1 Introduction
Starting with [16] and followed by [33] and [93,94], the eastern Variscan belt is interpreted as the result of Devonian to Carboniferous continent–continent collision, resembling the (sub-)recent Himalayan-Tibetan type collisional system. However, there is a wealth of data (see for example [93,94]) suggesting that this orogenic belt could have resulted from an Andean type convergence, i.e. as a typical upper plate orogen located above a long-lasting Devonian–Carboniferous subduction system.
The aim of this article is to show that all the current criteria defining an Andean type of convergent margin are present and surprisingly well preserved in the Variscan Bohemian Massif. These criteria are in particular [66,71,122,157]:
- • the development of Franciscan type blueschist-facies metamorphism in the lower plate;
- • arc type magmatism marked by calc-alkaline to potassium-rich (shoshonitic) series in the distance of 150–200 km from the trench;
- • back-arc basin developed on continental upper plate crust and replaced by thick continental root;
- • deep granulite-facies metamorphism associated with supposed underplating of the crust by mafic magmas at the bottom of the root;
- • continental lithosphere thrust underneath the thickened root system.
Based on these criteria, the architecture of the eastern Variscan belt is interpreted as the result of a large-scale and long-lasting subduction process associated with crustal tectonics, metamorphism, magmatic and sedimentary additions that developed over the width of at least 500 km, in present-day coordinates, and time scale of ∼80 Ma.
2 Present-day architecture of the Bohemian Massif and location of Palaeozoic sutures
The Bohemian Massif occurs at the eastern extremity of the European Variscan belt, representing one of its largest exposures (Fig. 1). From west to east, the Eastern Variscan belt forming the Bohemian Massif can be subdivided into four major units:
- • the Saxothuringian Neoproterozoic basement with its Palaeozoic cover corresponding to the continental crust of the Armorican plate [87,105,147];
- • the Teplá-Barrandian Unit consisting of Neoproterozoic basement and its Early Palaeozoic cover interpreted as an independent crustal block (the Bohemia Terrane of South Armorica sensu [34]);
- • the Moldanubian high- to medium-grade metamorphic domain intruded by numerous Carboniferous granitic plutons, altogether forming the high-grade core of the orogeny;
- • the eastern Brunia Neo-Proterozoic basement with Early to Late Palaeozoic cover [35,140].
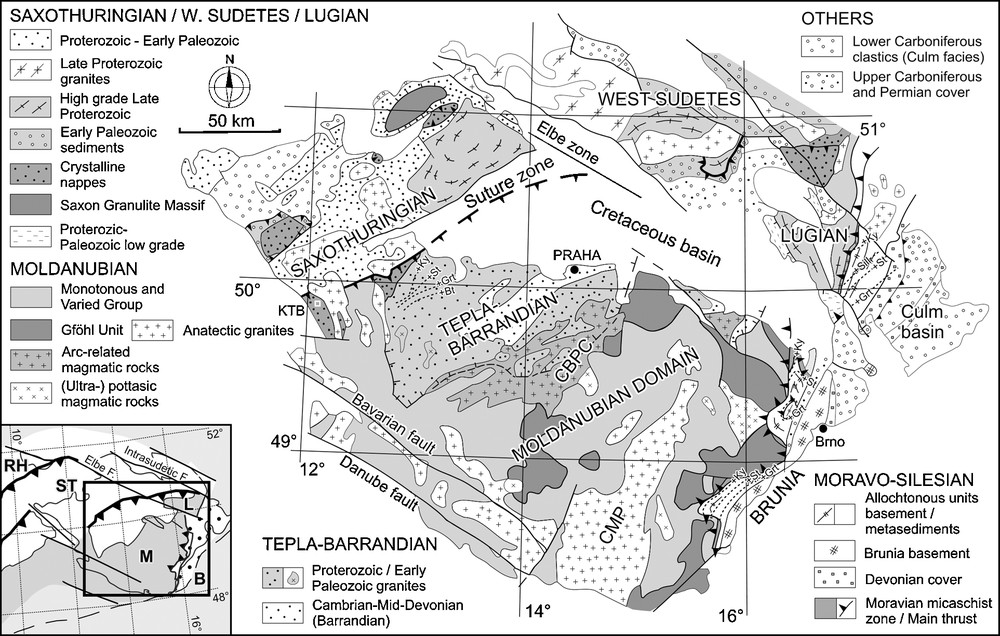
Simplified geological map of the Bohemian Massif (modified after [34]). CBPC: Central Bohemian Plutonic Complex; CMP: Central Moldanubian Pluton. The lower left insert shows position of the Bohemian Massif in the frame of the European Variscides (modified [22]). RH: Rhenohercynian zone; ST: Saxothuringian Zone; M: Moldanubian Zone; B: Brunia Continent; L: Lugian domain.
Fig. 1. Carte géologique simplifiée du Massif de Bohême (modifiée d’après [34]). CBPC : Complexe plutonique de Bohême centrale ; CMP : Pluton central moldanubien. L’encart du bas à gauche montre la position du Massif de Bohême dans le cadre des Variscides européennes (modifié d’après [22]). RH : Zone rhénohercynienne ; ST : Zone saxothuringienne ; M : Zone moldanubienne ; B : Brunia ; L : Zone lugienne.
The palaeontological record of Lower Palaeozoic (Cambrian and Ordovician) sediments of the Saxothuringian and Teplá-Barrandian domains shows affinities to the Gondwana faunas implying that these blocks were derived from the northern Gondwana margin [20,27,28,68]. In addition, there is a range of isotopic and U–Pb zircon data suggesting a Gondwanan provenance of all units composing the Bohemian Massif [96,106].
2.1 Saxothuringian domain
This domain is represented by Neoproterozoic par-autochthonous rocks (580–550 Ma) formed by migmatites and paragneisses intruded by Cambro-Ordovician calc-alkaline porphyritic granitoids converted to augen orthogneiss during the Variscan orogeny [38,39,76,89]. These rocks are unconformably covered by Cambrian and Ordovician rift sequences (Fig. 1) overlain by Late Ordovician to Famennian pelagic sediments and Famennian to Visean flysh of the Thuringian facies [85,125,144]. The par-autochthon is overthrust by allochthonous units containing deep-water equivalents of the Ordovician to Devonian rocks of the para-autochthon, and by proximal flysh sediments (the Bavarian facies).
The allochthonous units occur in Münchberg, Wildenfels and Frankenberg klippens and exhibit pile of thrust sheets marked by decreasing pressure and metamorphic age from the top to the bottom [34]. In the hangingwall occur thrust sheets with Mid-Ocean Ridge Basalt (MORB)-type metabasites of Ordovician protolith age eclogitized (Fig. 2; P = ∼ 25 kbar, T = 650 °C) [34] during the Devonian ∼395 Ma [102]. Structurally deeper occur sheets marked by medium pressure (MP) assemblages and Late Devonian (∼365 Ma) zircon and hornblende cooling ages. This rock pile represents both distal and proximal Late Ordovician to Devonian passive margin rocks tectonically inverted during the Devonian convergence [44,134]. In the Sudetic part (Figs. 1 and 2) of the Bohemian Massif, the Ordovician rift sequences are well developed and marked by the presence of deep marine sediments and MORB-type volcanics followed by Silurian and Devonian sedimentary sequences [95]. The Ordovician oceanic rocks are enhanced by a blueschist-facies metamorphic overprint of Late Devonian age [33,34].
