Description and interpretation of carbonate system data
All waters from limestone and dolomite currently have a calcium, and often magnesium carbonate composition, often more complex because of an evaporite contribution, calcium sulphate and/or sodium chloride. Then, the calcium carbonate system must be studied as a privileged informer, considering not only the measured variables (pH, Ca, Mg, HCO3), but also the calculated ones, the CO2 partial pressure, pCO2, and saturation indexes for calcite, SIc, and dolomite, SId [3]. The average pH (7.4), identical for all water types, in the range of 6.52–8.6, is classical for water from carbonate terrains. It explains the relationship between dissolved CO2 at a given pCO2, water and rock, as a function of the contact time and/or the existence of an external atmosphere controlling degassing of dissolved CO2 [3]. pH values higher than 8.0 correspond to degassed water, collected from rivers or widely open wells. The lowest values correspond to high pCO2. Shallow groundwater pH is slightly higher than neighbouring boreholes and springs because of CO2 degassing. Some boreholes have a pH lower than 7.0, corresponding to a pCO2 up to 0.1 atm. Groundwater normally presents pCO2 in the range of 1–3 × 10–2 atm when CO2 is supplied only by the soil [3]. Calculated pCO2 in the Saida area are between 0.06 and 9.6 × 10−2 atm, i.e. from values slightly higher than the atmosphere up to values much higher than normal pCO2 in soils and groundwater. This means that groundwater from the carbonate aquifer is locally highly enriched in CO2. We will show later that the enrichment is due to CO2 of deep origin, related to geothermal groundwater. The comparison of the two 1992 surveys reveals a general CO2 depletion during the low-flow season (Table 4). The recharge obviously contributes to the transport of a CO2 excess in the phreatic zone, identifiable by a pCO2 much higher than 3 × 10−2 atm.
Mean CO2 partial pressure and pCO2 values for samples collected during May and November 1992, in 10−2 atm
Tableau 4 Pression partielle moyenne de CO2 et valeurs de p CO2 pour les échantillons recueillis au cours des mois de mai 1992 et novembre 1992 en 10–2 atm
May | November | |||||||
Boreholes | Springs | Wells | All samples | Boreholes | Springs | Wells | All samples | |
Minimum | 0.3 | 0.76 | 0.06 | 0.06 | 0.3 | 0.42 | 0.12 | 0.12 |
Maximum | 9.6 | 5.8 | 6.5 | 9.6 | 2.9 | 2.8 | 2.23 | 2.9 |
Moyenne | 2.44 | 2.08 | 1.34 | 1.85 | 1.11 | 1.18 | 0.76 | 0.95 |
The total dissolved inorganic carbon (TDIC), total of HCO3– and hydrated CO2, CO32– ions being negligible at pH lower than 8.3, is in the range of 2.16–10.22 mmol/l, about 5.0 on average. Because springs integrate the whole groundwater flow, their TDICs are less dispersed than at wells and boreholes. TDIC from well water, highly dispersed, is lower than in boreholes, probably because of the precipitation of calcium carbonate in some wells, related to CO2 degassing. The highest TDIC values, associated with the highest NO3 concentrations, are due to pollution by waste waters.
Saturation indexes indicate that the groundwater had a contact time with an abundant mineral long enough to dissolve it (SI ≤ 0). Positive saturation indexes (SI > 0) indicate that water underwent important changes, CO2 degassing for carbonate minerals, temperature lowering and/or common ion enrichment for the others. Consequently, saturation indexes provide interesting information about groundwater flow conditions [3].
The calcite saturation index, SIc, indicates either a CO2 degassing when SIc > 0; therefore, a flow in contact with a gaseous phase, or a short contact time of groundwater with carbonate rocks when SIc < 0. When SIc = 0, this means that the contact time was at least a few weeks, and that groundwater has flown in the phreatic zone under confined conditions, not as an open channel flow. All the sampling sites in May show SIc ≤ 0, except the supersaturated water from the SNMC (No. 18) borehole. In November, only five boreholes show SIc < 0, all others have SIc ≥ 0. During high flow, most springs have likewise SIc < 0, whereas at the end of the low-flow, most have SIc > 0. As for other sampling sites, in May the wells have SIc ≤ 0, whereas in November most of them have SIc ≥ 0. This regional trend shows that after recharge, groundwater CO2 degassing occurs everywhere, generating calcite precipitation.
