1 Introduction
The high-grade terranes of the Mozambique Belt (Holmes, 1951) are part of the East African Orogen (McWilliams, 1981; Stern, 1994) that formed by continent – continent collision of East and West Gondwana fragments during the Late Neoproterozoic, between ca. 640 and 550 Ma (Collins and Pisarevsky, 2005; Key et al., 1989; Meert, 2003). In Tanzania, the Mozambique Belt constitutes a complex imbricated structural unit reworking pro-parte the Archean Tanzanian craton and the Usagaran Paleoproterozoic belt on its eastern border (Fig. 1). During the last decade, numerous field and laboratory studies in geochemistry, geochronology (Möller et al., 2000; Muhongo et al., 1999; Sommer et al., 2003, 2005) as well as updated geological synthesis at belt scale (Dewey and Burke, 1972; Shackelton, 1993; Deschamps et al., 2004; Pinna et al., 2004) have modified and improved the geological knowledge of the Tanzanian Mozambique Belt (Fig. 1). One of the most famous features of the Mozambique belt in Kenya, Tanzania and Madagascar is the occurrence of a wide variety of densely scattered gemstone deposits along the Mozambique Belt (Keller, 1992), earning it the name of the “Gemstone Belt of East Africa” (Knorring and Condliffe, 1987; Malisa and Muhongo, 1990). Recent research based on worldwide reference deposits have enabled a better understanding of the typology of gem deposits (i.e.: primary deposits [igneous, metamorphic, metasomatic, anatectic], secondary deposits [igneous, sedimentary]) and their genetic emplacement processes, specifically for metamorphic-metasomatic processes in amphibolite and low grade granulite facies (Garnier et al., 2004, 2008; Giuliani et al., 2007; Simonet et al., 2008).

Simplified geological map of north-eastern Tanzania with location of the studied areas: Fig. V. Longido district. Fig. II. Lossogonoi district. 1. Neogene-Quaternary volcanic formation. 2. Neoproterozoic metasedimentary rocks. 3. Granulitic rocks. 4. Undifferentiated gneisses. 5. Archean gneisses. 6. Foliation pattern. 7. Location of ruby deposits (Lossogonoi, Longido, Handeni, Babati, Umba).
Carte géologique simplifiée du Nord-Est de la Tanzanie avec la localisation des districts étudiés : Fig. V. Longido. Fig. II. Lossogonoï. 1. Formations volcaniques néogènes et quaternaires. 2. Roches sédimentaires néoprotérozoïques. 3. Granulites. 4. Gneiss indifférenciés. 5. Gneiss archéens. 6. Trajectoire de foliation. 7. Localisation des gisements de rubis (Lossogonoï, Longido, Handeni, Babati, Umba).
However, concerning Tanzania, detailed geological studies allowing a better understanding of the gemstone mineralizations in their geological, structural, metamorphic context at local and regional scale remain scarce (Malisa, 1987; Malisa and Muhongo, 1990; Mercier et al., 1999; Shackelton, 1993; Simonet, 2000). The present work is thus designed to constrain the geological setting of two major ruby deposits of north-eastern Tanzania, through east-west geological cross-sections and geochronological studies in the Masai steppe.
2 Analytical methods
Zircons were extracted after crushing (< 250 μm) by using standard heavy-liquid and magnetic-separation techniques. Single-zircon dating was carried out using the direct lead evaporation method (Kober, 1986, 1987), according to the detailed technical procedure described by Cocherie et al., 1992. The average 207Pb*/206Pb* age for all steps is given to ±1σ standard deviation on all 207Pb*/206Pb* individual ratios. Where several steps were recorded for a given grain, the “single age” was obtained with a ±1σ standard deviation calculated with all corresponding recorded 207Pb*/206Pb* ages. Finally, where several zircons gave the same age (within the analytical uncertainties) over various steps, a weighted average age based on all the individual steps was calculated with ±2σ (95% confidence limit) using the ISOPLOT/EX program (Ludwig, 1999).
3 The Lossogonoi district
3.1 Geological setting
Despite the occurrence of some of the most famous Tanzanian gemstones producing districts, namely Lossogonoi (ruby), Merelani (tanzanite) and Komolo (tsavorite) (Fig. 2), detailed geological studies in the Masai steppe are scarce (Grainger, 1964; Macfarlane, 1965; Malisa, 1987; Malisa and Muhongo, 1990; Shackelton, 1993). The regional structure is characterized by a large-scale antiform, the Lelatema fold structure, underlined by metasedimentary rocks (quartzites, marbles and pelites). The axial plane of the fold trends NNW and dips towards the east. Depending on the authors, this wide structure was produced either during one (Macfarlane, 1965) or two successive superimposed deformational events (Grainger, 1964; Malisa, 1987). Three main structural units are distinguished from west to east (Fig. 2):
- – Unit 1 comprises essentially layered mafic and felsic granulites including some lenses of metasedimentary rocks (marbles, quartzites, graphitic schists), known more to the west as the “Loibor Serrit granulites”, belonging to the “Central granulites” defined by Hepworth, 1972. According to Grainger, 1964, this unit is affected by a pervasive north-NNE-trending foliation with a moderate dip towards east-SE. Mafic granulites exhibit a garnet + clinopyroxene + hornblende + plagioclase synkinematic assemblage. Using classical geothermobarometers (Ellis and Green, 1979; Holland and Blundy, 1994; Moecher et al., 1988), P-T conditions of metamorphism are estimated at 12–14 kbar, 700 ± 50 °C (Table 1), thus similar in thermometry but higher in barometry than those obtained in the Usambara and Pare mountains, belonging to the “Eastern granulites” (9–10 kbar, 700–800 °C) (Appel et al., 1998). To the east, at the contact with the metasedimentary unit (unit 2), mafic granulites are retrogressed into mylonitic hornblende ± plagioclase-bearing amphibolites, affected by a gently (20°) south-eastward dipping foliation, trending N20°E. This second deformation event occurs under amphibolite facies metamorphic conditions. The related stretching lineation trends N40°E, with a gently (5°) south-westwards plunge; shear criteria indicate a south-westwards thrusting movement.
- – Unit 2 is a metasedimentary unit made of dolomitic marbles, quarzites, graphitic schists and layered amphibolites with a locally migmatitic aspect. This unit is affected by two deformation events:
- (i) the first one, characterized by a foliation parallel to the layering is coeval with a garnet + kyanite/sillimanite + biotite assemblage observed in metapelites or garnet + kyanite + graphite in graphitic schists;
- (ii) the second one is responsible of the refolding of the whole unit (Lelatema fold) and is associated with vertical shear zones.