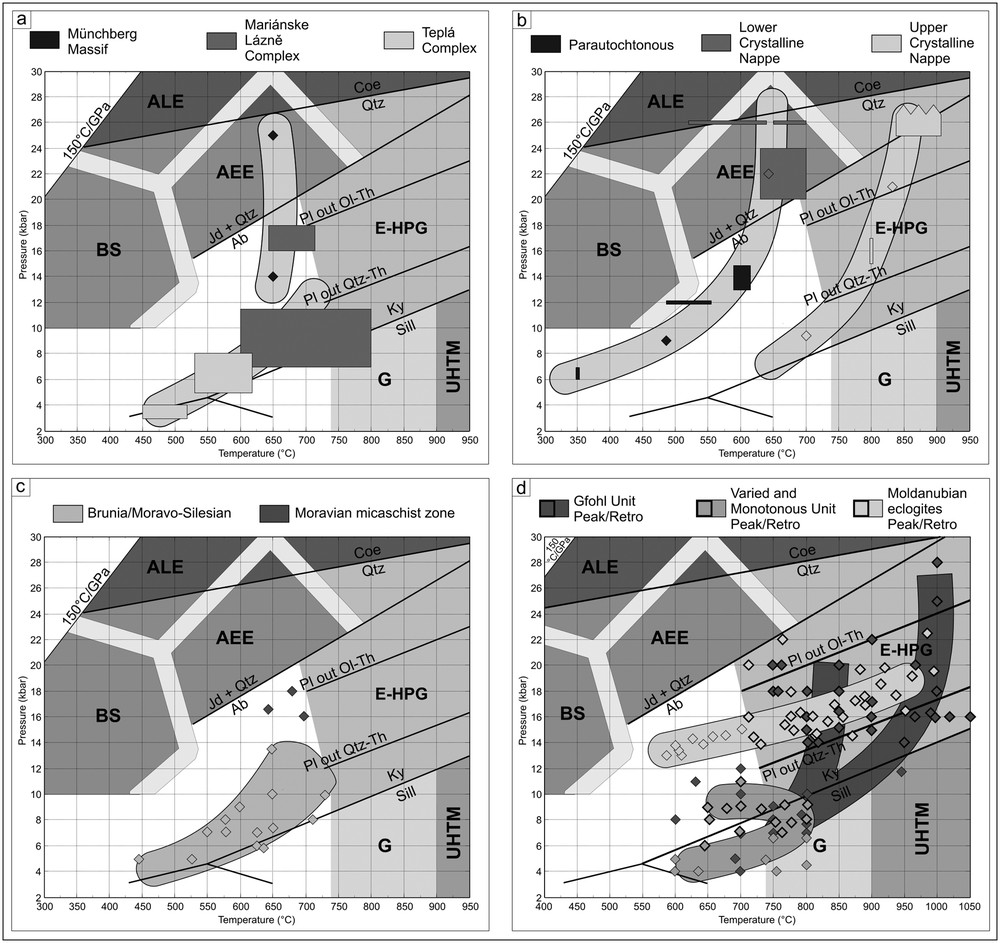
Pressure–temperature (P–T) diagram showing the location of the principal metamorphic facies in the P–T space (after Brown, [6]). BS: blueschist facies; AEE: amphibole-epidote eclogite facies; ALE: amphibole-lawsonite eclogite facies; LE: lawsonite eclogite facies; AE: amphibole eclogite facies; GS: greenschist facies; A: amphibolite facies; E-HPG: medium-temperature eclogite facies – high-pressure granulite metamorphism; G: granulite facies; UHTM: the ultra-high-temperature metamorphic part of the granulite facies. a: Devonian HP–LT metamorphism (390–380 Ma) from the Münchberg and Mariánské Láznĕ units contrasted to Devonian (380 Ma) MP metamorphism of the western part of the Teplá-Barrandian; b: Carboniferous (340 Ma) HP–LT metamorphism from the Saxothuringian par-autochthon and lower nappe contrasted to the HP–HT metamorphism of the granulite bearing upper nappe (modified after [71]); c: Carboniferous (340 Ma) HP–HT metamorphism of the orogenic lower crustal belt from the Moldanubian domain, HT–MP and LP metamorphism related to the exhumation of the orogenic lower crust contrasted to the MP–MT peak metamorphism of the orogenic middle crust followed by heating during exhumation (modified after [123]; d: Carboniferous HP–MT metamorphism of the Moravian eclogites contrasted to the MP–MT Barrovian metamorphism of the Moravian Zone (modified after [77]).
Fig. 2. Diagramme pression–temperature (P–T) montrant la localisation des principaux faciès métamorphiques dans l’espace P–T (d’après [6]). BS : faciès schiste bleu ; AEE : faciès éclogitique à amphibole-épitote ; ALE : faciès éclogitique à amphibole-lawsonite ; LE : faciès éclogitique à lawsonite ; E-HPG : métamorphisme à éclogite de moyenne température et à granulite de haute pression ; G : faciès granulite ; UHTM : partie métamorphique d’ultrahaute température du faciès granulite. a : métamorphisme dévonien HP–LT (390–380 Ma) des unités de Münchberg et Mariánské Láznĕ contrastant avec le métamorphisme MP (380 Ma) de la partie occidentale de l’unité de Teplá-Barrandien ; b : métamorphisme carbonifère HP–LT (340 Ma) du parautochtone Saxothuringien et de la nappe inférieure, contrastant avec le métamorphisme HP–HT de la granulite incluant la nappe supérieure (modifié d’après [71]) ; c : métamorphisme carbonifère HP–HT (340 Ma) de la ceinture orogénique crustale inférieure du domaine moldanubien, le métamorphisme HT–MP et LP, en liaison avec l’exhumation de la croûte orogénique inférieure contrastant avec le pic MP–MT du métamorphisme de la croûte orogénique moyenne, suivi d’une élévation de température pendant l’exhumation (modifié d’après [123]) ; d : métamorphisme carbonifère HP–MT des éclogites moraviennes, contrastant avec le métamorphisme MT barrovien de la zone moravienne (modifié d’après [77]).
However, a later Carboniferous underthrusting of the Saxothuringian/Armorican continental rocks underneath the easterly Teplá-Barrandian block was identified suggesting a continuous convergence in this area, which was responsible for eclogitization of both the oceanic and continental crust resulting from continental underthrusting [90] at ∼340 Ma [71]. The continental underthrusting reached even the pressure conditions of the diamond stability [150]. This event is responsible for the global reworking of the Saxothuringian terrane at HP conditions, imbrication of subducted continental crust and exhumation of deep rocks in form of crustal scale nappes [92]. Thus, the structure of the Saxothurinigian domain is defined by par-autochthonous domain (Fig. 2; P = 13–15 kbar, T = 580–630 °C) [69,70,72] and eclogite-bearing “lower” crustal nappe (P = 20–26 kbar, T = 630–700 °C) [70]. The highest tectonic unit, “the upper nappe” reached the high pressure (HP) granulite facies peak conditions (P = 15–20 kbar, T = 800 °C [69,116]) at 340 Ma [79,154]. The exhumation of granulite facies unit occurred at 340 Ma as shown by 40Ar/39Ar cooling ages [80,83].
2.2 The Saxothuringian–Teplá-Barrandian boundary
The boundary between the two crustal domains is characterized by the presence of units with high proportion of ultramafic and mafic rocks (Fig. 1; Mariánské Lázně Complex and Erbendorf–Vohenstrauss Zone). The former complex is marked by a presence of serpentinites at the bottom overlain by a thick sequence of amphibolites, eclogites and metagabbros. The U–Pb zircon protolith ages discriminate the mafic rocks into two main groups with Cambrian (∼540 Ma [145]) and Ordovician (∼496 Ma [5]) ages. On the other hand, the metamorphic and cooling ages (Sm–Nd garnet–pyroxene and Ar–Ar hornblende) are Devonian, ranging between 410 and 370 Ma [4,15]). Similarly, the newly determined U–Pb ages for metamorphic zircon in mafic rocks, as well as monazite from orthogneiss, and titanite in leucosome all cluster around 380 Ma and possibly date the exhumation of the whole Mariánské Lázně Complex [163]. The Devonian metamorphic evolution started with eclogite-facies metamorphism (Fig. 2; P = 16–18 kbar, T = 640–715 °C) [145] and continued at c. 380 Ma by granulite-facies re-equilibration [99]. The Erbendorf–Vohenstrauss Zone further west shows similar lithological and petrological zonation as the Mariánské Lázně Complex and it is thus commonly interpreted as a part of the same tectonic unit [101,145].