When SId < 0, the dolomite saturation index, SId, indicates that the water may dissolve dolomite. Contrary to SIc, SId > 0 cannot be interpreted as a possibility of precipitating dolomite, but shows that calcite has precipitated, enriching the solution in Mg. In May as well as at the end of the low-flow season, most boreholes are undersaturated or saturated with respect to dolomite (SId ≤ 0), as well as the springs. However, a general trend from undersaturation toward saturation, sometimes supersaturation, indicates that during low-flow periods, CO2 degassing and calcite precipitation may occur inside the aquifer or in the wells. This trend confirms that observed for calcite.
4.3.1.3 Interpretation of data from boreholes by means of factor analysis
Principal component analysis (PCA) was used to show [3] the distribution of thermal waters in the main aquifer. PCA is an efficient tool for summarising a large number of data in order to form groups characterised by comparable variations, driven by factors explaining groupings [3]. It groups the parameters whose variations are interrelated, as a function of factors explaining the observed groupings. The meaning of the factors is then studied to suggest assumptions about the causes for spatial and/or temporal variations of the studied variables. An analysis of the variable space shows the relationships between variables and factors structuring the dataset. The sample space shows the relationships between samples or sampling sites and factors. The factors responsible for the variation of the water chemical content can then be identified and their respective share quantified.
The analysis considered a table of 36 samples from boreholes, with 15 variables, from the May 1992 sampling campaign (Figs. 4 and 5). The main goal was to identify thermal water characteristics of deep origin and their entry into the studied aquifers. The considered variables are the following: temperature T, electrical conductivity C, related to the total dissolved solids; concentrations of dissolved inorganic compounds (Ca, Mg, Na, K, HCO3, SO4, Cl, NO3, SiO2); parameters of the CO2–H2O–carbonate system, pCO2 and saturation indexes SIc, SId and SIg.
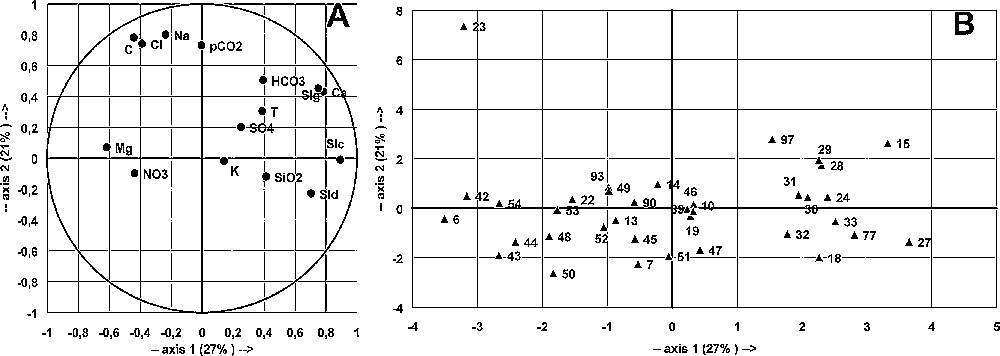
Principal component analysis of borehole samples collected during May 1992. Main plane F1–F2 for variables (A) and samples (B).
Fig. 4. Analyse en composantes principales des échantillons de forages recueillis au cours du mois de mai 1992. Plan principal F1–F2 pour les variables (A) et les échantillons (B).
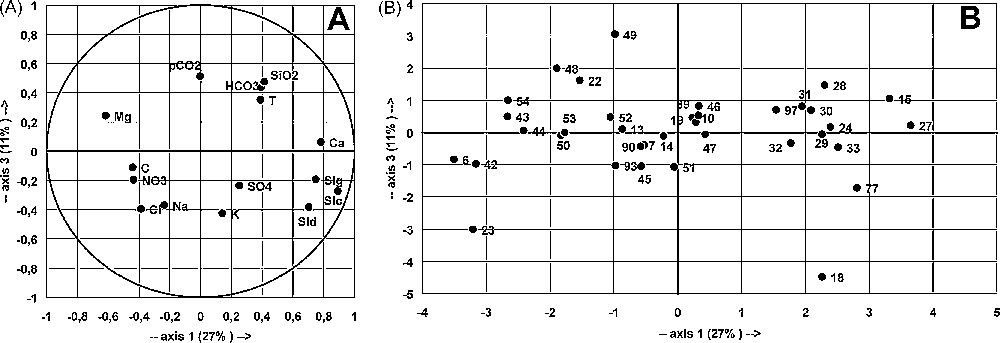
Principal component analysis of borehole samples collected during May 1992. Main plane F1–F3 for variables (A) and samples (B).