Close to the contact with unit one, folded pegmatitic veins are associated with a dextral N20 trending shear zone. In Merelani area, located in the hinge of the Lelatema antiform, P-T conditions have been estimated at 7–9 kbar, 600–720 °C (Malisa, 1987) or 5–6 kbar, 610–670 °C (Maboko and Nakamura, 2002). However, relationships between these estimations and the two deformation events are not clearly established. Further east, in SE Kenya (Taita Hills), P-T conditions in metasedimentary units, which constitute the equivalent of the Masai steppe metasediments, are estimated at 10–12 kbar, 800 °C for D1 and 5–8 kbar, 600–650 °C for D2. The first deformation event is dated at 630–645 Ma (Hauzenberger et al., 2007).
- − Unit 3 outcrops in the central part of the Lelatema fold and comprises essentially strongly deformed biotite or hornblende-bearing quartzo-feldspathic gneisses and weakly deformed granitoids, both constituting the surrounding country rocks of the Lossogonoi ruby-bearing veins (Fig. 2). Indeed, in the central part of the area, near Lossogonoi, granitoids present a N40-trending foliation plan bearing a subhorizontal stretching lineation. Shear bands indicate sinistral movements. These mylonitic shears are marked out by ultramafic bodies clusters, one of them being the host rock of the ruby–bearing Lossogonoi amphibolite (“anyolite”) veins (Fig. 2). At the mine scale, a set of four subparallel steeply southward-dipping N60 to N80-trending dark garnet-bearing amphibolite and “anyolite” veins crosscut a serpentinised and carbonatised meta-peridotite body. Most of the sporadic ruby production since 1968 comes from a single thin (ca. 0.5 m thick) dark-green mineralogically zoned ruby ± garnet amphibolite vein, locally grading to a zoisite-bearing “anyolite” – a local name indicating a rock mainly made up of black hornblende, green zoisite and red corundum (Simonet, 2000). Gem-quality ruby occurs as xenomorphous crystals in occasional pockets, mostly associated to dark green amphibole facies, more rarely with zoisite.
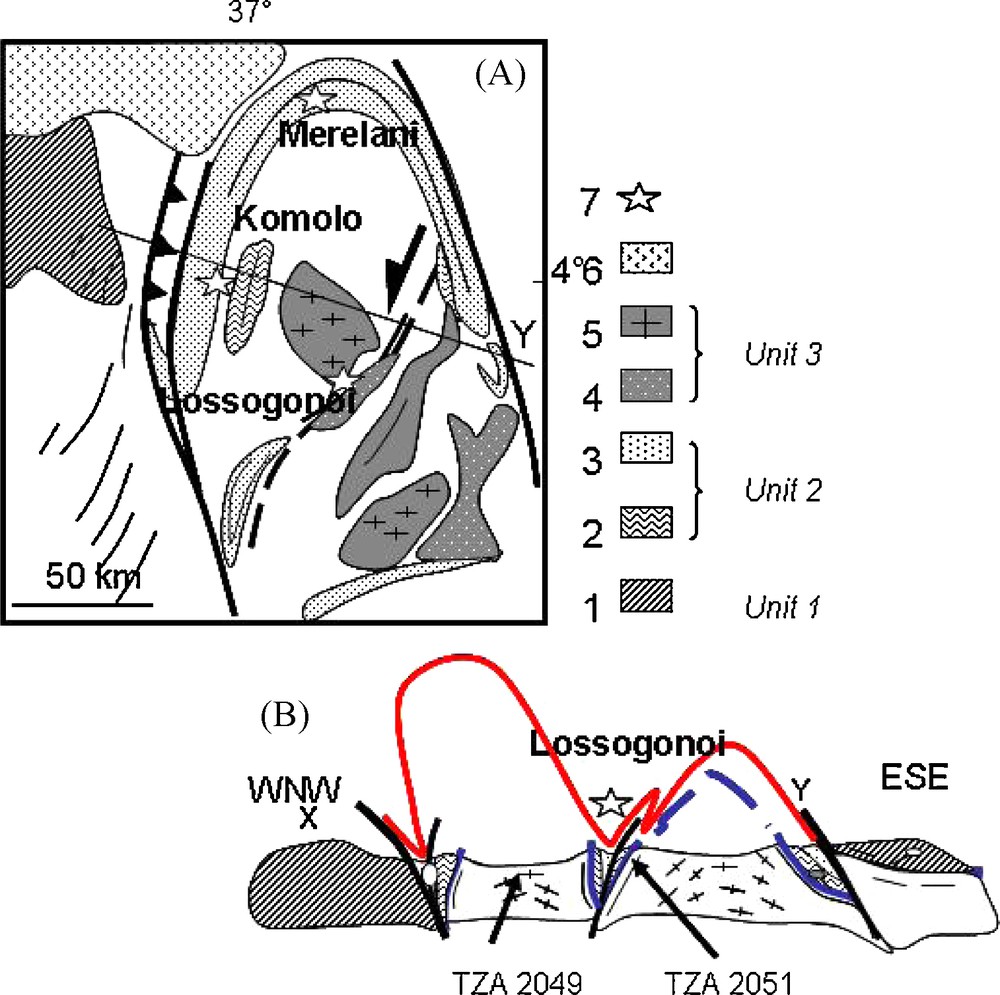
A. Simplified geological map. B. Cross-section of the Lossogonoi district and the Lelatema fold structure in the Masai steppe (Lossogonoi district): 1. Granulitic unit (Loibor Serrit granulite). 2. Migmatitic mafic rocks. 3. Metasedimentary formation. 4. Layered quartzo-feldspathic formation. 5. Layered gneisses and granitic formations, weakly deformed. 6. Cenozoic Rift volcanics. 7. Location of tsavorite deposit (Komolo), tanzanite deposit (Merelani), ruby deposit (Lossogonoi).
A. Carte géologique simplifiée. B. Coupe de la structure plissée de Lelatema dans la steppe Masai (district de Lossogonoï) : 1. Unité granulitique (granulite de Loibor Serrit). 2. Roches basiques migmatitiques. 3. Formation métasédimentaire. 4. Formation quatzofeldspathique litée. 5. Gneiss lités et granites peu déformés. 6. Volcanites cénozoïques du Rift. 7. Localisation des gîtes à tsavorite (Komolo), tanzanite (Merelani) et rubis (Lossogonoi).
Géothermobarométrie des granulites de Loibor Serrit à grenat et clinopyroxène.