2.3 Teplá-Barrandian domain
Stratigraphically, the Teplá-Barrandian Unit (Fig. 1) consists of Neoproterozoic basement with the lower arc-related volcano-sedimentary sequence (the Kralupy–Zbraslav Group), followed by siliceous black shales and a flyshoid sequence (shales, greywackes and conglomerates, [35]). The Neoproterozoic basement is unconformably overlain by a thick sequence (1500–2000 m) of Lower Cambrian conglomerates, greywackes, and sandstones [19,82] followed by shales and a rift-related volcanic sequence in the Upper Cambrian. The development of the Lower Palaeozoic Prague Basin [17] is marked by Early Ordovician (Tremadocian) transgression followed by mid-Ordovician rifting associated with volcanic activity, and with sedimentation of Silurian graptolite shales. The sedimentation continued mainly with carbonates, namely the Upper Silurian to Devonian calcareous flyshoid sequence. The Early Silurian was associated with important volcanic activity, accompanied by basaltic and ultramafic intrusions [51]. The sedimentation terminated in mid-Devonian with distal turbidites of the Srbsko Formation (Givetian) containing, among others, detrital zircons of Devonian (∼390 Ma) age [108].
The whole sequence is folded by steep folds presumably of Late Devonian age as shown by Culm facies sediments unconformably deposited on folded Ordovician strata [135]. The deformation affected also the underlying Neoproterozoic basement, having intensity and age increasing progressively to the west. In the same direction rises also the metamorphic grade reaching amphibolite-facies conditions close to the Teplá-Barrandian/Saxothuringian boundary [10]. In this area is developed a typical Barrovian metamorphic zonation ranging from biotite–garnet zone (Fig. 2a; P = 3–4 kbar, T = 450–520 °C) [146,156,160,161] in the east up to kyanite zone (Figs. 1 and 2a; P = 5–8 kbar, T = 530–620 °C) [156] in the west marked by a “normal” gradient (increase of pressure and temperature to the structural footwall). The 40Ar/39Ar muscovite and hornblende dating yielded exclusively Middle Devonian cooling ages [155].
2.4 The Teplá-Barrandian–Moldanubian domains boundary – the Central Bohemian Plutonic Complex
The igneous activity in the area of the Central Bohemian Plutonic Complex (Fig. 1) started with intrusions of calc-alkaline Devonian (protolith ∼370 Ma) tonalites to granodiorites, latter transformed into highly sheared orthogneisses [74]. The first unmetamorphosed plutonic rocks were Late Devonian (∼354 Ma) calc-alkaline tonalites, granodiorites, trondhjemites, quartz diorites and gabbros of the Sázava suite [62,64,158]. Their Sr–Nd isotopic ratios and trace-element signature indicate that the source of the basic magmas was a slightly depleted mantle above a subduction zone. In addition, a significant role for mixing with acidic magmas is to be assumed [60,64]. Further south/southeast occur voluminous Early Carboniferous (∼349–346 Ma [18,55,56]) high-K calc-alkaline plutonic bodies of the Blatná suite (mainly granodiorites with minor quartz monzonite and monzogabbro bodies). The intermediate rock types resulted from mixing of slightly enriched mantle-derived and crustal magmas [61]. Finally, further east occur syn-deformational bodies or post-tectonic elliptical intrusions of (ultra-)potassic rocks of mid-Carboniferous (∼343–337 Ma) ages [53,55,63,157]. Both the Sázava and Blatná suites contain numerous xenoliths, screens and roof pendants of the Barrandian-like Palaeozoic and Neo-Proterozoic sequences, indicating a major role for stopping as an emplacement mechanism [159].
All these features indicate that the Central Bohemian Plutonic Complex corresponds to a relatively shallow section (<10 km) through the Devonian–Carboniferous magmatic arc, which widened and expanded to the east with time. There is also a remarkable temporal trend of increase in the potassic character of magmas, presumably as the basic magmas tapped increasingly more enriched mantle sources [56,60,62].
2.5 The Moldanubian domain
According to Fuchs [41,42], three major units define this domain (Fig. 1):
- • the “Monotonous Group” of Proterozoic metasediments, with numerous Late Proterozoic to Early Palaeozoic orthogneisses [25,39,153], quartzites and amphibolites. The Monotonous group is traditionally considered to represent the structurally deepest tectonic unit;
- • structurally above the “Varied Group” composed of plagioclase-bearing paragneisses, quartzites and marbles intercalated with amphibolites and leptynites. The protoliths of metasediments are supposed to be at least partly Early Palaeozoic in age, based on the depleted-mantle Nd model ages of 430–500 Ma for the amphibolite layers [67] or on the Devonian age of the intimately associated felsic metavolcanics [37];
- • structurally highest the “Gföhl Unit” (Fig. 1) composed of orthogneiss with Ordovician protolith ages [39], amphibolitized eclogites, granulites, garnet- and spinel-bearing peridotites surrounded by felsic migmatites.
The upper amphibolite-facies metamorphism developed on the regional scale in the Monotonous and Varied groups reflecting maximal pressures of 10 kbar at temperatures of 650–700 °C (Fig. 2) [109,112]. However, higher grade (eclogitic) boudins have been identified, generally at the boundary between both groups (Fig. 2) [23,97].
Metamorphism of the Gföhl Unit is characterized by early eclogite facies (Fig. 2; ∼20 kbar, 650 °C) [97,129] followed by granulite-facies re-equilibration [103,129] and retrogression under amphibolite-facies conditions [131,142]. The granulites of the Gföhl Unit are considered as a product of prograde metamorphism with peak metamorphic assemblage of garnet-kyanite-mesoperthite-quartz in felsic protoliths and clinopyroxene-garnet-mesoperthite in the intermediate varieties indicating P–T conditions of c. 1000–1050 °C and 16–18 kbar (Fig. 2c) [8]. Associated Gföhl orthogneiss shows peak metamorphic conditions similar to granulites between 14–16 kbar above 950 °C (Fig. 2) [12]. However, Štípská and Powell [128] attributed the ultra-high temperature conditions to the magmatic emplacement of granulite protoliths at moderate depth of c. 7–13 kbar, followed by cooling and prograde metamorphic overprint and decompression occuring at temperatures lower than 850 °C [128,129]. The granulite-facies overprint is probably Visean in age as shown by a number of zircon ages [1,84,122,131,153] but recent studies indicate possible Devonian age of ∼370 Ma [2].
Based on existing pressure-temperature (P–T) estimates two NW–SE trending belts of HP rocks (granulites, eclogites and peridotites) are distinguished, one located close to the Barrandian–Moldanubian boundary (the western belt of Finger et al. [29]) and the other rimming the eastern margin of the Bohemian Massif. These belts alternate with MP units, represented by the Varied and Monotonous groups, which also form NW–SE trending wide belts.
The deformation history in the Moldanubian Zone reveals early vertical NNE–SSW trending fabrics, associated with crystallization of HP mineral assemblages [31,113,131,142,151]. These are reworked by flat deformation fabrics that are associated with MP to low-pressure (LP) and high-temperature (HT) mineral assemblages [50,133,142,143,151]. The flat fabrics show intense NE–SW trending mineral lineation that is commonly associated with generalized ductile flow towards northeast and this kind of deformation is typical of the whole eastern margin of the Bohemian Massif. The early steep fabrics are dated at 350 to 340 Ma [122], while the ages of the flat ones cluster generally around 335 Ma [123]. In the southwestern part of the Moldanubian domain, younger set of steep NW–SE metamorphic fabrics reworks the flat foliation, having been associated with LP metamorphic conditions at around 325–315 Ma [29,138].