Fig. 5. Analyse en composantes principales des échantillons de forages recueillis au cours du mois de mai 1992. Plan principal F1–F3 pour les variables (A) et les échantillons (B).
The data are poorly structured, as shown by the total (60%) variance explained by the three main factors (F1, 27%; F2, 21%; F3, 11%). In the variable space, F1 axis is defined by SIc, SId, SIg and Ca opposed to C, Cl and Mg; F2 axis is defined by pCO2, Na, Cl and C; F3 axis, poorly defined by pCO2, SiO2, HCO3 and T, is opposed to Na, K, Cl and SId (Figs. 4 and 5). The three factors include temperature in almost equal proportions, showing that thermal characteristics are driven by multiple factors, related to different geochemical histories according to the location inside the aquifer. This is a likely consequence of tectonic partitioning of the aquifer, as shown in another thermal karst aquifer in Algeria [13]. Abnormal temperatures may also be due to a high regional geothermal gradient affecting more or less all groundwater flows.
In the sample space, four groups, A, B, C and D were considered. Site 23 (Tircine), a Na- and Cl-rich water, with a high C and pCO2, opposed to all other waters, determines F2 axis (Figs. 4 and 5): Tircine is mostly deep groundwater that has leached evaporites and is enriched in deep CO2. Some other waters, Redjem (15), Sidi Gacem (28), Rebahia F27 (29) and Sidi Mimoun P 47 (97), show similar characteristics, with lower concentrations of Na and Cl. Group A assembles Ca-rich and high SI waters, opposed to group B. Groups C and D defined by F3 are difficult to interpret.
Neither space nor geological organisation can be defined within the groups, despite their strong spatial heterogeneity. The great spatial heterogeneity and the weak structuring of chemical data are the consequences of a split in the carbonate aquifer, caused by the graben and horst structure and/or by the karst drainage organisation. Comparable results were obtained by the other sampling campaigns. Complementary data, such as depth, pumping rate, etc., may help to explain the observed associations.
In the same way, the CO2 distribution is not related to the geological structure. pCO2 is related to factor F2, mainly determined by the Tircine borehole (23), opposed to all other sites. Some boreholes (15, 25, 97) also present high pCO2, more than 10−1 atm; they are situated mainly between Saida and Sidi Mimoun (Fig. 1), where a large number of boreholes are located.
4.3.1.4 Oxygen-18 content in groundwater
Seasonal variations were observed in the 18O contents, with groundwater more depleted in November, at the end of the low-flow season, than in May (Table 5), but within a very narrow range. Groundwater from boreholes is more depleted, on average −6.7 to −7.6‰, respectively in May and November, than that from shallow wells, on average −6.3 to −7.0‰. For most groundwater samples, the variation is lower than 1‰, which can be interpreted as the consequence of a homogenisation within the aquifer. However, a similar range of variation in 18O contents is observed in other karst aquifers [7,9,16,18,20,24].
18O contents in groundwater, in ‰ SMOW
Tableau 5. Teneurs en 18O dans l’eau souterraine en ‰ SMOW
May 1992 | November 1992 | Mean change | |||||
Mini | Maxi | Average | Mini | Maxi | Average | ||
Boreholes | −8.4 | −4.8 | −6.7 | −8.6 | −6.8 | −7.6 | −1.3 |
Springs | −7.1 | −5.7 | −6.5 | −7.9 | −6.3 | −7.3 | −0.9 |
Wells | −7.6 | −2.3 | −6.3 | −7.9 | −6.0 | −7.0 | −0.8 |
Regional average | −8.4 | −2.3 | −6.5 | −8.6 | −6.0 | −7.3 | −1.0 |
The elevation of the spring recharge areas was calculated from the spring elevation and the regional elevation gradient of the 18O content in rainfall, −0.25‰ per 100 m. The calculated elevations are in good agreement with the geological and geographical locations of the springs, except for two of them, Aïn Skhouna and Hammam Rabbi spa. For the first spring, the recharge area elevation is the same as the spring. For the second, it is definitely lower, which means that this groundwater is enriched in 18O compared to the present-day recharge. These two springs, especially Hammam Rabbi, show typical thermal characteristics. Some boreholes (Ouled Djelloul 4, Sidi Aïssa 37, Kerkab 38) and shallow wells (94, 102 and 103) are also enriched in 18O. It has been shown [14] that thermal waters can be enriched in 18O in aquifers, because of water–rock interactions at temperatures higher than 80–100 °C. The isotopic enrichment observed in some groundwater in the Saida area is interpreted as the contribution of thermal groundwater, at temperatures higher than 80 °C, flowing either at great depths, or in an area with a high geothermal gradient.