Geothermobarometers after | Sample 2030 | Sample 2031 |
Ellis and Green, 1979 (°C) | 620 ± 20 | 650 ± 40 |
Holland and Blundy, 1994 (°C) | 750 ± 20 | 820 ± 20 |
Moecher et al., 1988, at 650–750 °C (kbar) | 13–15 | 12–14 |
3.2 Geochronology
Single-zircon dating was carried out on two samples of unit 3:
- – Sample TZ2049 (3°56.894’ S/36°59.213’ E) is a widely outcropping weakly deformed pink porphyritic granite. Porphyroclasts of perthitic K-feldspar + plagioclase + quartz and hornblende are partly recrystallized in a fine-grained granoblastic and synkinematic microcline + plagioclase + quartz + garnet + biotite assemblage. Zircons are numerous, anhedral to sometimes subeuhedral, and of relatively large size (200–500 μm). They are yellow-orange to amber in colour, generally elongated, more or less translucent and frequently present a strong concentric zonation and numerous inclusions. Four zircons selected amongst the most translucent and well-crystallized grains have been analyzed, giving four to seven temperature steps (Table 2). The ages of the first steps are frequently younger than the higher temperature steps (three first steps of zircons A and B). The five steps of zircon C are very homogeneous (2635 Ma) and similar to ages obtained for the last steps of zircon A and the four last steps of zircon B. In contrast, the zircon D is always slightly younger with ages growing with temperature. The mean age, 2636.1 ± 2.4 Ma, calculated on ten temperature steps (Fig. 3) is interpreted as the emplacement age of the granitic protolith (Neoarchean).
- – Sample TZ2051 (4°02.816’ S/37°07.587’ E) belongs to outcrops located close to the ultramafic body hosting the Lossogonoi ruby-mine. These rocks are garnet-biotite-bearing migmatitic gneisses affected by a N115-trending foliation dipping 50° to the southwest. Foliation plane exhibits a N220-trending stretching lineation plunging 40° to the southwest. Some hundred meters farther, similar rocks present a mylonitic texture associated to a subvertical foliation plan, with a lineation pitch of 50° towards the northeast. We interpret the granoblastic plagioclase + microcline + biotite ± garnet assemblage as a synkinematic metamorphic assemblage. Some relics of quartz and perthitic K-feldspar porphyroclasts are however still preserved. The numerous and relatively large (200–500 μm) zircons are classified in two populations:
- (i) the first one consists of elongated crystals with concentric zonations, multifaceted overgrowth on the pyramids, smoky and yellowish-brownish coloured;
- (ii) the second population corresponds to rounded to ovoid zircons, internally homogeneous, translucent and pinkish coloured.
Amongst analysed zircons, three of them (A, B, E) belong to the first population and four other translucent crystals (C, D, F, G) belong to the second one.
Données isotopiques obtenues par évaporation du plomb sur monozircon de Tanzanie.
Sample | Zircon | T°C | Number of ratios | 206Pb/204Pb | 208Pb/206Pb | 207Pb*/206Pb* ± 1σ | Step age ± 1σ | Zircon age ± 1σ | Mean age ± 2σ |
TZ2049 | Zr A | 1420 | 8 | 9740 | 0.076 | 0.17469 ± 141 | 2589.6 ± 13.5 | ||
Porphyritic metagranite | 1440 | 70 | 16730 | 0.077 | 0.17576 ± 52 | 2608.3 ± 5.0 | |||
Lossogonoi | 1460 | 68 | 32050 | 0.089 | 0.17694 ± 27 | 2621.8 ± 2.5 | |||
1480 | 19 | 23310 | 0.106 | 0.17812 ± 47 | 2631.0 ± 4.4 | ||||
1500 | 72 | 45520 | 0.144 | 0.17870 ± 61 | 2635.1 ± 5.7 | 2634.3 ± 5.7 | |||
Zr B | 1400 | 13 | 5110 | 0.113 | 0.17422 ± 115 | 2587.6 ± 11.0 | |||
1420 | 64 | 37070 | 0.090 | 0.17702 ± 27 | 2622.6 ± 2.5 | ||||
1440 | 72 | 41620 | 0.104 | 0.17694 ± 31 | 2621.5 ± 2.9 | ||||
1460 | 72 | 52920 | 0.110 | 0.17867 ± 27 | 2638.1 ± 2.5 | ||||
1480 | 54 | 47160 | 0.133 | 0.17892 ± 32 | 2640.0 ± 3.0 | 2636.1 ± 2.4 | |||
1500 | 48 | 71660 | 0.163 | 0.17824 ± 32 | 2633.5 ± 3.0 | 2636.0 ± 4.1 | 10 steps | ||
1520 | 51 | 71540 | 0.159 | 0.17815 ± 49 | 2631.2 ± 4.6 | MSWD = 1.2 | |||
Zr C | 1400 | 72 | 88400 | 0.086 | 0.17806 ± 30 | 2632.0 ± 2.8 | |||
1420 | 72 | 55490 | 0.089 | 0.17827 ± 29 | 2634.0 ± 2.8 | ||||
1440 | 54 | 46030 | 0.117 | 0.17887 ± 31 | 2639.5 ± 2.9 | 2635.3 ± 4.1 | |||