The HP granulites are spatially, structurally and temporally associated with (ultra-)potassic melasyenites to melagranites, which can be divided into two groups differing in modal mineralogy and textures:
- • coarsely porphyritic K-feldspar melasyenites to melagranites of the so-called durbachite group/series with a “wet” mineral assemblage Mg-biotite plus actinolitic hornblende (e.g., Čertovo břemeno and Třebíč intrusions);
- • even-grained melasyenites–melagranites (Tábor and Jihlava intrusions), containing a variously retrogressed, originally almost “dry”, assemblage of two pyroxenes plus Mg-biotite [29,53,59,149].
For the most basic ultra-potassic rocks, the high contents of Cr and Ni with high melting point to derivation from an olivine-rich source (i.e., Earth's mantle). On the other hand, elevated concentrations of U, Th, LREE and LILE, pronounced depletion in HFSE as well as high K/Na and Rb/Sr ratios seem to contradict the mantle origin. This dual geochemical character and crustal-like Sr–Nd isotopic compositions require melting of anomalous lithospheric mantle sources, metasomatised and contaminated by mature crustal material ([59] and references therein), and interaction of these mafic melts with crustally-derived leucogranitic magmas.
The Moldanubian metamorphic units were penetrated by numerous and voluminous anatectic plutons loosely grouped into the Moldanubian (or South Bohemian) Plutonic Complex [26,45,46,54]. These are mostly felsic–intermediate, two-mica granitic to granodioritic intrusions with either S- or transitional I/S type character [26,88,148]. High-resolution conventional U–Pb zircon and monazite dating showed that the bulk of the Moldanubian Plutonic Complex (c. 80%) was emplaced at 331–323 Ma. The fine-grained granodiorites associated with minor diorites followed in a second, less important event at 319–315 Ma [47]. The post-tectonic intrusions of the Moldanubian Plutonic Complex postdated shortly the thermal peak of the regional metamorphism, but were significantly younger than the emplacement of both the Central Bohemian Plutonic Complex and (ultra-)potassic plutonic rocks scattered throughout the Moldanubian domain.
Granitic rocks in the southwestern sector of the Bohemian Massif (Bavarian Forest) have largely independent position. Here, large-scale crustal anatexis was connected with a significant reheating (LP–HT regional metamorphism) and a tectonic remobilisation of the crust (Bavarian Phase sensu [29]). The intrusions northeast of the Bavarian Pfahl Zone are dated between 328 and 321 Ma, whereas ages between 324 and 321 Ma are obtained southwest of this zone. It apparently represents an important terrane boundary and the granitic intrusions sampled distinct basement units [124].
2.6 The Moldanubian–Brunia continental transition zone
This boundary was defined by Suess [136,137] as a zone of severe deformation and metamorphism of continental rocks (the so-called Moravo-Silesian Zone, Fig. 1). In his seminal work, Suess [136] defined the deep thrusting of the Moldanubian Zone over more external and shallower Moravo-Silesian Zone, the latter emerging through Moldanubian nappe in a form of several tectonic windows. The contact between these units is marked by a particular unit, the Moravian “micaschist zone”, which is composed of kyanite-bearing micaschists that were interpreted by Suess [136] as a result of deep crustal retrogression (“diaftorism”) of the Moldanubian gneisses. Modern studies by Konopásek et al. [73] and Štípská et al. [132] have shown that this zone contains boudins of eclogites (Fig. 2; P = ∼ 16 kbar, T = ∼ 650 °C), HP granulites and peridotites embedded in the metapelites. This zone, which contains elements of both Moravian and Moldanubian parentage, is thus regarded as a first order tectonic boundary.
The underlying Moravo-Silesian Zone (Fig. 1) is characterized by two nappes composed of orthogneisses at the bottom and a metapelite sequence at the top. These nappes are thrust over the Neoproterozoic basement which is often imbricated with its Pragian to Famennian cover [9,43]. A number of isotopic studies (e.g. [28]) show that the orthogneisses of the Moravian Zone are derived from the underlying Brunia continent [20]. This 50 km wide and 300 km long zone of intense deformation is marked by an inverted metamorphic sequence ranging from chlorite to kyanite-sillimanite zones and P–T conditions ranging from 5–10 kbar and 550–650 °C (Fig. 2) [77,127]. The prograde metamorphism is interpreted as a result of continental underthrusting associated with intense top-to-the-NNE oriented shearing, development of sheath folds and gneissification of Brunia-derived granite protoliths [118,121]. The subsequent deformation is connected with recumbent folding and imbrication of Neoproterozoic gneisses with Devonian cover [119,120]. This later phase is interpreted as late imbrications of metamorphosed Brunia crust in a kinematic continuum with early underthrusting event. The cooling after the Barrovian metamorphism is constrained at 340–325 Ma by hornblende Ar–Ar data [14,40] and monazite inclusions in garnets yielding 340–330 Ma CHIME ages [78].
2.7 The Brunia continent
The Brunia continental foreland (Fig. 1) originally called the Bruno-Vistulicum by Dudek [20] consists of Neoproterozoic granitoids, migmatites and schists. They reveal the existence of a 680-Ma-old crust, intruded by 550-Ma-old granites [28,40]. This basement is unconformably overlain by Cambrian strata [7], Ordovician and shallow marine Lower Devonian quartzites and conglomerates followed by Givetian carbonate platform sedimentation [3,68]. Since the Early Carboniferous (∼350 Ma), foreland sedimentary environment developed being accompanied by extensional faulting indicating the flexural subsidence of the Brunia margin [49,96]. From 345 until 300 Ma, a 7.5 km thick Variscan flysch (Culm facies) was deposited onto the Brunia foreland. This sedimentation was coeval with the onset of massive exhumation in the neighbouring Moldanubian domain and the continuous loading of the Brunia continent [49]. Low-grade source rocks associated with clastic muscovites dated at 345–330 Ma [117] gradually pass to a high-grade metamorphic source material marked by pyrope-rich mineral fraction and granulite pebbles dated at 340–330 Ma [13,49,81,152]. Since 330 Ma, began also lateral shortening of the flysch basin marked by a deformation front progressively migrating from eastward in conjunction with decreasing intensity of deformation [30,57]. Deformation of this flysch terminated at c. 300 Ma as indicated by Ar–Ar cooling ages of the metamorphosed Culm facies in the west [91] and deformation of the Variscan molasse further east [11].
3 Geodynamic evolution of the Bohemian Massif
The spatial and temporal distribution of geological units, magmatic fronts and metamorphic zones can be interpreted in an evolutionary scheme very similar to that currently reported from the Andean type orogeny. The following account provides a succession of tectonic events that can be interpreted in terms of south-eastward (in the present-day coordinates) oceanic subduction underneath an active continental margin, obduction of the Saxothuringian oceanic domain, formation of a fore-arc region, growth of magmatic arc and development of a large-scale back-arc system on the continental lithosphere. The early oceanic subduction event could have been followed by a continental underthrusting of the Armorican plate leading to increased friction between the upper and lower plates, gradual flattening of the subduction zone marked by eastward migration of arc and subsequent crustal thickening. The latter event could have been responsible for the development of a thick continental root due to thickening of the upper plate represented by the Teplá-Barrandian and Moldanubian crustal material. The final evolution is marked by the continental indentation of eastern Brunia continent into a weak orogenic root, exhumation of the Moldanubian orogenic lower crust, collapse of the Teplá-Barrandian lid and Moldanubian thrusting over the Brunia platform.
3.1 Early Devonian oceanic subduction underneath the active continental margin
The contact between the Saxothuringian-Armorican plate and the overriding Teplá-Barrandian continent is marked by relics of Ordovician MORB eclogites and metabasites locally associated with Ordovician sediments metamorphosed under blueschist–eclogite facies conditions indicating a Mid-Devonian oceanic subduction [34] and an existence of Lower Palaeozoic Saxothuringian oceanic domain (Fig. 3a). The exact age and size of the Saxothuringian ocean is not known, because the differences of Ordovician and Lower Devonian faunas between Saxothuringian and Barrandian continental domains are not confirmed, precluding an existence of large oceanic barrier of biogeographical significance [10,52,106]. Therefore, the oceanic domain must have been narrow and potentially short lived in order not to be recorded by palaeobiogeographic faunal evidence. The only existing argument for larger oceanic separation are from palaeomagnetic data of Tait et al., [141] suggesting that the Saxothuringian and Tepla-Barrandian blocks rotated independently during Silurian and Devonian times.