Consequently, silica and (Na + K) geothermometers were applied to springs and to the main thermal boreholes. The (Na + K + Ca) geothermometer was not used, because the aquifer formation, mainly carbonated, contains Ca in abundance, and deep CO2 plays an important part in its dissolution. Temperatures calculated with the two geothermometers are very close: the averages, between 75 °C and 110 °C, are in good agreement with the 18O enrichment. For a normal geothermal gradient, such temperatures correspond to a flow depth of around 2000–3000 m, which is in good agreement with the known thickness of the sedimentary series and the graben fault throw.
4.3.1.5 Tritium content in groundwater
Only some samples from the October 1991 and May 1992 campaigns were analysed. Their tritium contents, in the range of 0–36 TU, define three groups of groundwater: (i) recent (3H > 14 TU), (ii) old (3H < 4 TU), and (iii) mixed in the range of 4–14 TU. Tritium contents are higher in October than in May.
The observed differences between the end of the recharge period and the end of the low-flow period, between 3 and 8 TU, are related to the variation of the old groundwater component in the samples, depending on the recharge component and its distribution inside the aquifer. The shallow wells show wider variations than the springs and boreholes, because they are directly recharged from recent, shallow formations.
4.3.1.6 Carbon-13 and carbon-14 content in groundwater
Carbon-13 and carbon-14 were measured on precipitated TDIC, in order to determine the sources of inorganic carbon involved in the dissolution process. Groundwater 13C contents vary within a wide range from −12.2 to −3.0‰ PDB. Four samples with contents higher than –5.3‰ are from the Ain Skhouna and Hammam Rabbi thermal springs and the Kerkab and Sidi Amar artesian boreholes. The 13C content originates from two poles, the dissolution of marine limestone (around 0‰) by the soil CO2 (−25‰), giving TDIC 13C around −12‰ PDB, and or by CO2 of geological origin, produced either by water–rock interactions or by decarbonatation (0‰) from mantle CO2 (around −3‰).
Groundwater 14C activities are all lower than 92% pmc, most of them lower than 70% pmc, half of them being lower than 50% pmc. 13C and 14C contents are not correlated to TDIC (Fig. 6; r = 0.14, n = 30), but are highly correlated with one another (r = 0.76). This correlation is the evidence of a groundwater enrichment in ‘dead carbon’ from exchanges between TDIC and carbonate rock [15]. However, the available data cannot be used to calculate the groundwater age, nor the ratio between the open void area exposed to the water–rock interaction and the groundwater volume [5], which could have yielded information about the extent of karstic features at depth in the aquifer.
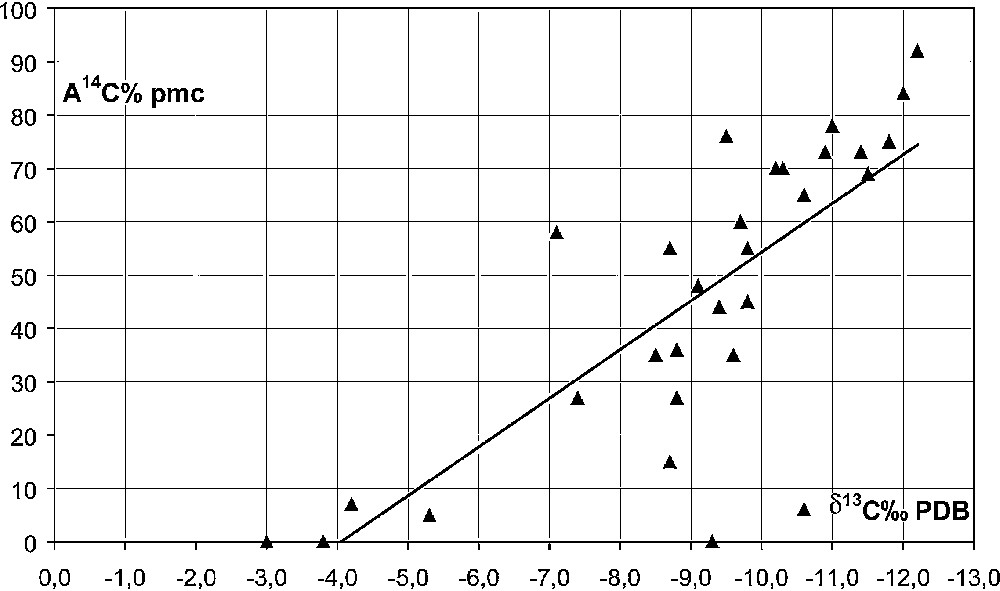
Relationship between 13C content and 14C activities in groundwater.