1460 | 31 | 106040 | 0.142 | 0.17873 ± 27 | 2638.7 ± 2.5 | ||||
1480 | 7 | 15120 | 0.095 | 0.17907 ± 76 | 2637.2 ± 7.1 | ||||
Zr D | 1400 | 12 | 12200 | 0.082 | 0.17571 ± 102 | 2603.1 ± 9.7 | |||
1420 | 70 | 64320 | 0.108 | 0.17633 ± 72 | 2611.9 ± 6.8 | ||||
1440 | 67 | 88410 | 0.143 | 0.17681 ± 35 | 2619.8 ± 3.3 | ||||
1460 | 62 | 49690 | 0.154 | 0.17719 ± 27 | 2624.2 ± 2.5 | ||||
TZ2051 | Zr A | 1400 | 20 | 7930 | 0.065 | 0.15886 ± 83 | 2434.7 ± 8.9 | ||
Biotite-garnet felsic migmatite Lossogonoi | 1420 | 69 | 27900 | 0.064 | 0.17081 ± 44 | 2561.3 ± 4.3 | |||
1440 | 22 | 20080 | 0.056 | 0.17188 ± 34 | 2572.8 ± 3.3 | ||||
1460 | 14 | 15270 | 0.049 | 0.17242 ± 42 | 2577.2 ± 4.1 | ||||
1480 | 55 | 74430 | 0.045 | 0.17362 ± 59 | 2587.1 ± 5.7 | ||||
1500 | 59 | 49180 | 0.045 | 0.17360 ± 53 | 2587.6 ± 5.1 | 2587.4 ± 5.4 | |||
Zr B | 1400 | 58 | 3560 | 0.090 | 0.12622 ± 234 | 2012.8 ± 33.2 | |||
Elongated zircons | 1420 | 70 | 12800 | 0.060 | 0.13664 ± 110 | 2171.0 ± 14.1 | |||
1440 | 72 | 11600 | 0.063 | 0.15130 ± 94 | 2350.0 ± 10.7 | 2590.1 ± 4.1 | |||
1460 | 69 | 25330 | 0.076 | 0.16715 ± 102 | 2519.0 ± 10.3 | 4 steps | |||
1480 | 71 | 23950 | 0.084 | 0.16835 ± 33 | 2538.1 ± 3.3 | MSWD = 0.3 | |||
1500 | 67 | 12190 | 0.105 | 0.16866 ± 24 | 2542.0 ± 2.3 | ||||
1520 | 66 | 19550 | 0.109 | 0.17384 ± 34 | 2591.7 ± 3.3 | 2591.2 ± 3.7 | |||
1540 | 69 | 77520 | 0.096 | 0.17383 ± 42 | 2590.8 ± 4.1 | ||||
Zr E | 1400 | 69 | 40300 | 0.055 | 0.16482 ± 48 | 2500.8 ± 4.9 | |||
1420 | 69 | 68170 | 0.060 | 0.16936 ± 44 | 2547.0 ± 4.3 | ||||
1440 | 40 | 179880 | 0.055 | 0.17165 ± 28 | 2571.0 ± 2.8 | ||||
1460 | 38 | 9140 | 0.066 | 0.17193 ± 31 | 2573.5 ± 3.1 | ||||
Zr C | 1440 | 30 | 1390 | 0.048 | 0.06452 ± 134 | 714.3 ± 44.3 | |||
1460 | 67 | 33570 | 0.023 | 0.06261 ± 29 | 685.2 ± 9.8 | ||||
1480 | 57 | 5590 | 0.028 | 0.06667 ± 37 | 815.9 ± 11.5 | ||||
1500 | 63 | 10620 | 0.025 | 0.06775 ± 34 | 850.6 ± 10.4 | ||||
1520 | 66 | 27120 | 0.021 | 0.06896 ± 23 | 890.7 ± 6.8 | ||||
1540 | 31 | 34050 | 0.018 | 0.06970 ± 38 | 908.2 ± 11.3 | ||||
Zr D | 1440 | 54 | 26760 | 0.027 | 0.06051 ± 33 | 610.2 ± 11.7 | |||
Round-shape zircons | 1460 | 34 | 44750 | 0.026 | 0.06060 ± 26 | 615.9 ± 9.2 | |||
1480 | 58 | 84490 | 0.023 | 0.06024 ± 19 | 605.2 ± 7.0 | ||||
1500 | 68 | 22130 | 0.024 | 0.06044 ± 21 | 611.6 ± 7.6 | 609.8 ± 9.6 | |||
1520 | 61 | 16140 | 0.024 | 0.06038 ± 25 | 608.2 ± 9.0 | ||||
1540 | 58 | 20730 | 0.023 | 0.06045 ± 28 | 609.7 ± 10.0 | ||||
Zr F | 1460 | 6 | 2290 | 0.019 | 0.05944 ± 91 | 549.5 ± 33.7 | |||
Zr G | 1420 | 17 | 6470 | 0.020 | 0.05927 ± 42 | 561.6 ± 15.4 | 609.5 ± 5.6 | ||
1440 | 8 | 1680 | 0.034 | 0.05980 ± 61 | 574.1 ± 22.4 | 10 steps | |||
1460 | 27 | 4670 | 0.025 | 0.06034 ± 28 | 605.9 ± 9.9 | MSWD = 0.1 | |||
1480 | 38 | 5490 | 0.026 | 0.06042 ± 24 | 610.3 ± 8.5 | ||||
1500 | 65 | 12920 | 0.020 | 0.06050 ± 28 | 611.6 ± 9.9 | 609.5 ± 10.4 | |||
1520 | 61 | 8380 | 0.021 | 0.06045 ± 32 | 608.2 ± 11.6 | ||||
1540 | 8 | 1950 | 0.039 | 0.06062 ± 26 | 616.5 ± 9.3 | ||||
1560 | 8 | 5580 | 0.020 | 0.06047 ± 26 | 611.1 ± 9.2 | ||||
TZ2035 | Zr A | 1400 | 40 | 5630 | 0.110 | 0.14710 ± 79 | 2303.1 ± 9.3 | ||
Garnet-bearing felsic orthogneiss | 1420 | 71 | 22490 | 0.111 | 0.15255 ± 32 | 2371.2 ± 3.6 | |||
1440 | 68 | 14180 | 0.116 | 0.15333 ± 29 | 2380.1 ± 3.2 | ||||
1460 | 60 | 14230 | 0.138 | 0.16090 ± 113 | 2453.2 ± 11.9 | ||||
1480 | 46 | 13830 | 0.140 | 0.16045 ± 43 | 2455.9 ± 4.5 | 2454.4 ± 9.5 | |||
Longido | Zr B | 1400 | 60 | 8690 | 0.102 | 0.13959 ± 209 | 2196.0 ± 26.2 | ||
1420 | 39 | 16290 | 0.103 | 0.14177 ± 196 | 2224.8 ± 24.1 | ||||
1440 | 64 | 21940 | 0.115 | 0.15469 ± 82 | 2389.4 ± 9.0 | ||||
1460 | 18 | 24650 | 0.120 | 0.15655 ± 111 | 2406.7 ± 12.1 | ||||
1480 | 66 | 7610 | 0.123 | 0.15791 ± 56 | 2427.4 ± 6.0 | ||||
1500 | 13 | 26800 | 0.128 | 0.16041 ± 115 | 2447.8 ± 12.2 | 2447.8 ± 4.4 | |||
1520 | 54 | 53580 | 0.127 | 0.15979 ± 63 | 2446.8 ± 6.7 | 2446.0 ± 6.9 | 9 steps | ||
1540 | 54 | 41000 | 0.124 | 0.15943 ± 46 | 2444.8 ± 4.9 | MSWD = 0.7 | |||
Zr C | 1400 | 31 | 2180 | 0.077 | 0.09516 ± 89 | 1513.6 ± 17.7 | |||
1420 | 61 | 4010 | 0.088 | 0.11543 ± 196 | 1855.7 ± 30.9 | ||||