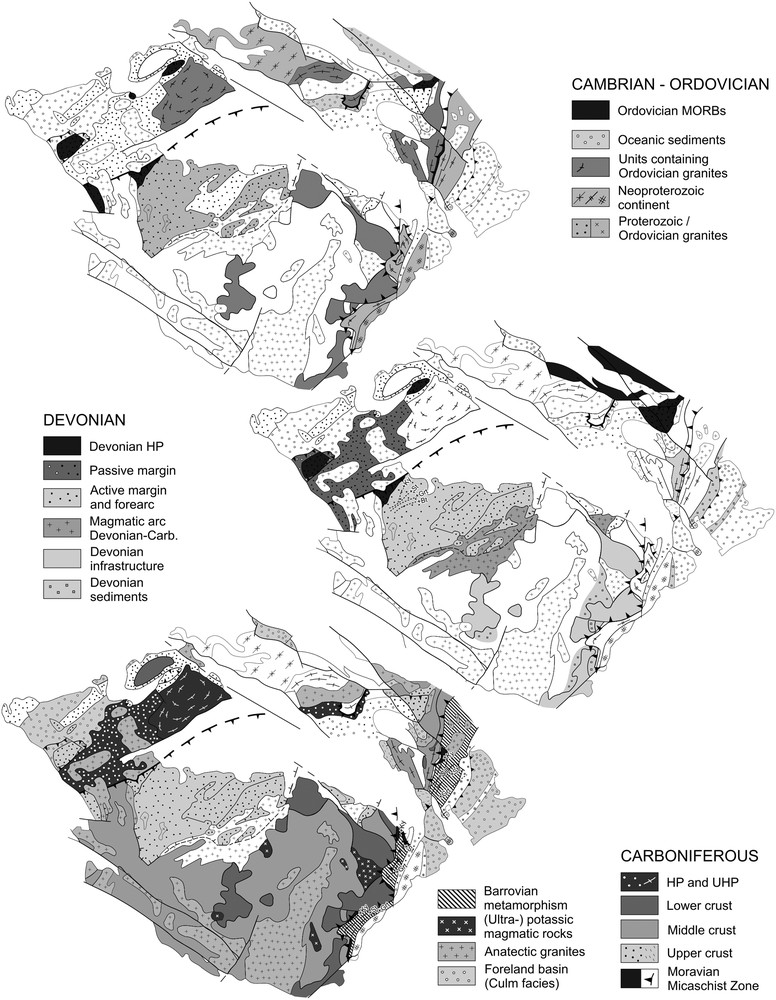
Time slices maps showing the chronological and tectonic significance of the various units of the Bohemian Massif. Top: Ordovician time slice showing the location of Ordovician MORB and deep marine sediments, and that of the Proterozoic units containing the Ordovician magmatism; middle: Devonian time slice showing the location of Devonian high pressure (HP) rocks, the passive margin sequences, the active margin metamorphism, the fore-arc region, the magmatic arc, the location of Devonian infrastructure and sediments; bottom: Carboniferous time slice shows the location of orogenic lower and middle crust belts in the Moldanubian domain, the metamorphism of the Moravian Zone, the Moravian Micaschist Zone, the HP units in Saxothuringian domain and the various types of magmatic rocks.
Fig. 3. Cartes selon des tranches de temps montrant la signification chronologique et tectonique des diverses unités du Massif de Bohême. En haut : tranche de temps ordovicienne montrant la localisation du MORB ordovicien et des sédiments marins profonds et celle des unités protérozoïques incluant le magmatisme ordovicien ; au milieu : tranche de temps dévonienne montrant la localisation des roches de haute pression (HP) dévoniennes, les séquences de marge active, le domaine avant-arc, l’arc magmatique et la localisation de l’infrastructure et des sédiments dévoniens ; en bas : tranche de temps carbonifère montrant la localisation des chaînes orogéniques crustales inférieure et moyenne du Domaine moldanubien, le métamorphisme de la zone moravienne, de la zone des micaschistes moraviens, des unités HP du domaine saxothuriningien et des différents types de roches magmatiques.
The hornblende and mica cooling ages of the Vohenstraus–Erbendorf Zone, Mariánské Lázně complex and the Sovie Gory granulites cluster around 380 Ma, which indicates early collision between the Teplá-Barrandian and Saxothuringian domains (Figs. 3middle and 4B). This is also confirmed by Famennian flysh sediments of the Saxothuringian basin that contain detrital zircons dated at 380 Ma [114] which is a direct evidence of mutual contact between the Teplá-Barrandian and Saxothuringian domains during Famennian but most likely already during mid-Devonian. The Barrovian metamorphic zonation developed at the western flank of the Teplá-Barrandian domain (Figs. 3middle and 4B) is potentially related to deformation of the overriding Teplá-Barrandian continental margin and is consistent with a model of extrusion of lower part of the Teplá-Barrandian crust during Devonian shortening event [160,161]. The folding of the eastern very low grade shales and volcanics of the Neoproterozoic sequences is interpreted as a supracrustal response to the same deformation event affecting high grade rocks in the west [161]. Thus, the exhumation of HP rocks in the Mariánské Lázně Complex and the deformation of the Teplá-Barrandian basement are coupled and related to convergent processes at the Saxothuringian plate boundary (Figs. 3middle and 4B).
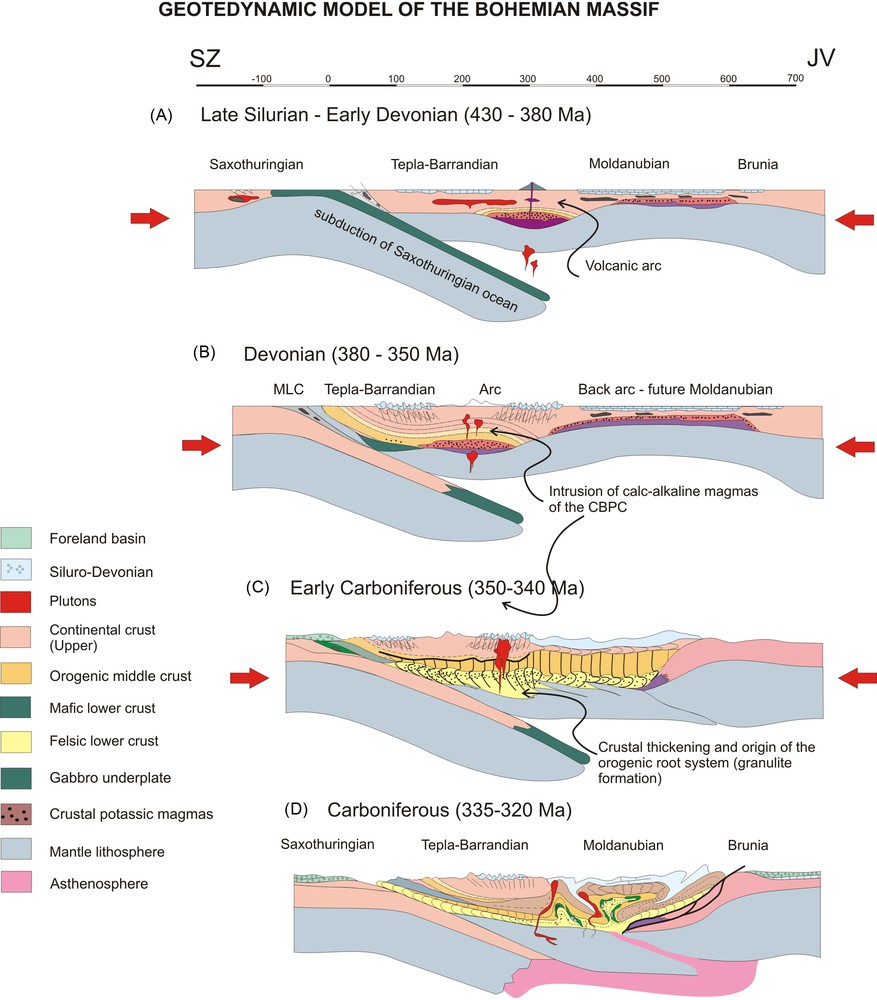
Conceptual model of the geodynamic evolution of the Bohemian Massif shown as schematic cross sections through the orogen. A. Silurian–Early Devonian subduction setting with the beginning of arc and back-arc formation on upper plate. B. Onset of the Mid-Devonian continental underthrusting of Saxothuringian lithosphere, deformation of active margin, formation of magmatic arc and persistence of back-arc region on the upper plate. C. Crustal thickening processes marked by the influx of Saxothuringian crust and progressive individualization of the Brunia active margin. D. Brunia continent indentation associated with the channel flow process, imbrications of underthrust Brunia and the formation of the Moravian Zone.
