1 Introduction
The early detection of volcanic unrest mainly relies on the monitoring of volcanic seismicity and ground deformation. These methods provide insights into the dynamics of magma pressurization and transport. However, despite considerable effort, the precise forecasting of eruptions and their intensity has proven to be difficult. Therefore, there is a constant need for novel observational methods to obtain information about the ongoing volcanic processes. Pressurized volcanic fluids (magma, water) or gas induce deformation and thus perturbations of the elastic properties of volcanic edifices. These small perturbations can be detected as changes of seismic wave properties using repetitive seismic sources (Grêt et al. (2005), Ratdomopurbo and Poupinet (1995), Wegler et al. (2006)). However, none of these approaches were apt to provide a continuous monitoring of volcano elastic properties.
Our work relies on both theoretical and applied results, which show that the Green's function between two sensors can be reconstructed from the correlation of seismic noise (see Campillo (2006) for a review). This property has been extensively used to image the Earth's Interior at a global (Nishida et al. (2009)), regional (Shapiro et al. (2005)), or local scale (Brenguier et al. (2007)). In a pioneering work, Sens-Schoenfelder and Wegler (2006) proposed to use the repetitive waveforms of seismic noise cross-correlations to track for subsurface volcanic edifice velocity changes. In this manner, the continuous recording of ambient seismic noise allows continuous monitoring of volcano interiors.
As in any monitoring technique, the time resolution, fixed by the time between two independent measurements, should be as short as possible. This requirement points to a major limitation of the method of monitoring using seismic noise correlations. More precisely, cross-correlations computed from short time series of ambient seismic noise may not have converged to stable functions. The cross-correlation fluctuations spoil measurements of waveform perturbations linked to volcano interior changes. There are two main origins for residual fluctuations observed for successive noise cross-correlation functions:
- • the first is the inadequate intrinsic length of seismic noise record used to converge to a stable cross-correlation function. The length of the seismic noise record required to converge to a stable correlation function is controlled by the nature of the noise, the distance between sensors and by the intrinsic attenuation and scattering properties of the studied medium (Larose et al. (2008));
- • the second is the non-stationarity of ambient seismic noise (e.g., Hadziioannou et al. (2009)). The temporal fluctuations of ambient seismic noise varies depending on the dominant noise sources. These can be seasonal for ocean generated noise sources (e.g., Stehly et al. (2006)) or daily, or weekly for anthropic noise sources (e.g., Bonnefoy-Claudet et al. (2006)).
In the the following, we will describe recent results of temporal monitoring using cross-correlations of ambient seismic noise at Piton de la Fournaise Volcano. We will present techniques that rely on:
- • the estimate of travel time perturbations of coda waves of noise cross-correlations;
- • the measure of decorrelation of cross-correlation functions. These results suggest that monitoring volcanoes using seismic noise should improve our ability to forecast eruptions, their intensity and thus potential environmental impact.
2 Piton de la Fournaise Volcano
Piton de la Fournaise Volcano (PdF) is a hot spot, shield volcano located on La Réunion island in the Indian Ocean (Fig. 1). It erupted more than 30 times between 2000 and 2010. These eruptions lasted from a few hours to a few months and were associated with the emission of mainly basaltic lava with volume ranging from less than one to tens of million cubic meters (Peltier et al. (2009)). The time period we consider (1999–2008) started and ended with 2 major eruptions, namely the March 1998 eruption (60 million of cubic meter of lava emitted) and the April 2007 eruption associated to the 300 m high collapse of the main Dolomieu crater (130 million of cubic meter of lava emitted) (Staudacher et al. (2009)). The intense eruptive activity together with a weak tectonic activity makes Piton de la Fournaise Volcano well suited for studies focused on the processes of magma pressurization and injection and for the development of innovative monitoring methods.