1440 | 68 | 6080 | 0.101 | 0.14076 ± 78 | 2226.9 ± 9.6 | ||||
1460 | 70 | 10070 | 0.119 | 0.15395 ± 63 | 2383.2 ± 7.0 | ||||
1480 | 68 | 21070 | 0.121 | 0.15742 ± 66 | 2421.0 ± 7.2 | ||||
1500 | 69 | 21610 | 0.124 | 0.15688 ± 59 | 2415.9 ± 6.4 | ||||
1520 | 40 | 6070 | 0.123 | 0.15910 ± 43 | 2441.5 ± 4.5 | ||||
1540 | 62 | 7170 | 0.128 | 0.16041 ± 95 | 2450.0 ± 10.0 | 2446.5 ± 9.4 | |||
Zr D | 1400 | 39 | 4000 | 0.108 | 0.13753 ± 116 | 2181.6 ± 14.8 | |||
1420 | 7 | 6350 | 0.107 | 0.14772 ± 85 | 2309.7 ± 10.0 | ||||
1440 | 7 | 8320 | 0.118 | 0.15606 ± 70 | 2405.8 ± 7.7 | ||||
1460 | 27 | 25980 | 0.124 | 0.16036 ± 105 | 2448.3 ± 11.0 | ||||
1480 | 20 | 12950 | 0.124 | 0.16033 ± 107 | 2447.8 ± 11.3 | 2448.09 ± 11.2 |
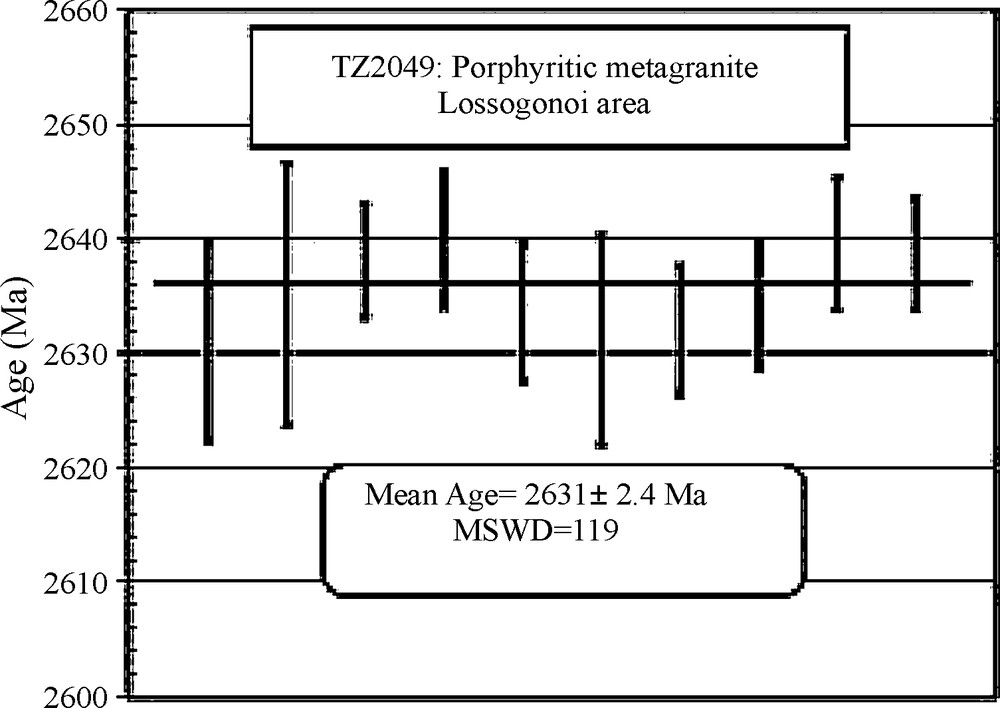
Diagram of temperature step vs 207Pb/206Pb age for the zircons of sample TZ2049, porphyritic metagranite, Lossogonoi area.
Diagramme de paliers de température vs l’âge de 207Pb/206Pb des zircons de l’échantillon TZ2049, métagranite porphyrique, région de Lossogonoï.
Elongated zircons of population (i) are characterized by four to eight successive temperature steps (Table 2). The ages of the first steps are frequently younger than high temperature steps, but all ages are old. A “plateau” seems to be reached with the two latest steps of zircons A and B around 2590 Ma. The zircon E is always younger with a maximum age at 2570 Ma.
The ovoid zircons of population (ii) deliver one to eight temperature steps (Table 2). Zircon C, intermediate between rounded and elongated types, indicates more recent ages than elongated zircons, but increasing correlatively with temperature from 700 to 910 Ma. This is interpreted as a recent recrystallisation around an older core. Other zircons (specially D and G) give homogeneous 610 Ma ages on several temperature steps (Table 2).
Two ages are thus obtained:
- (i) Neoarchean ages issued from elongated zircons (mean age of 2590.1 ± 4.1 Ma, Fig. 4A) which are interpreted as the protolith age (or minimum protolith age) of the migmatitic gneisses;
- (ii) Late Neoproterozoic ages (mean age 609.5 ± 5.6 Ma, Fig. 4B) on 10 steps from two rounded zircons, characterized by low 206Pb/208Pb ratios (> 0.03) that are typical for newly formed metamorphic zircons.

Diagram of temperature step vs 207Pb/206Pb age for the zircons of samples TZ2051, biotite-garnet felsic migmatite, Lossogonoi area. A. Elongated and zoned magmatic zircons. B. Multifaceted and round-shaped metamorphic zircons.
Diagramme de paliers de température vs l’âge de 207Pb/206Pb des zircons de l’échantillon TZ2051, migmatite feldspathique à biotite grenat. A. Zircons magmatiques allongés et zonés. B. Zircons métamorphiques arrondis et multifacettés.
These ages are interpretated as representative of the metamorphic recristallization of the layered gneiss, coeval with the zircon neoformation.
Two events are thus defined: a Neoarchean magmatic event at ≥ 2590.1 ± 4.1 Ma and a Late Neoproterozoic (Pan-african) metamorphic event at 609.5 ± 5.6 Ma, which also corresponds to the age of ruby formation (see discussion).
4 The Longido district
The Longido district (Fig. 1V) correspond to a cluster of ruby-bearing “anyolite” occurrences (Guest and Pickering, 1966; Hartley, 1965) hosted in typically small serpentinized ultramafic bodies scattered within high-grade metamorphic and migmatitic rocks of the Mozambique Belt. Metasediments are dominant, including pelitic and psammitic compositions (quartzo-feldspathic gneisses), aluminous terms (biotite-kyanite-garnet gneisses), quartzitic layers and local thin marble bands. Hornblende-gneisses and amphibolites layers are less common (Guest and Pickering, 1966; Hartley, 1965). Foliation with a dominant NNE-SSW trend and a nearly subvertical dip is associated with large-scale isoclinal folds plunging at shallow angles to the ENE or northeast and sinistral and dextral shear zones, where ultramafic bodies hosting ruby-veins are preferentially hosted. A later folding event associated to open folds is evidenced by complex patterns of metamorphic foliation at map and outcrop scale (Fig. 5).