Fig. 4. Modèle conceptuel de l’évolution géodynamique du Massif de Bohême représenté par des coupes schématiques au travers de l’orogène. A. Subduction Silurien–Dévonien inférieur se mettant en place avec le début de la formation de l’arc et de l’arrière-arc sur la plaque supérieure. B. Au Dévonien moyen, mise en place du sous-charriage continental et de la lithosphère saxothuringienne, déformation de la marge active, formation de l’arc magmatique et persistance de la zone d’arrière-arc sur la plaque supérieure. C. Processus d’épaississement crustal marqué par la venue de croûte saxothuringienne et individualisation progressive de la marge active de Brunia. D. Indentation de Brunia, associée à un processus de flux de chenal, imbrications du sous-charriage de Brunia et formation de la zone moravienne.
The strong argument for eastward subduction of the Saxothuringian ocean underneath the eastern Teplá-Barrandian continent is a Devonian–Lower Carboniferous magmatic arc that is firmly founded on the continental crust. This arc, the Central Bohemian Plutonic Complex, separated the Teplá-Barrandian domain from the future Moldanubian domain and in this model the former unit represented a fore-arc region during Devonian (Figs. 3middle and 4B). Thus, the position of Devonian HP rocks thrust over the Saxothuringian basement, existence of the Mariánské Lázně Complex – the oceanic fragment at suture position, and location of calc-alkaline magmatic rocks further east confirm a polarity of the oceanic subduction underneath the eastern fore-arc and magmatic arc system during Late Devonian (Figs. 3middle and 4B). The distance between the arc and the trench area represented by the suture indicates that the dip of subduction zone was probably moderate (30–40°, Fig. 4B). The temporal evolution of magma geochemistry from calc-alkaline to more potassic/shoshonitic affinities (from 370 to 336 Ma) is compatible with flattening of the subduction zone and increased melting of continental material during Early Carboniferous. The Barrandian (Prague) Basin is interpreted as a part of the fore-arc basin marked by 380-Ma-old detrital zircons found in Mid-Devonian flyshoid sequences [135] suggesting early erosion of the eastern magmatic arc.
The most problematic question in the Devonian subduction model for the Bohemian massif is the role of the future Moldanubian domain as well as the position of the Brunia continent. The amphibolites derived from Siluro-Devonian tholeiitic basalts associated with carbonates, widespread in Lower Austria and South Bohemia, are interpreted as volcanic products of a relic of large-scale back-arc system [24,67]. In addition, the felsic metavolcanics and amphibolite layers in the Varied Group are regarded as the continuity of back-arc bimodal volcanism till Givetian [37]. However, the supposed depositional Devonian age of the Varied group is questioned thanks to low Sr isotopic ratios which indicate shallow marine environment during Late Proterozoic rather than Palaeozoic [32]. Schulmann et al. [122] interpreted the common occurrence of Late Silurian–Early Devonian zircons in the high grade amphibolites of the Moldanubian domain as a result of important magmatic reworking of the lower part of the continental crust associated with mafic magmatic additions during lithospheric thinning of this domain (Fig. 3middle). In their model, the Monotonous Group represents relic of Proterozoic middle crust that is less affected by thermal and magmatic reworking while the upper crust recorded sedimentary and volcanic evolution related to the Silurian–Devonian extensional event. A back-arc environment is further supported by bimodal volcanic activity in narrow Devonian basins developed on the north-eastern margin of the Brunia continent [107] suggesting only minor thinning of continental crust at the easternmost termination of the back-arc system (Figs. 3middle and 4B). In this concept the rest of the Brunia platform represents a stable continental domain not affected by the back-arc spreading.
The Devonian Saxothuringian oceanic subuduction and onset of continental underthrusting of the Saxothuringian–Armorican lower plate underneath the upper plate is thus recorded in all units forming the present-day Bohemian Massif (Fig. 5). The following contemporaneous processes were identified:
- • eclogitization of the Saxothuringian crust and its exhumation along the Teplá suture;
- • sedimentation of Mid-Devonian distal turbidites of the Srbsko Formation followed by inversion of the fore-arc basin in the Teplá-Barrandian domain, origin of the magmatic arc in the area of the Central Bohemian Plutonic Complex and exhumation of the western metamorphosed margin of the Teplá unit suggesting convergent processes in the upper plate;
- • formation of the back-arc system on the continental lithosphere as shown by isotopic data in the Moldanubian domain and marginally in the Devonian basins affecting western margin of the Brunia continent (here, the formation of small oceanic basin is not excluded). All existing data point out to the subduction process that operated at least 45 million years from 400 to 355 Ma (Fig. 5).
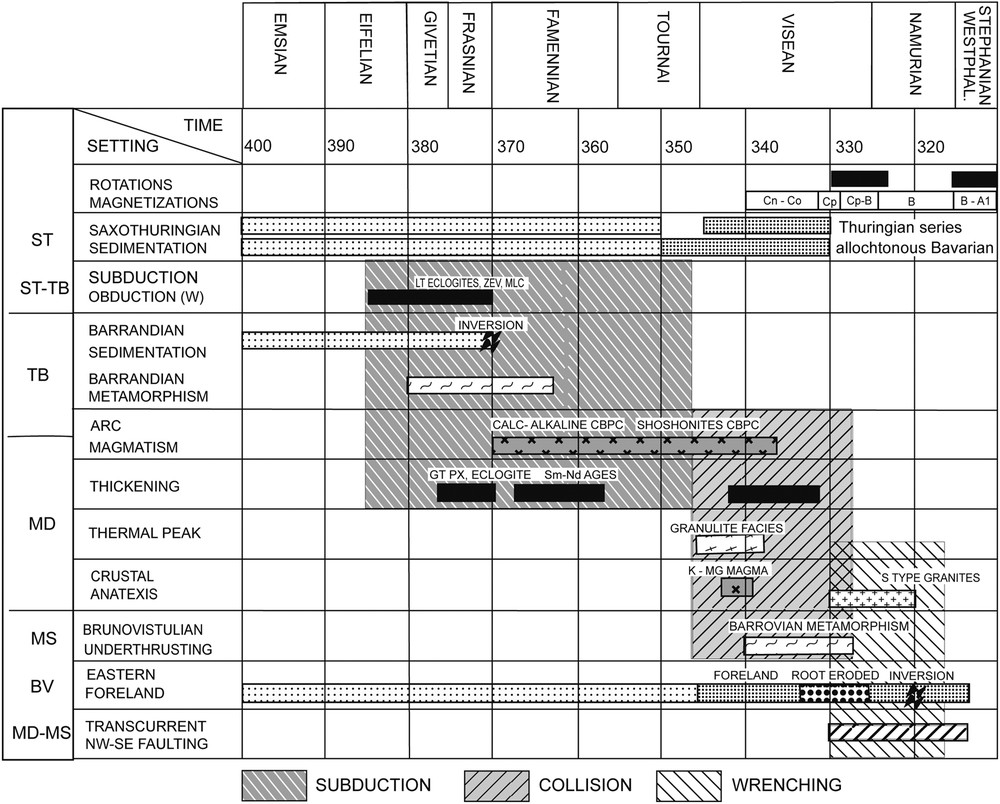
Summary of geochronology data showing the time scales of the three major events forming the Bohemain Massif: oceanic subduction and continental underthrusting of Saxothuringian domain, building of thick orogenic root system and collapse of orogen due to indentation of Brunia.