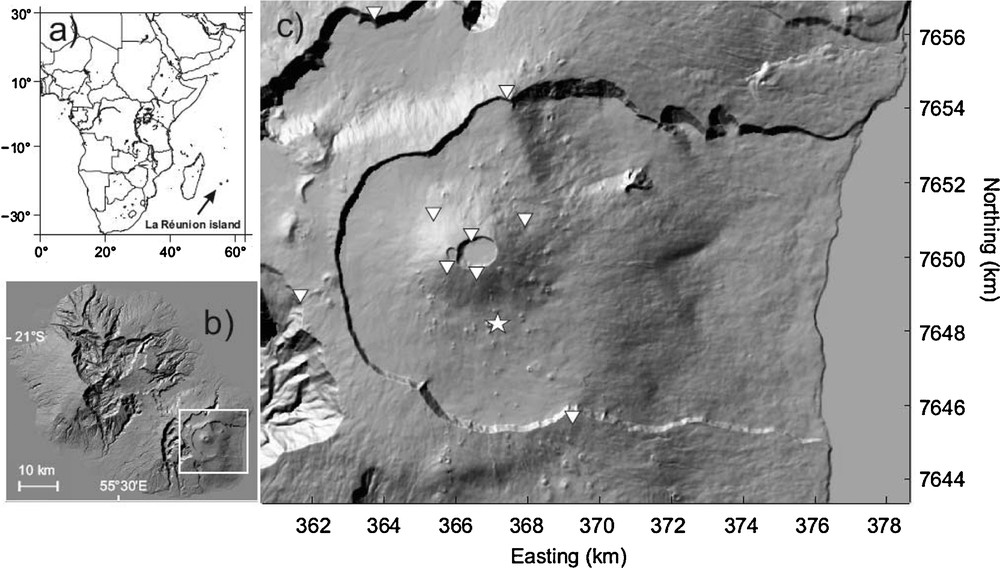
Geographical location of a) La Réunion island, b) Piton de la Fournaise Volcano, and c) the seismic network (inverted triangles) and extensometer (star) used in our analysis.
Localisation : (a) de l’Île de la Réunion, (b) du volcan du Piton de la Fournaise ; du réseau sismique (triangles inversés) et de l’extensomètre (étoile) utilisés dans cette étude.
3 Seismic velocity changes revealed from noise cross-correlations: application to Piton de la Fournaise Volcano
The method we use consists in measurements of very small waveform time delays in the coda of noise cross-correlations. This method is described as the so-called Moving Window Cross Spectrum (Ratdomopurbo and Poupinet (1995), Clarke et al. (2010)) or Coda Wave Interferometry (Snieder et al. (2002)) techniques. Coda waves (late part of seismograms) are scattered waves that travel long distances and thus accumulate time delays as a consequence, for example, of a uniform seismic velocity change in the propagating medium. Measuring travel time perturbations in the coda thus allows detecting very small velocity changes that would not be detectable by a classical measure of first arrival time delays. The drawback of that approach is that it is difficult to estimate the travel path of the scattered waves constituting the coda. However, recent promising results suggest it may be possible to produce refined 3D maps of small changes in a near future (Larose et al. (2010)).
In a previous work (Brenguier et al. (2008)), we analyzed 18 months (July 1999–December 2000) of continuous seismic records from the Piton de la Fournaise (PdF) Volcano Observatory. During the period of study, 5 eruptions occurred lasting on average 26 days each. We applied the Moving Window Cross Spectrum technique between a reference Cross Correlation Function (CCF, stack of 18 months of cross-correlations) and current cross-correlation functions (ten-day-stacks of CCFs, see Brenguier et al. (2008) for details). As a result, we estimate average (for different receiver pairs) relative travel time shifts (Δτ/τ), which is equivalent to the opposite of the medium uniform relative velocity change (Δv/v = −Δτ/τ).
As described by Stehly et al. (2007) and Sens-Schoenfelder (2008), it is important to correct noise records from eventual timing discrepancy because these will perturb the relative travel time shift estimates. Fig. 2a shows the continuous estimates of relative velocity changes. This curve has been obtained by removing the long-term trend (see Brenguier et al. (2008) for details). This plot clearly shows that the volcanic edifice average seismic velocity decreases by ≈ 0.1% before each eruption of PdF Volcano. At the same time, extensometer data (star on Fig. 1 and blue line in Fig. 2 a) only show a precursory opening for the fourth eruption in the series shown in the figure (Peltier et al. (2006)). Fig. 2b shows inter-eruptive volcano-tectonic seismicity (pre-eruptive swarms are excluded). Seismicity is also clearly a precursor of PdF volcanic eruptions but is more diffuse in time than the precursory velocity changes. We interpret precursory velocity decrease as due to the opening of cracks, which is a consequence of the edifice inflation associated with magma pressurization. A similar observation and interpretation has been proposed by Mordret et al. (2010) for the 2006 eruption of Mt Ruapehu (New-Zealand). Following this approach, Duputel et al. (2009) compared the estimates of seismic velocity changes to deformation recorded by GPS for recent eruptions of PdF Volcano.