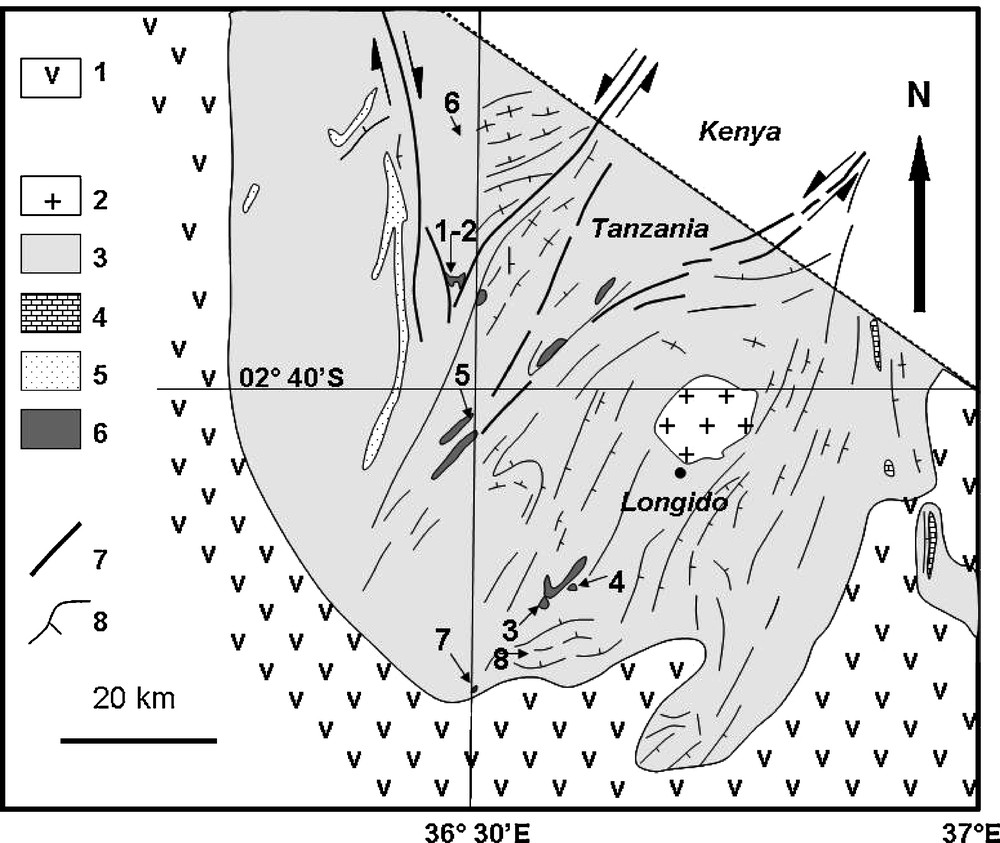
Simplified geological map of Longido area and location of ruby-deposits. Legend of the geological map: 1-Neogene Rift volcanics. 2–6. Neoproterozoic Mozambique Belt: 2. Late Pan-African granite. 3. Undifferentiated gneiss. 4. Marble. 5. Quartzite. 6. Serpentinite. Structural elements: 7. Fault with kinematics. 8. Foliation pattern. “Anyolite” occurrences: 1. Mundarara mine. 2. Mula mine. 3. Lomwinyi. 4. Elkunulesilali. 5. Ketumbeine. 6. Engare Naibor. 7. Lolkinyoyo. 8. Kimasarua.
Carte géologique simplifiée de la région de Longido et localisation des gîtes à rubis. Légende de la carte géologique :1. Volcanites néogènes du Rift. 2–6. Ceinture néoprotérozoïque de Mozambique: 2. Granite tardipanafrican. 3. Gneiss indifférenciés. 4. Marbres. 5. Quartzite. 6. Serpentinite. Eléments structuraux : 7. Faille avec cinématique. 8. Trajectoires de la foliation. Indices d’« anyolite » : 1. Mine de Mundarara. 2. Mine de Mula. 3. Lomwinyi. 4. Elkunulesilali. 5. Ketumbeine. 6. Engare Naibor. 7. Lolkinyoyo. 8. Kimasarua.
Detailed study of mineralisation and its geological environment was focused on the main current ruby-producing site, namely the Mundarara underground mine (X = 36° 28.421’E; Y = 2° 37.876'S) and the very close artisanal Mula mine, of similar geological characteristics (36° 28.219’ E; 2° 38.014'S) (Fig. 6). Ruby ore (“anyolite”) occurs in both mines as single foliated and transversally zoned “veins” – actually very flat (< 1 m thick for ca. 150 to 250 m lateral extension and down dip in the Mundarara mine) pinch and swell lenses – boudinaged in the regional metamorphic foliation. Field and thin section structural arguments bring to interpret “anyolite” emplacement and ruby crystallisation as the result of synmetamorphic – metasomatic processes in an open system.
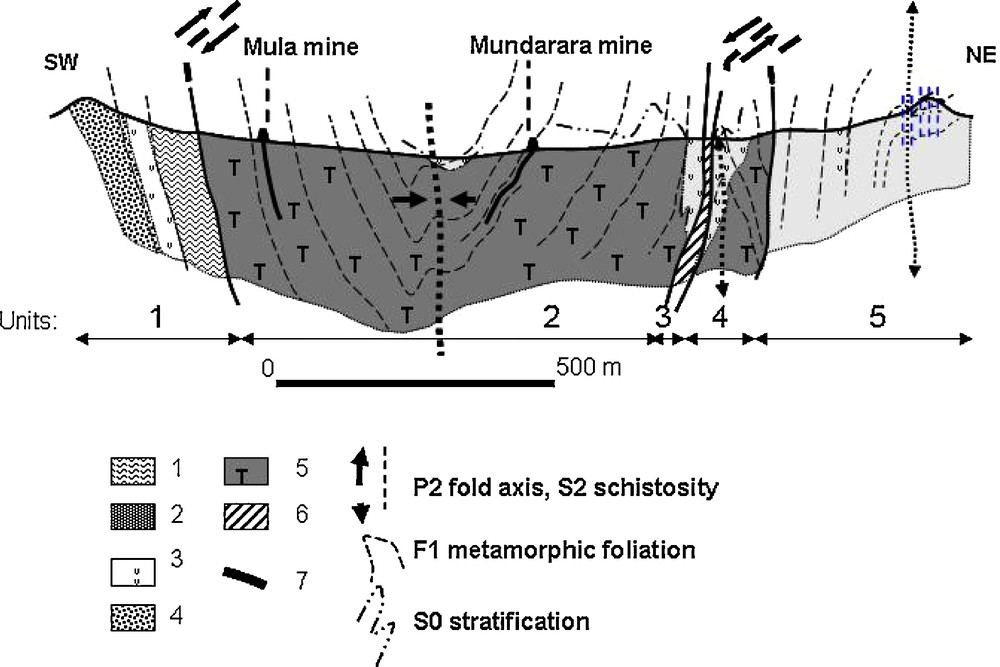
Geological cross-section of Mula and Mundarara mines in Longido district. 1: Migmatic paragneiss (biotite + garnite ± kyanite); 2: marble lens; 3: amphibolite, calc-silicate gneiss; 4: quartzo-feldspathic gneiss; 5: serpentinite; 6: orthogneiss; 7: anyolite vein.