Fig. 5. Résumé des données chronologiques montrant les échelles de temps des trois évènements majeurs de la formation du Massif de Bohême : subduction océanique et sous-charriage du domaine saxothuringien, édification de l’épais système d’enracinement orogénique et « collapse » de l’orogène, en raison de l’indentation de Brunia.
3.2 The Early Carboniferous crustal thickening of the upper plate
This event is recognized in all units except the Teplá-Barrandian supracrustal unit. The western margin of the Bohemian Massif is characterized by the arrival of the Saxothuringian continental crust and its subduction underneath the eastern Teplá-Barrandian–Moldanubian domain (Fig. 4B). The main thrust boundary migrated further west, so that the continental crust was thrust underneath the fossil Devonian suture and former fore-arc region [71]. At the same time the deformation regime changed in the far field back-arc region (future Moldanubian domain), which recorded the progressive thickening of the whole previously thinned and thermally softened domain as indicated by several recent petrological studies (e.g. [77,128]). Recent structural studies have shown that the earliest preserved fabrics have been sub-horizontal [31,112,123,131], which may indicate that the lower crustal material was originally flowing horizontally from the area of the continental subduction channel towards the region of eastern backstop in a manner proposed by [104] for the closure of the Rhenohercynian Basin and formation of the Mid-German Crystalline Rise.
Indeed, the influx of lower crustal material transported by south-east dipping Saxothuringian continental subduction zone underneath the fore arc (the Teplá-Barrandian domain) and further below the former back-arc domain is regarded to be at the origin of the future “Gföhl Unit”. This hypothesis is in line with the whole-rock geochemical and Sr–Nd isotopic composition as well as the zircon inheritance patterns in the Moldanubian HP–HT granulites [59,65]. Importantly, the crustal material involved in the subduction and extruded over the sub-arc and sub-back-arc mantle lithosphere may have been turned into voluminous HP granulites known from many regions of the Bohemian Massif [59,100]. Such a processing of the lower plate continental lithosphere in a subduction zone and its extrusion above mantle wedge was successfully modelled by Gerya and Stockert [48]. Alternatively, the back-arc domain with high thermal budget inherited from Devonian stretching may have been thickened and the partially molten lower crust may have been transported downwards and transformed into the HP granulites as suggested by Štípská and Powell [129] or Schulmann et al.[122]. However, this model fails to explain the occurrence of the Gföhl gneiss and felsic granulites in the lower crustal position.
The onset of thickening of the root is not recorded in the Teplá domain (Fig. 4C), which behaved as a supra-structural unit at this time, but it is shown by deformation of the Lower Palaeozoic rocks of the Prague basin and adjacent Late Proterozoic rocks. Here, the steep fabric is well dated by syntectonic calc-alkaline plutons at about 355–345 Ma [64,122,158]. In contrast, the eastern sector of the orogen records onset of loading of the Brunia platform (Fig. 4C) during Tournaisian manifested by destruction of the Givetian carbonate platform and sedimentation of coarse basal clastics [49].
The timing of crustal thickening is relatively poorly constrained compared to the subduction and later exhumation processes (Fig. 5). However, the Tournaisian and Early Visean massive clastic sedimentation on both Saxothuringian and Brunia plates suggests load of both continental lithospheric plates and high topography in between them. This corroborates the peak metamorphic ages in the granulites and the Moldanubian eclogites as well as the Visean age of compression of the magmatic arc. All geochronological and other geological information point to a crustal thickening period that was very short and did not last more than 20 million years, from 355 to 335 Ma, with a peak around 340 Ma (Fig. 5).
3.3 Late Visean exhumation of orogenic lower crust of the upper plate
The exhumation of the Variscan lower crust during Early Carboniferous is exemplified by the three NE–SW trending belts of granulites, eclogites and peridotites (Fig. 3c) intimately associated with the (ultra-)potassic magmatites [29,59]. The first granulite belt is represented by narrow strip of felsic granulites that occur at the Saxothuringian–Teplá-Barrandian boundary and it is interpreted as an extrusion of the orogenic lower crust along a large scale crustal shear zone at ∼340 Ma [71,163]. The coeval ∼340 Ma age of the Saxothuringian eclogites and of the felsic granulites [80,150] led Konopásek and Schulmann [71] to propose a model of simultaneous exhumation of nappes derived from the Saxothuringian crust and viscous extrusion of orogenic lower crust from underneath the Teplá-Barrandian supra-crustal unit (Fig. 4D) [36]. The second belt, recognized east of the Central Bohemian Plutonic Complex, i.e., “the magmatic arc”, was exhumed along huge west dipping detachment zone [111,157,158], which was also responsible for collapse of the upper part of the magmatic arc system and the downthrow of the whole Barrandian section [115,157]. Such a huge vertical material transfer (Fig. 4D) could have been responsible for vertical exchange of the lower crustal and upper crustal material in a range of 50 km with final throw of 15 km [110,162]. The cooling ages from the lower crustal domain show that the granulites have crossed the 300 °C isotherm during Carboniferous (∼330–310 Ma) [75,138] suggesting the time at which the lower crustal bulge reached shallow position in the upper plate.
The third lower crustal belt rims the eastern margin of the Bohemian Massif, i.e. the boundary with the Brunia continent (Fig. 4D). Here the granulite fabric is also vertical and interpreted in terms of massive vertical exchanges with orogenic middle crust [123]. The zone of the lower crustal bulge is interpreted as an enormous zone of vertical extrusion surrounded by a middle crust coevally transported downwards in form of a crustal scale synform. The vertical material transfer along the Moldanubian lower crustal belts was a matter of research for several Czech authors during the last five years [31,112,123,131,142]. The model of vertical extrusion is based on the concept of buckling of the lower and mid-crustal interface followed by growth of crustal scale antiforms. This process is thought to be triggered by rheological and thermal instabilities in the arc region, while to the east it is forced by rigid backstop, preserved only locally [130].
However, the most important feature of the eastern Variscan front is the development of horizontal fabrics in the Moldanubian root zone parallel to the Brunia continental margin. The intense deformation of the Brunia continent leading to the formation of the Moravo-Silesian imbricated nappe system was associated with the development of tectonically inverted Barrovian metamorphism (Fig. 4D) and formation of crustal mélange in its upper part (the Moravian Micaschist Zone). These phenomena, as well as mixing of HP rocks and migmatites in the overlying Moldanubian nappe have been recently interpreted in terms of indentation of the Brunia continent into the hot and thick continental root [123]. This lower crustal indentation and flow of hot lower crustal rocks in supracrustal levels are consistent with a model of continental channel flow driven by the arrival of a crustal plunger, a model which is advocated for two decades for the deformation of the Eastern Cordillera in the Andes (e.g. [87]). Finally, the continuous load of the Brunia platform related to deep indentation process led to the development and eastward propagation of the foreland basin. In our model, as the hot Moldanubian rocks advance over the Brunia platform, an imbricated footwall nappe system is generated and thrust over the progressively buried foreland basin rocks.