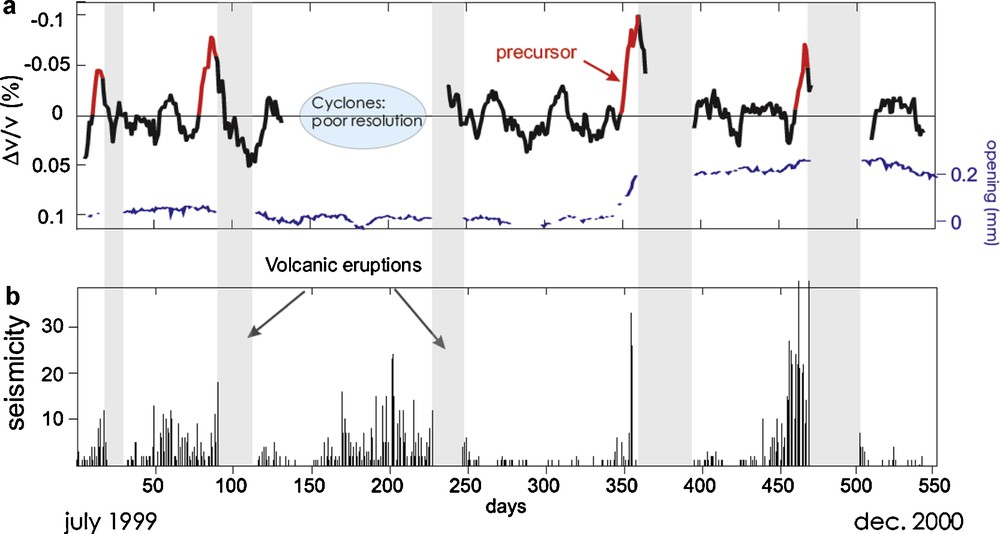
a) Relative velocity changes compared to extensometer (FORX). The travel time shifts are measured in the frequency range 0.1-0.9 Hz. For details, see Brenguier et al. (2008) b) Inter-eruptive seismicity (pre-eruptive swarms are excluded).
(a) Variations relatives de vitesse, comparées à l’extensomètre (FORX). Les décalages de temps de parcours sont mesurés dans la gamme de fréquence 0,1-0,9 Hz. Pour plus de détails, voir Brenguier et al. (2008). (b) Sismicité inter-éruptive (les essaims sismiques pré-éruptifs sont exclus).
Furthermore, to improve the accuracy and thus time resolution of velocity measurements, Baig et al. (2009) developed a cross-correlation filtering method based on time-frequency transforms and phase coherence filtering. This method allowed the detection of a clear decrease in velocity before the June eruption of PdF Volcano (fourth eruption in Fig. 2) with a time resolution as small as one day.
Recently, we computed the velocity change time series for 10 years (1999–2008) during which 28 eruptions occurred at PdF Volcano. The entire time series of velocity change will be presented in a joint paper (in preparation). However, as a preliminary result, we observe velocity decreases that are not associated with eruptions (Fig. 3). These periods are also associated with elevated seismicity. It is interesting to note that these transients last longer (1 or 2 months) than the previously reported pre-eruptive velocity decreases (few days). We thus propose that these transient velocity changes are due to long-lasting intrusions of magma not accompanied by eruptive activity or to pressure buildup associated with the replenishing of the magma reservoir.
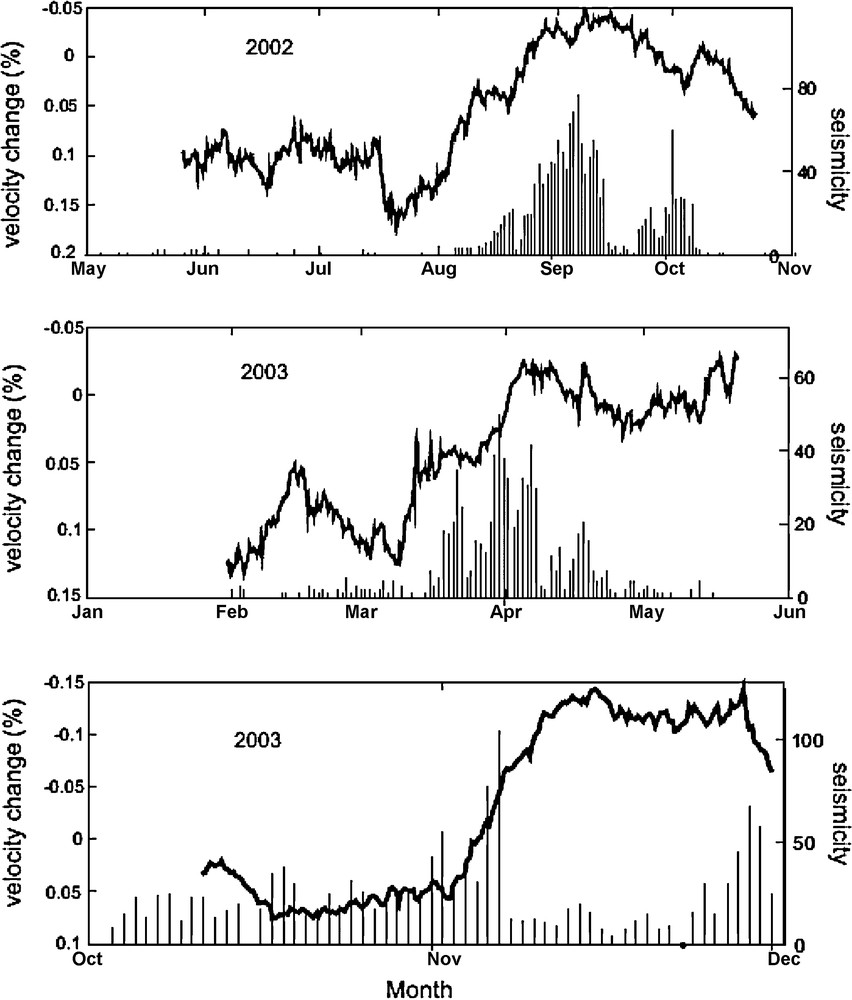
Seismic velocity changes at PdF Volcano not associated with eruptions. Seismicity is given as the number of summit volcano-tectonic events per day excluding seismic swarms.
Variations de vitesse sismique au volcan du Piton de la Fournaise, non associées aux éruptions. La sismicité est donnée en tant que nombre d’évènements volcano-tectoniques sommitaux par jour, à l’exclusion des essaims sismiques.
4 Shallow changes of PdF Volcano detected by decrease of coherence of noise cross-correlation waveforms