Coupe géologique des mines à rubis de Mula et Mundarara dans le district de Longido. 1 : paragneiss mignatique à biotite + granat ± disthène ; 2 : marbre ; 3 : amphibolite, gneiss calco-silicaté ; 4 : gneiss quartzofeldspathique ; 5 : serpentinite ; 6 : orthogneiss ; 7 : veine d’anyolite.
Local structure involves several lithotectonic units separated by steeply-dipping north-south to NNE-SSW syn-metamorphic ductile strike-slip shear faults (Fig. 6). A highly-deformed serpentinized ultramafic body of harzburgitic origin (Ketto, 2008; Simonet, 2000), host of the ruby-bearing anyolite veins, geometrically overlain by coarse-grained massive to foliated orthoamphibolites constitute the central ultramafic-mafic units (2 and 4, Fig. 6). Synkinematic garnet + plagioclase + hornblende ± quartz, garnet + clinopyroxene + plagioclase + hornblende + quartz or clinopyroxene + plagioclase + hornblende + quartz assemblages in metabasites bring to P-T conditions of deformation of 12–13 kbar and 670–720 °C (Ketto, 2008). A narrow and discontinuous submeridian tectonic slice (unit 3, Fig. 6) of banded leucocratic orthogneiss is intercalated between the ultramafic – mafic units 2 and 4. The contact is clearly identified as a major subvertical sinistral-normal shear fault. Both sides of the mafic-ultramafic units are metasedimentary units, made of dominant aluminous biotite-garnet ± kyanite migmatitic paragneiss, leucocratic quartzo-feldspathic gneiss, calc-silicate clinopyroxene gneiss layers, amphibolite layers and thin impure marble levels, corresponding to the regional metasedimentary series of Guest and Pickering, 1966; Hartley, 1965. The syn-metamorphic dextral shear-fault nature of both contacts between metasedimentary and ultramafic-mafic units is evidenced by the mylonitic character of paragneisses foliation and clear dextral shear criteria. Close to the contact, mylonitic metapelitic rocks are retrogressed in a biotite-bearing synkinematic assemblage. Late post-foliation north-south to NW-SE trending upright open folds with subhorizontal axis affect all units at different scales, inducing medium-to-large-scale perturbations of the metamorphic foliation pattern (Fig. 6).
4.1 Geochronology
Sample TZ2035B (X = 36° 28.593’ E/Y = 02° 37.891’ S) is a garnet-bearing felsic orthogneiss representative of unit 3 (Fig. 6). The orthogneiss exhibits a centimetre-scale alternance of hornblende + plagioclase + quartz ± clinopyroxene, hornblende + plagioclase + garnet + quartz and garnet + hornblende + K-feldspar + quartz layers. Garnet is never in contact with clinopyroxene.
Zircons are rather big (300–800 μm), slightly pinkish to smoky, elongated, sometimes subeuhedral, and generally translucent. They contain numerous opaque inclusions, numerous cavities, and are frequently fissured. Pyramids very often exhibit multifaceted overgrowths. Four crystals have been analysed, each one delivering from five to eight temperature steps. The resulting ages are reported in Table 2. On all of these zircons, an increase of age is correlated with an increase of temperature, with a maximum range between 1510 and 2450 Ma for zircon C. This reveal important lead losses, either linked to very high uranium content and thus an important deterioration of the crystal lattice during disintegrations, or related to a major metamorphic event. The non-metamict character of zircons, as well as the presence of important overgrowths, favour the second assumption, the metamorphism being able to induce a partial recrystallization of grains and thus episodical lead losses. Age of this event is not constrained. High temperature steps (Table 2) on all crystals are nevertheless homogeneous and several steps on a single zircon grain correspond to a same age at around 2450 Ma. This is confirmed on the two last steps of zircons A, C and D, as well as on the three last steps of zircon B. We thus reach a repetitive plateau on several steps and several zircon grains, which can be considered as representative of the crystallization age of this orthogneiss. The mean weighted age calculated on nine steps (Fig. 7) of 2447.8 ± 4.4 Ma is interpreted as the emplacement age of the orthogneiss protolith (Lower Paleoproterozoic or Siderian).
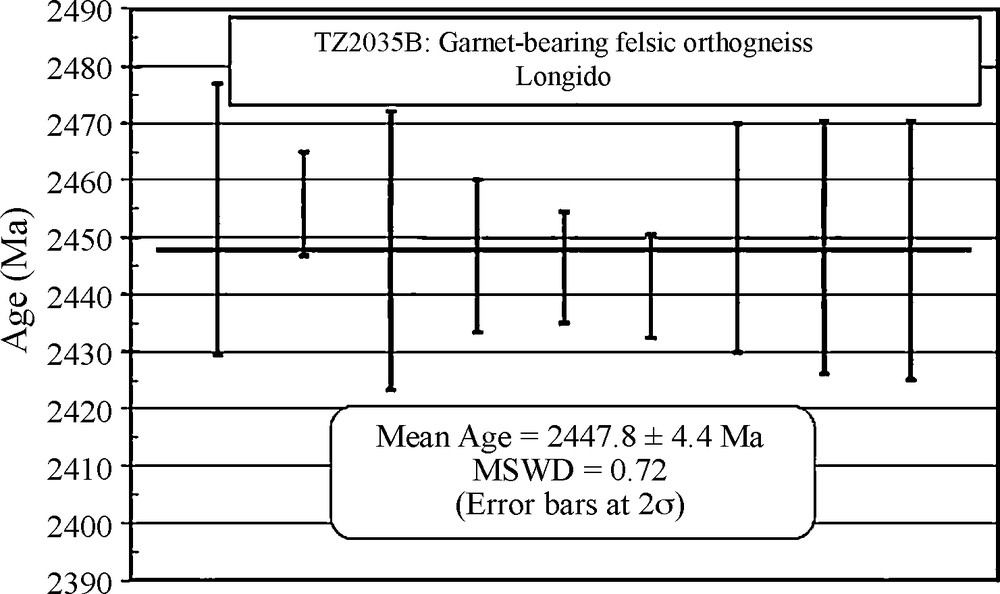
Weighted mean of different 207Pb*/206Pb* ages for retained steps of zircons from sample TZ2035, garnet-bearing felsic orthogneiss, Mundarara mine, Longido.