Fig. 6. Relations entre les teneurs en 13C et les activités 14C dans l’eau souterraine.
5 Discussion and conclusion
The Saida regional aquifer includes a hydrothermal component characterised by relatively high temperatures at depth (80–100 °C) and a deep CO2 flux, not necessarily linked, probably because the deep CO2 rising is local. These thermal waters indicate the presence of a palaeorecharge, which cannot be dated nor climatically defined, because of water–rock interactions at high temperatures and CO2 content at depth. This CO2 thermal component is necessarily at the origin of the karstic conduits in the phreatic zone, which were formed by an ascending water–O2 flux.
Cave systems of hydrothermal origin have been described and studied in several parts of the world [4,8,17]. The conduits crossed at depth in the Saida area in some boreholes, particularly in the northeastern part, where deep CO2 is abundant, show the importance of deep CO2 in the development of hydrothermal karsts. Moreover, the seasonal variability of chemical and isotopic contents in the groundwater also shows the existence of some functioning karst in the phreatic zone. Unlike classic karsts developing from the surface, hydrothermal karsts are located in zones favourable to deep CO2 rising, related to open fractures created by the distension due to the regional geologic structure in horsts and grabens.
At the surface, the concentrated recharge at the contact between impermeable and carbonate rocks shows that a karstic functioning occurs in the recharge zones, contrary to the discharge zones, where springs are numerous and scattered in the graben, the four main springs discharging about 30% of the total outflow. In a well-developed classic karstic system, groundwater flow is organised with a conduit pattern from the recharge area towards a single outflow, which is not observed in the Saida karstic aquifer. Here classical karstic processes have not been able to fully develop a conduit system in the phreatic zone converging to a single discharge point, because the groundwater flow under these semi-arid conditions is very low, requiring a much longer time span for karstic development than under humid conditions [2]. The development of the karst has certainly been modified by hydrothermal phenomena. The large number of springs is most likely the consequence of hydrothermal developments in the phreatic zone by the deep CO2 flux.
Besides the palaeorecharge present in the deep thermal system, the aquifer is presently recharged by direct infiltration on carbonate outcrops concentrated into swallow holes. This present-day recharge is responsible for the variability of spring flow rates, water levels in wells and dissolved chemical contents. As shown by the seasonal sampling, it periodically changes the water head and consequently the proportion of thermal water and deep CO2. These two components, present recharge and thermal groundwater, in proportions variable with time and space, depend on the local interconnection between hydrothermal and classic karstic conduits close to the surface, as well as on climatic conditions. This aquifer structure and functioning should be compared with the one studied in eastern Algeria, in the Constantine area [13], both with active tectonics and deep CO2 fluxes, as also occurs in southern France [1,22].
The complex organisation of flow paths inside the karstic Saida aquifer has important practical consequences. When interconnected conduits are crossed in a borehole, the pumping rate may be high, resulting in an overexploitation, worsened by an increase in the part of thermal waters that may have geochemical characteristics unfit for human consumption or for irrigation, as observed in Spain [11]. These complex drainage systems mix deep, old, unpolluted groundwater with potentially polluted, shallow, recent water. The pollutions in the Saida area are the consequences of the vulnerability of carbonate rock outcrops and of the lack of protective measures. Generally poorly known, this type of complex aquifers should be thoroughly investigated in order to determine the conditions of the development of the karstic drainage system and to suggest appropriate management plans for their sustainable exploitation and protection.