As a complementary approach to the Moving Window Cross Spectrum analysis, the estimate of waveform decorrelation has been used to detect subtle changes within Mount Erebus Volcano (Grêt et al. (2005)), to image the time dependence of single scaterrers on the San-Andreas fault (Taira et al. (2008)), or to locate small changes in a medium (Larose et al. (2010)).
At PdF Volcano, we test the waveform changes of noise Cross-Correlation Functions (CCFs) induced by changes in the near-surface medium properties associated with dike intrusion and volcanic eruptions (Aoki and Ferrazzini (2009)). We focus on the time period between March 29 and April 02 2007. During this period, two eruptions occurred, the first on March 30–31, with duration of 10 hours and location on the south-eastern flank, and the second on April 2nd located about 8 km from the summit with duration of 30 days (Fig. 4a). We compute hourly noise cross-correlation functions for 2 receiver pairs in the frequency range of 0.5–3 Hz and then average the causal and acausal parts of the signal (Fig. 4b). We then compute, for each receiver pair, the correlation coefficients between all possible hourly cross-correlation functions (Fig. 4c). Either for the March 30–31 or April 2nd eruption, correlation coefficients computed between before and during these eruptive periods are low (Fig. 4b and c). This means that noise cross correlations for receiver pairs fer-NTR and NTR-tkr are significantly perturbed during the March 30–31 and April 2nd eruptions. A possible explanation is that the volcanic tremor signal associated with the eruptions dominates the ambient noise signal and thus strongly perturbs the noise CCFs. Cross-correlation functions computed between fer and NTR exhibit a strong decorrelation between before and after the March 30–31 eruption, which cannot be explained by a change in the ambient noise properties because NTR-tkr CCFs do not exhibit a similar decorrelation. This is confirmed by the observation that fer-NTR CCFs are stable after the March 30–31 and before the April 2nd eruptions (Fig. 4c). We thus conclude that the fer-NTR cross-correlation function decorrelation is caused by the change in the near-surface edifice properties associated with the intrusion of a dike and occurrence of the eruption. This preliminary study shows that monitoring noise cross-correlation waveform decorrelation is a promising tool for locating the areas of magma injection and the eruptive fissures.