Moyenne pondérée des différents âges 207Pb*/206Pb* pour les paliers retenus de l’échantillon TZ2035, orthogneiss acide à grenat, mine de Mundarara, Longido.
5 Discussion and conclusion
5.1 Lossogonoi area
Structural relationships between the dated Archean gneisses (this study) and other units in the Masai steppe are poorly documented. According to our field observations, Archean gneisses are overthrusted by metasedimentary units (marbles, quartzites, pelites) – assumed to be of neoproterozoic age, see below – and the Loibor Serrit granulites (units 1 and 2). Both should have been affected by the 640 Ma granulitic metamorphism, if we assume the equivalence between the Masai and Umba steppe metasediments and the Loibor Serrit and Eastern granulites (Pare and Usambara mountains). This hypothesis remains open to verification. Due to the lack of outcrops, the contact between Archean rocks and Neoproterozoic metamorphic units is not observed. Nevertheless, occurrence of granulitic assemblages in Archean gneisses would be a crucial argument to understand initial relationships between Archean and Neoproterozoic units. At the present day, no such assemblage has been observed, but in fact this could be partly due to unfavourable granitic to granodioritic whole-rock compositions. The strong heterogeneity of deformation in the Archean gneisses in comparison with the overlying Neoproterozoic units is also another point to be underlined. Indeed, the sub-isotropic granite dated at 2636.1 ± 2.4 Ma (TZ2049) does not show any younger ages that could be related to a later metamorphic event. In contrast, the mylonitic layered gneisses dated at 2590.1 ± 4.1 Ma, located at the vicinity of a strongly ductile lithology (i.e. serpentinites) reveal a metamorphic recrystallization episode at 610 Ma. In both lithologies, no evidence of the 640 Ma episode of recrystallization is observed. We thus consider that Archean units constitute an old (autochtonous or para-autochtonous?) basement, non-affected by the 640 Ma-old peak granulitic event. Thrusting of Neoproterozoic granulitic nappes, responsible of the exhumation of high-pressure units occurred thus after the peak of granulitic metamorphism dated at 640 Ma, in amphibolite facies conditions. The stacked units were later affected by folding and shearing in amphibolite facies at 610 Ma, during the final shortening event. The 610 Ma shearing deformation event seems to be coeval with the crystallization of ruby in Lossogonoi area. Indeed, shear zones affect both host-rocks and are consistent with deformation and syn-tectonic mineralogical assemblages observed in ruby-bearing veins.
5.2 Longido area
The complex imbrications of lithotectonic units resulting from the Pan-African thrusting, folding and shearing tectonics make difficult the reconstruction of the original succession. A first deformation event is characterized by a pervasive foliation, meanwhile a second one is related to local subvertical shear zones. Two distinct lithostratigraphic packages are evidenced:
- (i) a volcano-sedimentary succession affected by the two deformational events, grading from ultramafic-mafic terms at the bottom towards supracrustals sedimentary argillaceous and detrital sequences. Synkinematic assemblages lead to P-T conditions of D1 deformation in metabasites of 12–13 kbar and 670–720 °C (Ketto, 2008). In aluminous metapelites, this event is expressed by a garnet + kyanite + biotite assemblage, which is retrogressed in biotite-muscovite mylonitic gneiss in D2 shear zone. By comparison with other domains of the Mozambique Belt, this succession is correlated with the Neoproterozoic sequences identified in the upper allochtonous granulitic nappes of the Western granulites and Eastern granulites, (Hauzenberger et al., 2007), as well as in the Mahenge and Morogoro districts (Le Goff et al., 2008), were a maximum age of sedimentation for these platform metasedimentary series was recently constrained at 686 ± 7 Ma by electronic microprobe U-Pb-Th datation (Le Goff et al., 2008).
- (ii) a Lower Proterozoic orthogneissic unit (protolith age 2447 Ma, this study), interpreted as a basement unit slice, exhumated and structurally reworked during the Pan-African tectonometamorphic event. This unit is thus to be correlated with the lower autochtonous or para-autochtonous basement domain of the Mozambique Belt, where extensive occurrences of tonalitic – trondhjemitic and granitic gneisses of Neoarchean (Johnson et al., 2003; Maboko, 1995; Möller et al., 1998; Muhongo et al., 2001; Sommer et al., 2003, 2005), and Paleoproterozoic (Cutten and Johnson, 2006) emplacement ages are well documented.
5.3 Conclusions
This study improves the regional geological knowledge of the Mozambique belt in the north-eastern part of Tanzania and documents for the first time in this area the existence of Archean (2.63 and 2.59 Ga in Lossogonoi) and Paleoproterozoic (2.45 Ga in Longido area) relics reworked during the Pan-African tectono-metamorphic event in this part of the belt. Such an ancient crust has already been evidenced further south in the Magobike region, where SHRIMP U-Pb dating on zircon reveal an Archean (2.7 Ga) age for granitic protoliths (Johnson et al., 2003). Furthermore, several Late Archean Pb-Pb evaporation and SHRIMP U-Pb ages are known on high-grade gneisses from the Mozambique Belt of eastern Tanzania (igneous emplacement ages ranging between 2740 and 2608 Ma) (Muhongo et al., 2001) and 2648 Ma (Sommer et al., 2003). More recently, in the SE Kenya, east of the Galana shear zone, 1500 to 2900 Ma Nd model ages were obtained in migmatitic gneisses (Hauzenberger et al., 2007).
According to our structural and geochronological data, we consider that both Neoproterozoic volcano-plutonic and metasedimentary formations are affected by a first deformation event under high-pressure granulitic conditions at 640 Ma. These stacked units are later thrusted over an old Neoarchean to Lower Paleoproterozoic basement during a second deformation event, under amphibolite facies conditions. Finally all these units are sheared and folded at 610 Ma. During this last stage, deformation is strongly heterogeneous and is characterized by shear zones following highly ductile zone such serpentinites bodies where are located ruby veins at Longido and Lossogonoi districts. In both districts, ruby is coeval with this deformation event. Furthermore, chemical and mineralogical zonations between the ruby vein and the country rocks indicate that a metamorphic-metasomatic process is responsible of the crystallization of ruby as already suggested (Simonet, 2000). Datation of ruby veins (anyolite) has not been investigated. However, in Lossogonoi district, geochronological data on migmatitic gneiss affected by this episode indicate an age of 610 Ma that we interpret as the age of the shear zone and consequently the age of cristallization of ruby.