The time scale of exhumation of the orogenic lower crust is well constrained due to a set of well dated diachronous processes that start with the exhumation of the West-Bohemian granulites, growth of western and eastern orogenic lower crustal antiforms and a shallow channel flow of partially molten lower crust associated with the inversion of the eastern foreland basin. All that is linked with a major thermal event in the mantle as shown by the ages of a syn-extrusion high potassic magmatism, the exhumation of large number of mantle fragments and the overall melting of the Moldanubian crust. The time scale of all these processes was surprisingly short, i.e., about of 20 Ma, and ranges from 335 to 315 Ma (Fig. 5).
4 Palaeographic constraints for Andean type orogeny in the Bohemian Massif
Finger and Steyrer [24] attempted to explain the tectonic processes at the Moldanubian–Moravian boundary with a plate tectonic model involving the subduction of a Silurian–Devonian oceanic domain westward beneath the Moldanubian zone. This concept supposes large rotation of the Brunia platform forming a part of an Old Red continent (Avalonia) around the core of the Bohemian Massif during Visean times [140]. The model of westward subduction is also based on the presence of Silurian MORB-type amphibolites [25] within the Gföhl Unit, which are interpreted as relics of oceanic crust of the Rheic Ocean. In addition, the occurrences of eclogites located along the eastern Variscan front [73,132] can serve as an additional argument for this subduction model.
However, the palaeomagnetic investigations carried out on granitoids of the Central Bohemian Pluton and its extension to the east (the Nasavrky Plutonic Complex [58]) demonstrate that the Teplá-Barrandian, the Moldanubian as well as Brunia domains had a common geodynamic behaviour since the Late Visean (335–330 Ma according to Edel et al. [22]). Therefore, the interpretation of palaeomagnetic directions from Devonian sediments of the Brunovistulian platform [86,98,139] in terms of an oroclinal bending of the Rhenohercynian domain along the Moldanubian core cannot be valid for several reasons:
- • the consistency of these “Devonian” palaeomagnetic directions with Middle-Late Carboniferous directions (Cp directions at 330–325 Ma and B directions at 320–315 Ma, Fig. 6) in the Moldanubian zone suggests that the Devonian sediments overlying the Brunia continent were re-magnetized in Late Variscan time. Consequently, no relative rotation between the Brunia continent and the Moldanubian core can be considered prior to 330 Ma;
- • magnetic overprinting of the Devonian sequences on the Brunia continent is supported by important deformation and burial of Culm and Devonian sediments during Late Carboniferous deformation of the Brunia margin [30,120,126]. Hence, it is unlikely that primary magnetizations could have survived such a severe structural and thermal reworking of the Devonian sediments. We suggest that magnetic overprinting was the result of the Carboniferous tectono-thermal processes associated with the underthrusting of the Brunia platform together with Culm accretionary wedge [11] underneath the hot Moldanubian root zone and with later uplift and erosion of the Moldanubian–Moravian nappe sequence.
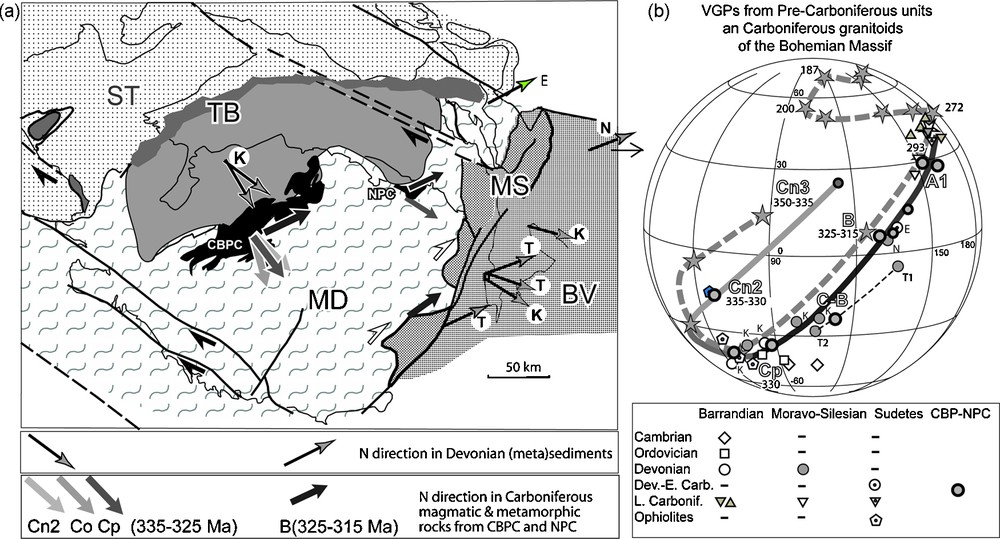
a: paleomagnetic north directions obtained in Devonian (meta)sediments from the Teplá-Barrandian and Brunia zones (K: [86]; N: [98]; T: [139]; E: [21]) and from Early Carboniferous granitoids from Central Bohemian Pluton and Nasavrky Pluton [22]. Cn and Co directions represent magnetizations acquired at the end of the NW–SE shortening and the uplift of the Moldanubian root; Cp and B directions correspond to overprints acquired during NNE–SSW compression and clockwise rotation of the Variscides. Note the similarity of “Devonian” directions with Carboniferous directions; b: published mean virtual geomagnetic poles (VGPs) and associated apparent polar wander curve (grey dashed line) from Early Paleozoic rocks of the Bohemian Massif and mean poles from western Europe Variscides (stars with ages of magnetization) and associated apparent polar wandering curve (dark grey line) [22].
Fig. 6. a : directions du nord paléomagmatique obtenues dans les (méta)sédiments dévoniens des zones Teplá-barrandienne et Brunia (K :[86] ; N :[98] ; T :[139] ; E :[21]) et dans les granitoïdes du Carbonifère inférieur et du Pluton Nasavrky [22]. Les directions Cn et Co représentent les magnétisations acquises à la fin du raccourcissement NW–SE et du soulèvement de la racine moldanubienne ; les directions Cp et B correspondent à des réaimantations acquises durant la compression NNE–SSW et la rotation dans le sens des aiguilles d’une montre des Variscides. À noter, la similarité des directions « dévoniennes » et carbonifères ; b : pôles géomagnétiques virtuels (VGP) publiés, associés à la courbe de dérive apparente des pôles (ligne en tiretés) pour les roches du Paléozoïque inférieur du Massif de Bohême et pôles moyens pour les Variscides d’Europe occidentale (étoiles avec âges de la magnétisation), associés à la courbe de dérive des pôles (ligne continue gris foncé) [22].
The Siluro-Devonian basin in the area of the Moldanubian zone existed as a back-arc basin above the Saxothuringian subduction zone [122] but the question of development of oceanic crust in this area remains a matter of discussions. Therefore, in contrast to the generally accepted rotation of a Brunia continent independent of a stationary Moldanubian domain associated with a closure of a large (Rheic) oceanic domain we propose a model of common geodynamic history of the Bohemian Massif during Devonian and Early Carboniferous (Fig. 5). In our model the palaeomagnetic, crustal scale geophysical and structural data are in favour of an early counterclockwise rotation of composite blocks (Saxothuringian, Barrandian, Moldanubian and Brunia altogether) accommodated by a large scale NW–SE trending dextral wrench zones (such as the Elbe, Pfahl, Franconian and Pays de Bray faults) during Early Visean [22]. These movements could have resulted from northwest drift of Gondwana continental masses and continued after 330 Ma by clockwise rotation of the whole Variscan belt around the Euler pole that was continuously translated westward parallel to the Teysseire–Tornquist zone (southern margin of the Baltica).
Acknowledgements
The French National Science Foundation project “ANR LFO in orogens”, internal research funds of CNRS UMR 7615 and grant MSM0021620855 of the Ministry of Education of the Czech Republic are acknowledged for financial support and salary of Ondrej Lexa. Jiří Konopásek appreciates the financial support of the Grant Agency of the Charles University (project No. B-GEO-270/2006), as well as the support by the Ministry of Education, Youth and Sports of the Czech Republic through the Scientific Centre “Advanced Remedial Technologies and Processes” (identification code 1M0554).