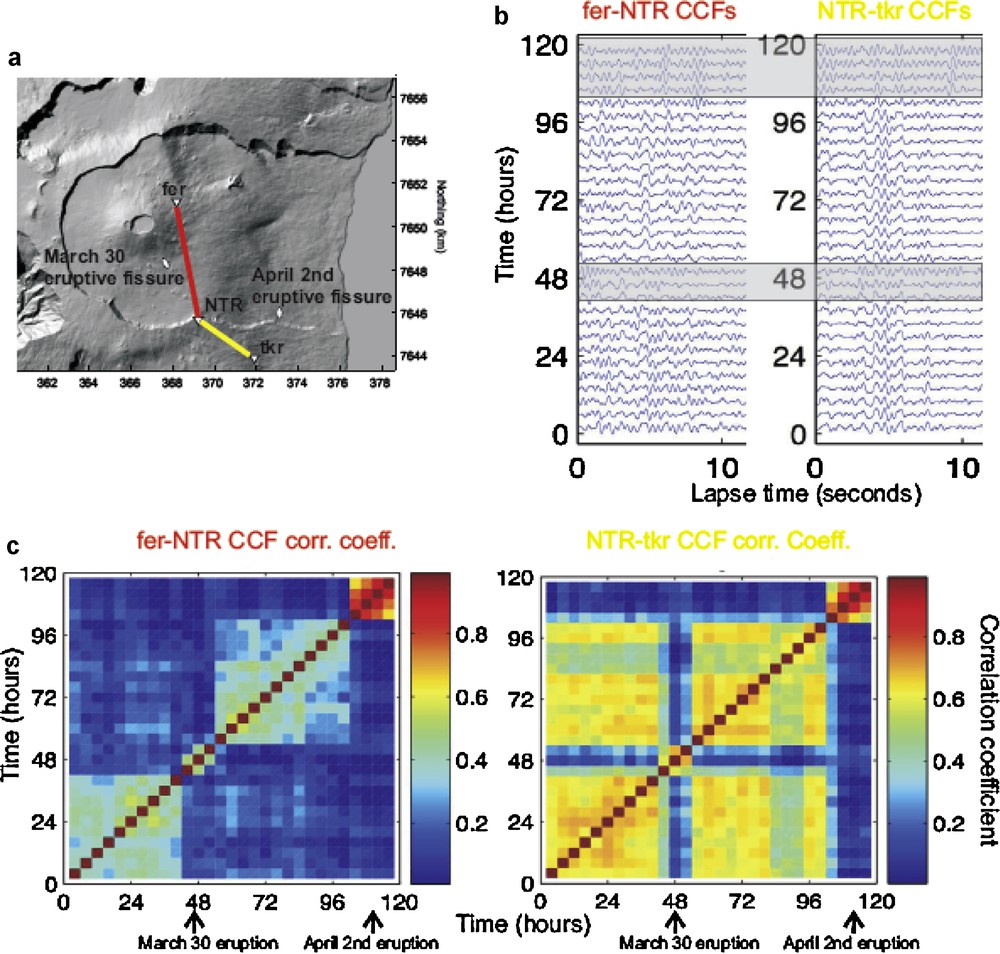
a) Position of March 30 and April 2nd eruptive fissures and of seismic stations used in this study. b) Hourly Cross-Correlation Functions (CCFs). Grey transparent rectangles illustrate periods of eruption c) Correlation coefficients computed between the hourly cross-correlation functions shown in b).
(a) Positions des fissures éruptives du 30 mars et du 2 avril et des stations sismiques utilisées dans cette étude. (b) Fonctions de corrélations horaires (CCFs). Les rectangles transparents gris illustrent les périodes d’éruption (c). Coefficients de corrélation, calculés entre les fonctions de corrélation horaires, présentées en (b).
5 Conclusions
Monitoring the active Earth and in particular volcanoes using seismic noise correlations is a new topic and promising area of investigation. Future methodological challenges concern:
- • the reduction of the time resolution. As described previously, filtering techniques using, for example, phase coherence may yield a great improvement;
- • the spatial localization of subtle changes detected. This problem will probably require a better understanding of the nature of waves that compose the coda of noise cross-correlations.
Finally it is important to emphasize that these methods are based on the massive processing of continuous seismic records using dense arrays and that future developments will require dense high-quality seismic networks with continuous recording.
Acknowledgments
All the data used in this study were collected at the Piton de la Fournaise Volcano Observatory. We are grateful to Elodie Rivemale for providing information about inter-eruptive seismicity, Aline Peltier for providing extensometer data, and Thomas Staudacher for fruitful discussions. We are also grateful to Bernard Chouet for a detailed review of the manuscript. This work has been supported by ANR (France) under contracts 05-CATT-010-01 (PRECORSIS), ANR-06-CEXC-005 (COHERSIS), ANR-08-RISK-011 (UNDERVOLC) and by a FP7 European Research Council advanced grant 227507 (WHISPER). This is IPGP contribution number 3105.